Chapter 5 Genetics
5.18 Case Study Conclusion: Cancer in the Family
Created by: CK-12/Adapted by Christine Miller
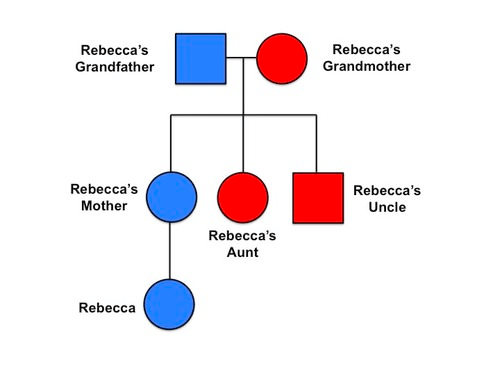
Case Study Conclusion: Cancer in the Family
Rebecca’s family tree, as illustrated in the pedigree above (Figure 5.18.1), shows a high incidence of cancer among close relatives. But are genes the cause of cancer in this family? Only genetic testing, which is the sequencing of specific genes in an individual, can reveal whether a cancer-causing gene is being inherited in this family.
Fortunately for Rebecca, the results of her genetic testing show that she does not have the mutations in the BRCA1 and BRCA2 genes that most commonly increase a person’s risk of getting cancer. This doesn’t mean, however, that she doesn’t have other mutations in these genes that could increase her risk of getting cancer. There are many other mutations in BRCA genes whose effect on cancer risk is still not known — and there may be many more yet to be discovered. Figure 5.18.2 from the National Cancer Institute illustrates many of the different types of known mutations in the BRCA1 gene. It is important to continue to study the variations in genes such as BRCA in different people to better assess their possible contribution to the development of disease. As you now know from this chapter, many mutations are harmless, while others can cause significant health effects, depending on the specific mutation and the gene involved.
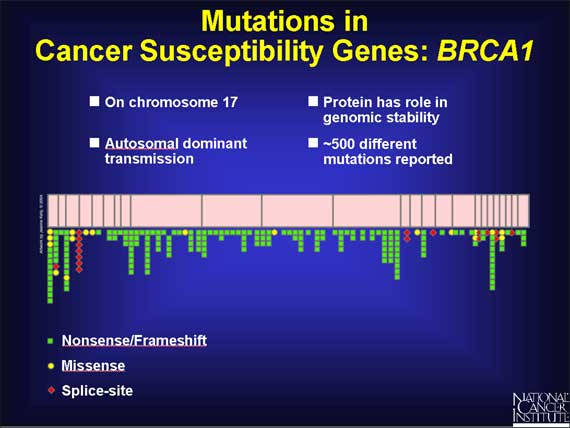
Mutations in BRCA genes are particularly likely to cause cancer because these genes encode for tumor-suppressor proteins that normally repair damaged DNA and control cell division. If these genes are mutated in a way that causes the proteins to not function properly, other mutations can accumulate and cell division can run out of control, which can cause cancer.
BRCA1 and BRCA2 are on chromosomes 17 and 13, respectively, which are autosomes. As Rebecca’s genetic counselor mentioned, mutations in these genes have a dominant inheritance pattern. Now that you know the pattern of inheritance of autosomal dominant genes, if Rebecca’s grandmother did have one copy of a mutated BRCA gene, what are the chances that Rebecca’s mother also has this mutation? Because it is dominant, only one copy of the gene is needed to increase the risk of cancer, and because it is on autosomes instead of sex chromosomes, the sex of the parent or offspring does not matter in the inheritance pattern. In this situation, Rebecca’s grandmother’s eggs would have had a 50 per cent chance of having a BRCA gene mutation (Mendel’s law of segregation). Therefore, Rebecca’s mother would have had a 50 per cent chance of inheriting this gene. Even though Rebecca does not have the most common BRCA mutations that increase the risk of cancer, it does not mean that her mother does not, because there would also only be a 50 per cent chance that she would pass it on to Rebecca. Rebecca’s mother, therefore, should consider getting tested for mutations in the BRCA genes, as well. Ideally, the individuals with cancer in a family should be tested first when a genetic cause is suspected, so that if there is a specific mutation being inherited, it can be identified, and the other family members can be tested for that same mutation.
Mutations in both BRCA1 and BRCA2 are often found in Ashkenazi Jewish families. However, these genes are not linked in the chromosomal sense, because they are on different chromosomes and are therefore inherited independently, in accordance with Mendel’s law of independent assortment. Why would certain gene mutations be prevalent in particular ethnic groups? If people within an ethnic group tend to produce offspring with each other, their genes will remain prevalent within the group. These may be genes for harmless variations such as skin, hair, or eye colour, or harmful variations such as the mutations in the BRCA genes. Other genetically based diseases and disorders are sometimes more commonly found in particular ethnic groups, such as cystic fibrosis in people of European descent, and sickle cell anemia in people of African descent. You will learn more about the prevalence of certain genes and traits in particular ethnic groups and populations in the chapter on Human Variation.
As you learned in this chapter, genetics is not the sole determinant of phenotype. The environment can also influence many traits, including adult height and skin colour. The environment plays a major role in the development of cancer, too. Ninety to 95 per cent of all cancers do not have an identified genetic cause, and are often caused by mutagens in the environment, such as UV radiation from the sun or toxic chemicals in cigarette smoke. But for families like Rebecca’s, knowing their family health history and genetic makeup may help them better prevent or treat diseases that are caused by their genetic inheritance. If a person knows they have a gene that can increase their risk of cancer, they can make lifestyle changes and have early and more frequent cancer screenings. They may even choose to have preventative surgeries that can help reduce their risk of getting cancer and increase their odds of long-term survival if cancer does occur. The next time you go to the doctor and they ask whether any members of your family have had cancer, you will have a deeper understanding why this information is so important to your health.
Chapter 5 Summary
In this chapter you learned about genetics — the science of heredity. Specifically you learned that:
- Chromosomes are structures made of DNA and proteins that are encoded with genetic instructions for making RNA and proteins. The instructions are organized into units called genes, which are segments of DNA that code for particular pieces of RNA. The RNA molecules can then act as a blueprint for proteins, or directly help regulate various cellular processes.
- Humans normally have 23 pairs of chromosomes. Of these, 22 pairs are autosomes, which contain genes for characteristics unrelated to sex. The other pair consists of sex chromosomes (XX in females, XY in males). Only the Y chromosome contains genes that determine sex.
- Humans have an estimated 20 thousand to 22 thousand genes. The majority of human genes have two or more possible versions, called alleles.
- Genes that are located on the same chromosome are called linked genes. Linkage explains why certain characteristics are frequently inherited together.
- Determining that DNA is the genetic material was an important milestone in biology.
-
- In the 1920s, Griffith showed that something in virulent bacteria could be transferred to nonvirulent bacteria, making them virulent, as well.
- In the 1940s, Avery and colleagues showed that the “something” Griffith found was DNA and not protein. This result was confirmed by Hershey and Chase, who demonstrated that viruses insert DNA into bacterial cells.
- In the 1950s, Chargaff showed that in DNA, the concentration of adenine is always the same as the concentration of thymine, and the concentration of guanine is always the same as the concentration of cytosine. These observations came to be known as Chargaff's rules.
- In the 1950s, James Watson and Francis Crick, building on the prior X-ray research of Rosalind Franklin and others, discovered the double helix structure of the DNA molecule.
- Knowledge of DNA’s structure helped scientists understand how DNA replicates, which must occur before cell division. DNA replication is semi-conservative because each daughter molecule contains one strand from the parent molecule and one new strand that is complementary to it.
- The central dogma of molecular biology can be summed up as: DNA → RNA → Protein. This means that the genetic instructions encoded in DNA are transcribed to RNA. From RNA, they are translated into a protein.
- RNA is a nucleic acid. Unlike DNA, RNA consists of just one polynucleotide chain instead of two, contains the base uracil instead of thymine, and contains the sugar ribose instead of deoxyribose.
- The main function of RNA is to help make proteins. There are three main types of RNA: messenger RNA (mRNA), ribosomal RNA (rRNA), and transfer RNA (tRNA).
- According to the RNA world hypothesis, RNA was the first type of biochemical molecule to evolve, predating both DNA and proteins.
- The genetic code was cracked in the 1960s by Marshall Nirenberg. It consists of the sequence of nitrogen bases in a polynucleotide chain of DNA or RNA. The four bases make up the “letters” of the code. The letters are combined in groups of three to form code “words,” or codons, each of which encodes for one amino acid or a start or stop signal.
-
- AUG is the start codon, and it establishes the reading frame of the code. After the start codon, the next three bases are read as the second codon, and so on until a stop codon is reached.
- The genetic code is universal, unambiguous, and redundant.
- Protein synthesis is the process in which cells make proteins. It occurs in two stages: transcription and translation.
-
- Transcription is the transfer of genetic instructions in DNA to mRNA in the nucleus. It includes the steps of initiation, elongation, and termination. After the mRNA is processed, it carries the instructions to a ribosome in the cytoplasm.
- Translation occurs at the ribosome, which consists of rRNA and proteins. In translation, the instructions in mRNA are read, and tRNA brings the correct sequence of amino acids to the ribosome. Then rRNA helps bonds form between the amino acids, producing a polypeptide chain.
- After a polypeptide chain is synthesized, it may undergo additional processing to form the finished protein.
- Mutations are random changes in the sequence of bases in DNA or RNA. They are the ultimate source of all new genetic variation in any species.
-
- Mutations may happen spontaneously during DNA replication or transcription. Other mutations are caused by environmental factors called mutagens.
- Germline mutations occur in gametes and may be passed on to offspring. Somatic mutations occur in cells other than gametes and cannot be passed on to offspring.
- Chromosomal alterations are mutations that change chromosome structure and usually affect the organism in multiple ways. Charcot-Marie-Tooth disease type 1 is an example of a chromosomal alteration.
- Point mutations are changes in a single nucleotide. The effects of point mutations depend on how they change the genetic code, and may range from no effects to very serious effects.
- Frameshift mutations change the reading frame of the genetic code and are likely to have a drastic effect on the encoded protein.
- Many mutations are neutral and have no effects on the organism in which they occur. Some mutations are beneficial and improve fitness, while others are harmful and decrease fitness.
- Using a gene to make a protein is called gene expression. Gene expression is regulated to ensure that the correct proteins are made when and where they are needed. Regulation may occur at any stage of protein synthesis or processing.
-
- The regulation of transcription is controlled by regulatory proteins that bind to regions of DNA called regulatory elements, which are usually located near promoters. Most regulatory proteins are either activators that promote transcription or repressors that impede transcription.
- A regulatory element common to almost all eukaryotic genes is the TATA box. A number of regulatory proteins must bind to the TATA box in the promoter before transcription can proceed.
- The regulation of gene expression is extremely important during the early development of an organism. Homeobox genes, which encode for chains of amino acids called homeodomains, are important genes that regulate development.
- Some types of cancer occur because of mutations in genes that control the cell cycle. Cancer-causing mutations most often occur in two types of regulatory genes, called tumor-suppressor genes and proto-oncogenes.
- Mendel experimented with the inheritance of traits in pea plants, which have two different forms of several visible characteristics. Mendel crossed pea plants with different forms of traits.
-
- In Mendel’s first set of experiments, he crossed plants that only differed in one characteristic. The results led to Mendel’s first law of inheritance, called the law of segregation. This law states that there are two factors controlling a given characteristic, one of which dominates the other, and these factors separate and go to different gametes when a parent reproduces.
- In Mendel’s second set of experiments, he experimented with two characteristics at a time. The results led to Mendel’s second law of inheritance, called the law of independent assortment. This law states that the factors controlling different characteristics are inherited independently of each other.
- Mendel’s laws of inheritance, now expressed in terms of genes, form the basis of genetics, the science of heredity. Mendel is often called the father of genetics.
- The position of a gene on a chromosome is its locus. A given gene may have different versions called alleles. Paired chromosomes of the same type are called homologous chromosomes and they have the same genes at the same loci.
- The alleles an individual inherits for a given gene make up the individual’s genotype. An organism with two of the same alleles is called a homozygote, and an individual with two different alleles is called a heterozygote.
- The expression of an organism’s genotype is referred to as its phenotype. A dominant allele is always expressed in the phenotype, even when just one dominant allele has been inherited. A recessive allele is expressed in the phenotype only when two recessive alleles have been inherited.
- In sexual reproduction, two parents produce gametes that unite in the process of fertilization to form a single-celled zygote. Gametes are haploid cells with only one of each pair of homologous chromosomes, and the zygote is a diploid cell with two of each pair of chromosomes.
- Meiosis is the type of cell division that produces four haploid daughter cells that may become gametes. Meiosis occurs in two stages, called meiosis I and meiosis II, each of which occurs in four phases (prophase, metaphase, anaphase, and telophase).
- Meiosis is followed by gametogenesis, the process in which the haploid daughter cells change into mature gametes. Males produce gametes called sperm through spermatogenesis, and females produce gametes called eggs through oogenesis.
- Sexual reproduction produces offspring that are genetically unique. Crossing-over, independent alignment, and the random union of gametes result in a high degree of genetic variation.
- Mendelian inheritance refers to the inheritance of traits controlled by a single gene with two alleles, one of which may be completely dominant to the other. The pattern of inheritance of Mendelian traits depends on whether the traits are controlled by genes on autosomes or by genes on sex chromosomes.
-
- Examples of human autosomal Mendelian traits include albinism and Huntington’s disease. Examples of human X-linked traits include red-green colour blindness and hemophilia.
- Two tools for studying inheritance are pedigrees and Punnett squares. A pedigree is a chart that shows how a trait is passed from generation to generation. A Punnett square is a chart that shows the expected ratios of possible genotypes in the offspring of two parents.
- Non-Mendelian inheritance refers to the inheritance of traits that have a more complex genetic basis than one gene with two alleles and complete dominance.
-
- Multiple allele traits are controlled by a single gene with more than two alleles. An example of a human multiple allele trait is ABO blood type.
- Codominance occurs when two alleles for a gene are expressed equally in the phenotype of heterozygotes. A human example of codominance occurs in the AB blood type, in which the A and B alleles are codominant.
- Incomplete dominance is the case in which the dominant allele for a gene is not completely dominant to a recessive allele, so an intermediate phenotype occurs in heterozygotes who inherit both alleles. A human example of incomplete dominance is Tay Sachs disease, in which heterozygotes produce half as much functional enzyme as normal homozygotes.
- Polygenic traits are controlled by more than one gene, each of which has a minor additive effect on the phenotype. This results in a continuum of phenotypes. Examples of human polygenic traits include skin colour and adult height. Many of these types of traits, as well as others, are affected by the environment, as well as by genes.
- Pleiotropy refers to the situation in which a gene affects more than one phenotypic trait. A human example of pleiotropy occurs with sickle cell anemia, which has multiple effects on the body.
- Epistasis is when one gene affects the expression of other genes. An example of epistasis is albinism, in which the albinism mutation negates the expression of skin colour genes.
- Genetic disorders are diseases, syndromes, or other abnormal conditions that are caused by mutations in one or more genes or by chromosomal alterations.
-
- Examples of genetic disorders caused by single-gene mutations include Marfan syndrome (autosomal dominant), sickle cell anemia (autosomal recessive), vitamin D-resistant rickets (X-linked dominant), and hemophilia A (X-linked recessive). Very few genetic disorders are caused by dominant mutations because these alleles are less likely to be passed on to successive generations.
- Nondisjunction is the failure of replicated chromosomes to separate properly during meiosis. This may result in genetic disorders caused by abnormal numbers of chromosomes. An example is Down syndrome, in which the individual inherits an extra copy of chromosome 21. Most chromosomal disorders involve the X chromosome. An example is Klinefelter’s syndrome (XXY, XXXY).
- Prenatal genetic testing (by amniocentesis, for example) can detect chromosomal alterations in utero. The symptoms of some genetic disorders can be treated or prevented. For example, symptoms of phenylketonuria (PKU) can be prevented by following a low-phenylalanine diet throughout life.
- Cures for genetic disorders are still in the early stages of development. One potential cure is gene therapy, in which normal genes are introduced into cells by a vector such as a virus to compensate for mutated genes.
- Genetic engineering is the use of technology to change the genetic makeup of living things for human purposes.
-
- Genetic engineering methods include gene cloning and the polymerase chain reaction. Gene cloning is the process of isolating and making copies of a DNA segment, such as a gene. The polymerase chain reaction makes many copies of a gene or other DNA segment.
- Genetic engineering can be used to transform bacteria so they are able to make human proteins, such as insulin. It can also be used to create transgenic crops, such as crops that yield more food or resist insect pests.
- Genetic engineering has raised a number of ethical, legal, and social issues including health, environmental, and privacy concerns.
- The human genome refers to all of the DNA of the human species. It consists of more than 3.3 billion base pairs divided into 20,500 genes on 23 pairs of chromosomes.
- The Human Genome Project (HGP) was a multi-billion dollar international research project that began in 1990. By 2003, it had sequenced and mapped the location of all of the DNA base pairs in the human genome. It published the results as a human reference genome that is available to anyone on the Internet.
- Sequencing of the human genome is helping researchers better understand cancer and genetic diseases. It is also helping them tailor medications to individual patients, which is the focus of the new field of pharmacogenomics. In addition, it is helping researchers better understand human evolution.
Chapter 5 Review
-
- What are the differences between a sequence of DNA and the sequence of mature mRNA that it produces?
- Scientists sometimes sequence DNA that they “reverse transcribe” from the mRNA in an organism’s cells, which is called complementary DNA (cDNA). Why do you think this technique might be particularly useful for understanding an organism’s proteins versus sequencing the whole genome (i.e. nuclear DNA) of the organism?
- A person has a hypothetical A a genotype. Answer the following questions about this genotype:
- What do A and a represent?
- If the person expresses only the phenotype associated with A, is this an example of complete dominance, codominance, or incomplete dominance? Explain your answer. Also, describe what the observed phenotypes would be if it were either of the two incorrect answers.
- Explain how a mutation that occurs in a parent can result in a genetic disorder in their child. Be sure to include which type of cell or cells in the parent must be affected in order for this to happen.
- What is the term for an allele that is not expressed in a heterozygote?
- What might happen if codons encoded for more than one amino acid?
- Explain why a human gene can be inserted into bacteria and can still produce the correct human protein, despite being in a very different organism.
- What is gene therapy? Why is gene therapy considered a type of biotechnology?
Attributions
Figure 5.18.1
Rebeccas Pedigree Cancer by CK-12 Foundation is used under a CC BY 3.0 (https://creativecommons.org/licenses/by/3.0/) license.
©CK-12 Foundation Licensed under
• Terms of Use • Attribution
Figure 5.18.2
Mutations_on_BRCA1 by National Cancer Institute (NCI) on Wikimedia Commons is in the public domain (https://en.wikipedia.org/wiki/Public_domain).
Reference
Brainard, J/ CK-12 Foundation. (2016). Figure 1 Pedigree for Rebecca’s family, as described in the beginning of this chapter, [digital image]. In CK-12 College Human Biology (Section 5.17) [online Flexbook]. CK12.org. https://www.ck12.org/book/ck-12-college-human-biology/section/5.17/
A diagram that shows the occurrence and appearance of phenotypes of a particular gene or organism and its ancestors from one generation to the next, most commonly humans, show dogs, and race horses.
A group of diseases involving abnormal cell growth with the potential to invade or spread to other parts of the body.
A sequence of nucleotides in DNA or RNA that codes for a molecule that has a function.
An alteration in the nucleotide sequence of the genome of an organism.
Any chromosome that is not a sex chromosome.
A threadlike structure of nucleic acids and protein found in the nucleus of most living cells, carrying genetic information in the form of genes.
Deoxyribonucleic acid - the molecule carrying genetic instructions for the development, functioning, growth and reproduction of all known organisms and many viruses.
A class of biological molecule consisting of linked monomers of amino acids and which are the most versatile macromolecules in living systems and serve crucial functions in essentially all biological processes.
A nucleic acid of which many different kinds are now known, including messenger RNA, transfer RNA and ribosomal RNA.
A pair of chromosomes that determines biological sex.
A variant form of a given gene, meaning it is one of two or more versions of a known mutation at the same place on a chromosome. It can also refer to different sequence variations for a several-hundred base-pair or more region of the genome that codes for a protein.
Genes that are likely to be inherited together because they are physically close to one another on the same chromosome.
The rules developed by Erwin Chargaff stating that in a double stranded strand of DNA, the amount of adenine is always equal to the amount of thymine, and the amount of guanine is always equal to the amount of cytosine.
The process by which a parent cell divides into two or more daughter cells. Cell division usually occurs as part of a larger cell cycle.
The process by which DNA is copied.
An explanation of the flow of genetic information within a biological system. It is often stated as "DNA makes RNA and RNA makes protein."
A complex organic substance present in living cells, especially DNA or RNA, whose molecules consist of many nucleotides linked in a long chain.
A large family of RNA molecules that convey genetic information from DNA to the ribosome, where they specify the amino acid sequence of the protein products of gene expression.
A type of RNA that acts as the primary building block for ribosomes and the assembly line on which protein synthesis occurs in those ribosomes.
A small RNA molecule that participates in protein synthesis. Each tRNA molecule has two important areas: an anticodon and a region for attaching a specific amino acid.
A sequence of 3 DNA or RNA nucleotides that corresponds with a specific amino acid or stop signal during protein synthesis.
Amino acids are organic compounds that combine to form proteins.
The specific location in DNA where a set of codons will code for a certain protein. The reading frame begins with the start codon (AUG).
The process by which DNA is copied (transcribed) to mRNA in order transfer the information needed for protein synthesis.
The process in which mRNA along with transfer RNA (tRNA) and ribosomes work together to produce polypeptides.
A physical or chemical agent that changes the genetic material, usually DNA, of an organism.
Mutation in cells destined to become egg or sperm cells. These mutations can be passed to offspring.
Mutations acquired by a cell that can be passed to future cells arising from the mutated cell in the course of cell division.
A mutation that changes the structure of an individual chromosome, leading to imbalance involving only a part of a chromosome, such as duplication, deletion, or translocation.
A mutation that only affects a single nucleotide of nucleic acid.
A genetic mutation caused by a deletion or insertion in a DNA sequence that shifts the way the sequence is read.
The process by which information from a gene is used in the synthesis of a functional protein.
A sequence of DNA to which proteins bind that initiate transcription of a single mRNA from the DNA downstream of it.
Regulatory proteins that prevent transcription by impeding the progress of RNA polymerase along the DNA strand, so the DNA cannot be transcribed to mRNA.
A DNA sequence that indicates where a genetic sequence can be read and decoded. It is a type of promoter sequence, which specifies to other molecules where transcription begins.
A large group of similar genes that direct the formation of many body structures during early embryonic development.
The part of a protein that attaches (binds) to specific regulatory regions of the target genes.
A cycle of growth and division that cells go through. It includes interphase (G1, S, and G2) and the mitotic phase.
Allele pairs separate or segregate during gamete formation and randomly unite at fertilization.
The alleles of two (or more) different genes get sorted into gametes independently of one another.
A specific, fixed position on a chromosome where a particular gene or genetic marker is located.
Two pieces of DNA within a diploid organism which carry the same types genes, one from each parental source.
The part of the genetic makeup of a cell, and therefore of any individual, which determines one of its characteristics (phenotype).
An organism with identical pairs of genes (or alleles) for a specific trait. If both of the two gametes (sex cells) that fuse during fertilization carry the same form of the gene for a specific trait, the organism is said to be homozygous for that trait.
An individual who has two different forms of a particular gene, one inherited from each parent.
The set of observable characteristics of an individual resulting from the interaction of its genotype with the environment.
Refers to the relationship between two versions of a gene. Individuals receive two versions of each gene, known as alleles, from each parent. If the alleles of a gene are different, one allele will be expressed; it is the dominant gene. The effect of the other allele, called recessive, is masked.
A gene that can be masked by a dominant gene. In order to have a trait that is expressed by a recessive gene, such as blue eyes, you must get the gene for blue eyes from both of your parents.
A type of reproduction that involves a complex life cycle in which a gamete with a single set of chromosomes combines with another to produce an organism composed of cells with two sets of chromosomes.
A mature haploid male or female germ cell which is able to unite with another of the opposite sex in sexual reproduction to form a zygote.
The fusion of haploid gametes, egg and sperm, to form the diploid zygote.
The union of the sperm cell and the egg cell. Also known as a fertilized ovum, the zygote begins as a single cell but divides rapidly in the days following fertilization. After this two-week period of cell division, the zygote eventually becomes an embryo.
The term used when a cell has half the usual number of chromosomes.
Describes a cell that contain two copies of each chromosome.
A special type of cell division in sexually-reproducing organisms used to produce the gametes, such as sperm or egg cells. It involves two rounds of division that ultimately result in four cells with only one copy of each chromosome.
The process whereby a haploid cell (n) is formed from a diploid cell (2n) through meiosis and cell differentiation. Gametogenesis in the male is known as spermatogenesis and produces spermatozoa. Gametogenesis in the female is known as oogenesis and result in the formation of ova.
The production or development of mature spermatozoa.
The production or development of an ovum.
The exchange of genetic material between two homologous chromosomes non-sister chromatids that results in recombinant chromosomes during sexual reproduction.
The way in which different genes independently separate from one another when reproductive cells develop. During meiosis, the pairs of homologous chromosome are divided in half to form haploid cells, and this separation, or assortment, of homologous chromosomes is random.
A type of biological inheritance that follows the principles originally proposed by Gregor Mendel in 1865 and 1866, re-discovered in 1900 and popularised by William Bateson.
A square diagram that is used to predict the genotypes of a particular cross or breeding experiment. Named after Reginald C. Punnett, who devised the approach, this diagram is used by biologists to determine the probability of an offspring having a particular genotype.
Traits controlled by a single gene with more than two alleles.
Means that neither allele can mask the expression of the other allele.
A heredity pattern in which phenotype of the heterozygous genotype is distinct from and often intermediate to the phenotypes of the homozygous genotypes.
One whose phenotype is influenced by more than one gene. Traits that display a continuous distribution, such as height or skin color, are polygenic.
Describes the genetic effect of a single gene on multiple phenotypic traits. The underlying mechanism is genes that code for a product that is either used by various cells or has a cascade-like signaling function that affects various targets.
A phenomenon in genetics in which the effect of a gene mutation is dependent on the presence or absence of mutations in one or more other genes, respectively termed modifier genes. In other words, the effect of the mutation is dependent on the genetic background in which it appears.
Diseases, syndromes, or other abnormal conditions caused by mutations in one or more genes, or by chromosomal alterations.
The failure of homologous chromosomes or sister chromatids to separate properly during cell division.
A medical procedure used primarily in prenatal diagnosis of chromosomal abnormalities and fetal infections as well as for sex determination. In this procedure, a small amount of amniotic fluid, which contains fetal tissues, is sampled from the amniotic sac surrounding a developing fetus.
An experimental technique that uses genes to treat or prevent disease.
The use of technology to change the genetic makeup of living things for human purposes.
The process of isolating and making copies of a gene.
A method used widely in molecular biology to make millions to billions of copies of a specific DNA sample rapidly, allowing scientists to take a very small sample of DNA and amplify it to a large enough amount to study in detail.
Plants used in agriculture, the DNA of which has been modified using genetic engineering methods. In most cases, the aim is to introduce a new trait to the plant which does not occur naturally in the species.
Refers to all the DNA of the human species.
An international scientific research project with the goal of determining the base pairs that make up human DNA, and of identifying and mapping all of the genes of the human genome from both a physical and a functional standpoint.