Alan Jenks
Heart rate is one of the most accessible physiological measures in sports medicine and exercise physiology. Monitoring heart rate under different physiological conditions can provide a wealth of knowledge about the health of an individual. It is controlled by a sophisticated network of integrated mechanisms, which includes hormonal regulation (such as epinephrine), baroreceptors, chemoreceptors, local tissue metabolism and respiration. The most important and primary control mechanism, however, is the autonomic nervous system (ANS) and its two branches: the parasympathetic and sympathetic nervous systems.
The normal heartbeat starts with an action potential initiated at the sino-atrial (SA) node, which first spreads throughout both atria via two pathways – the interatrial and internodal pathways, the latter of which leads the action potential to the AV node. The AV node is the only location where an action potential can spread from the atria to the ventricles. From the AV node, the action potential spreads rapidly throughout the ventricles via a specialized ventricular conduction system consisting of the bundle of His and Purkinje fibers. A record of the overall spread of electrical activity throughout the heart can be interpreted through an electrocardiogram signal (ECG), where different portions of the ECG signal have been associated with specific aspects of the cardiac cycle.
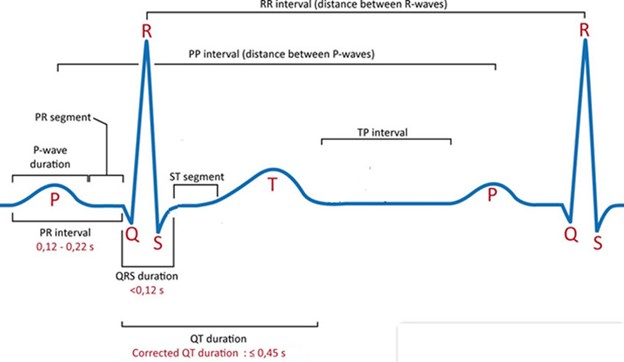
Heart rate is measured as the frequency that the heart beats per minute. Beats are traditionally measured from R wave to R wave (RR interval), where the R wave is part of the QRS complex on the ECG signal, denoting ventricular depolarization. Within the heart, the SA node (the pacemaker), will naturally discharge (initiate action potentials) around 120 beats/min. External modulation of the SA node is mainly controlled by the ANS. The parasympathetic nervous system acts directly on the SA node, the atrial myocardium and the AV node via release of acetylcholine by the vagus nerve (Aubert et al., 2003). This slows the activity at the pacemaker and resultant cardiac impulses sent through to the ventricles, slowing heart rate. The sympathetic nervous system has the opposite effect on heart rate. Sympathetic nerve endings are positioned all over the myocardium, which release norepinephrine and stimulate the SA node. This increases the rate of SA node discharge, accelerating atrio-venticular conduction, increasing heart rate (Aubert et al., 2003).
At rest, vagal tone normally prevails, setting a resting heart rate on average between 60- 80 beats/min. Resting heart rate can be altered by a number of variables, including age, sex, mental stress, smoking, alcohol, and physical activity (Valentini et al., 2009). For example, regular physical activity can decrease resting heart rate, which it is thought to be due in part by greater parasympathetic control and reduced sympathetic modulation. Unfortunately, since the factors that affect resting heart rate are multi-factorial and complex, using resting heart rate a precise marker of ANS activity is not suggested. It can, however, be used as a general gauge of neural cardiovascular control.
The variation in time between heartbeats (i.e. the length of RR intervals) has been a topic of much exploration in the past few decades. Variation in beat-to-beat length has been shown to represent the fine-tuning of both arms of the ANS (HRV, 1996). These autonomic signals are modulated by central and peripheral mechanisms, leading to different oscillations in interval lengths between beats and between series of beats. These varying oscillations have been associated with different neural effects.
Higher frequency (faster occurring) oscillations have been shown to be under the control of the parasympathetic nervous system. These oscillations are intrinsically tied to respiration, through a phenomenon termed respiratory sinus arrhythmia (RSA). RSA is a rhythmical fluctuation in heartbeat intervals characterized by a shortening of RR intervals on inspiration and lengthening on expiration. This is tied to vagal nerve activity, as lung expansion inhibits the vagus nerve (shortening RR interval time) and expiration leads to preferential firing of the vagus nerve via stimulation of arterial chemoreceptors and baroreceptors (Yasuma et al., 2004). These oscillations in RR intervals occur between 9-40 times per minute (which is equal to a frequency of 0.15 – 0.4 Hz (cycles/second), known as a high frequency band).
Contrastingly, longer oscillations also occur across many RR intervals, which have been associated primarily with sympathetic activity, and to a less extent, the parasympathetic nervous system. These occur anywhere from 2.4 – 9 cycles per minute (0.04 – 0.15 Hz, known as a low frequency band). Comparing the ratio of these different oscillatory bands (low frequency, high frequency, and their ratio (LF/HF)) can provide information about autonomic control of the heart. These high frequency (HF) and low frequency (LF) oscillations can be extracted from a RR – interval data set by breaking down each component into its sinusoidal wave-form through a fast-fourier transformation.
This type of analysis, known as frequency domain analysis of heart rate variability, is used in a multitude of exercise science areas, from measures of training and performance status to clinical measures such as assessment of risk after a heart attack or assessment of diabetic neuropathy (HRV, 2009).
Interestingly, even with the complexity of the physiology behind understanding heart rate variability, a number of wearable tech companies have included this measurement in their repertoire of metrics. Indeed the lay person may loosely interpret a greater heart rate variability as a more relaxed state, and a lower heart rate variability as a more stressed state. Perhaps you have even done this yourself when looking over the numbers on your apple watch!
Media Attributions
- Picture1
- Picture2