4.12 Cell Cycle and Cell Division
Created by: CK-12/Adapted by Christine Miller
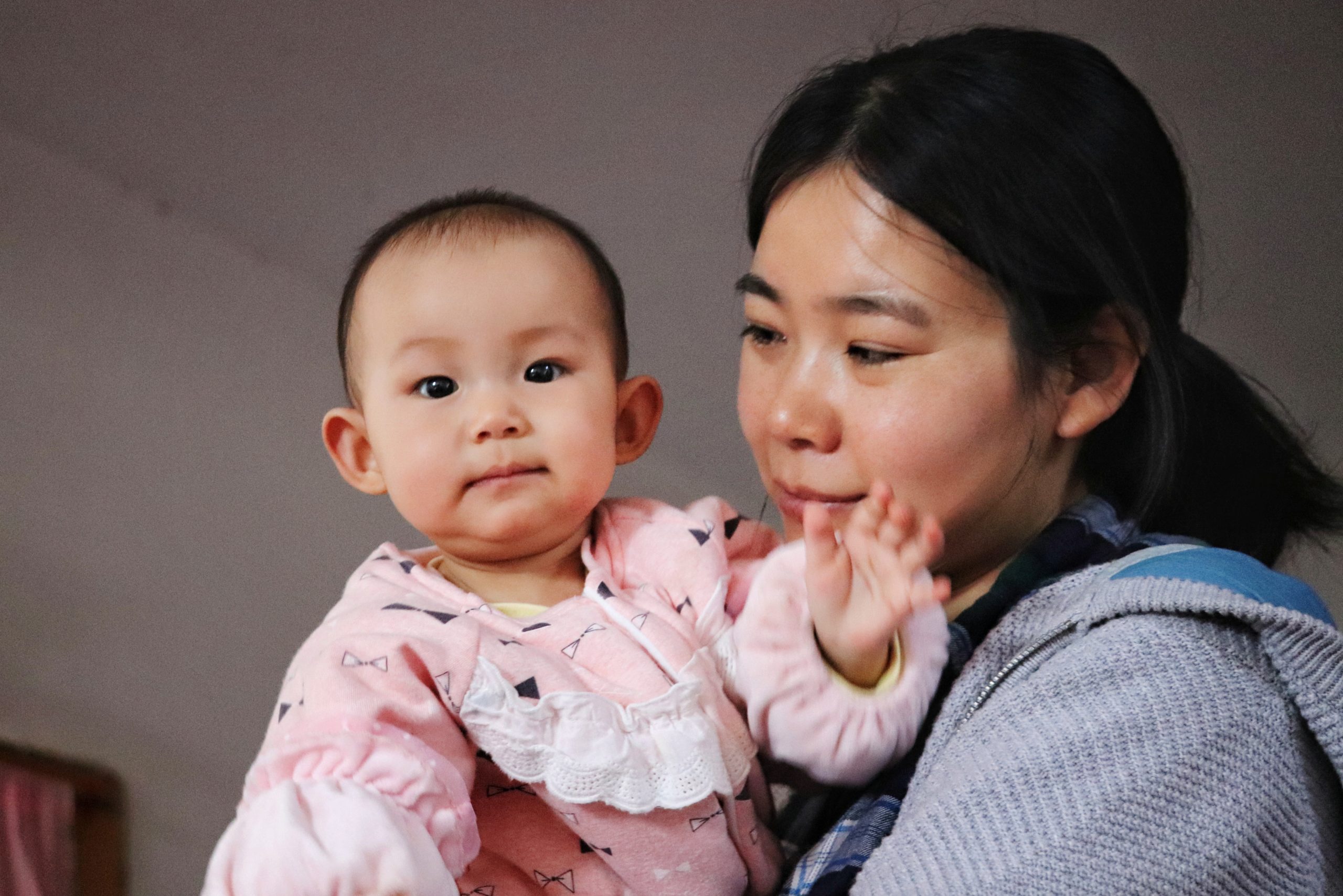
So Many Cells!
This baby girl (Figure 4.12.1) has a lot of growing to do before she’s as big as her mom. Most of her growth will be the result of cell division. By the time she is an adult, her body will consist of trillions of cells. Cell division is just one of the stages that all cells go through during their life. This includes cells that are harmful, such as cancer cells. Cancer cells divide more often than normal cells, causing them to grow out of control. In fact, this is how cancer cells cause illness. In this concept, you will read about how cells divide, what other stages cells go through, and what causes cancer cells to divide out of control and harm the body.
The Cell Cycle
Cell division is just one of several stages that a cell goes through during its lifetime. The cell cycle is a repeating series of events that includes growth, DNA synthesis, and cell division. The cell cycle in prokaryotes is quite simple: the cell grows, its DNA replicates, and the cell divides. In eukaryotes, the cell cycle is more complicated.
Eukaryotic Cell Cycle
The diagram in Figure 4.12.2 represents the cell cycle of a eukaryotic cell. As you can see, the eukaryotic cell cycle has several phases. The mitotic phase (M) actually includes both mitosis and cytokinesis. This is when the nucleus and then the cytoplasm divide. The other three phases (G1, S, and G2) are generally grouped together as interphase. During interphase, the cell grows, performs routine life processes, and prepares to divide. These phases are discussed below.
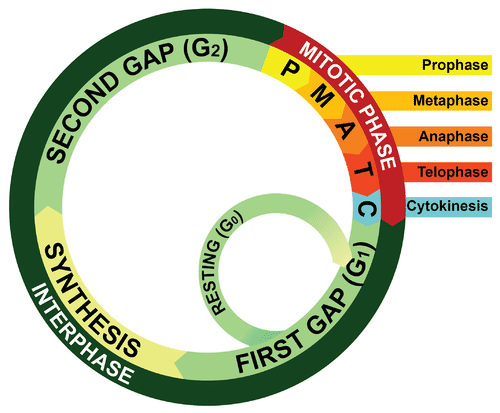
Interphase
The interphase of the eukaryotic cell cycle can be subdivided into the three phases described below, which are represented in Figure 4.12.2.
- Growth Phase 1 (G1): During this phase, the cell grows rapidly, while performing routine metabolic processes. It also makes proteins needed for DNA replication and copies some of its organelles in preparation for cell division. A cell typically spends most of its life in this phase. This phase is also known as gap phase 1.
- Synthesis Phase (S): During this phase, the cell’s DNA is copied in the process of DNA replication, in order to prepare for the upcoming mitotic phase.
- Growth Phase 2 (G2): During this phase, the cell makes final preparations to divide. For example, it makes additional proteins and organelles. This phase is also known as gap phase 2.
Control of the Cell Cycle
If the cell cycle occurred without regulation, cells might go from one phase to the next before they were ready. What controls the cell cycle? How does the cell know when to grow, synthesize DNA, and divide? The cell cycle is controlled mainly by regulatory proteins. These proteins control the cycle by signaling the cell to either start or delay the next phase of the cycle. They ensure that the cell completes the previous phase before moving on. Regulatory proteins control the cell cycle at key checkpoints, which are shown in Figure 4.12.3. There are a number of main checkpoints.
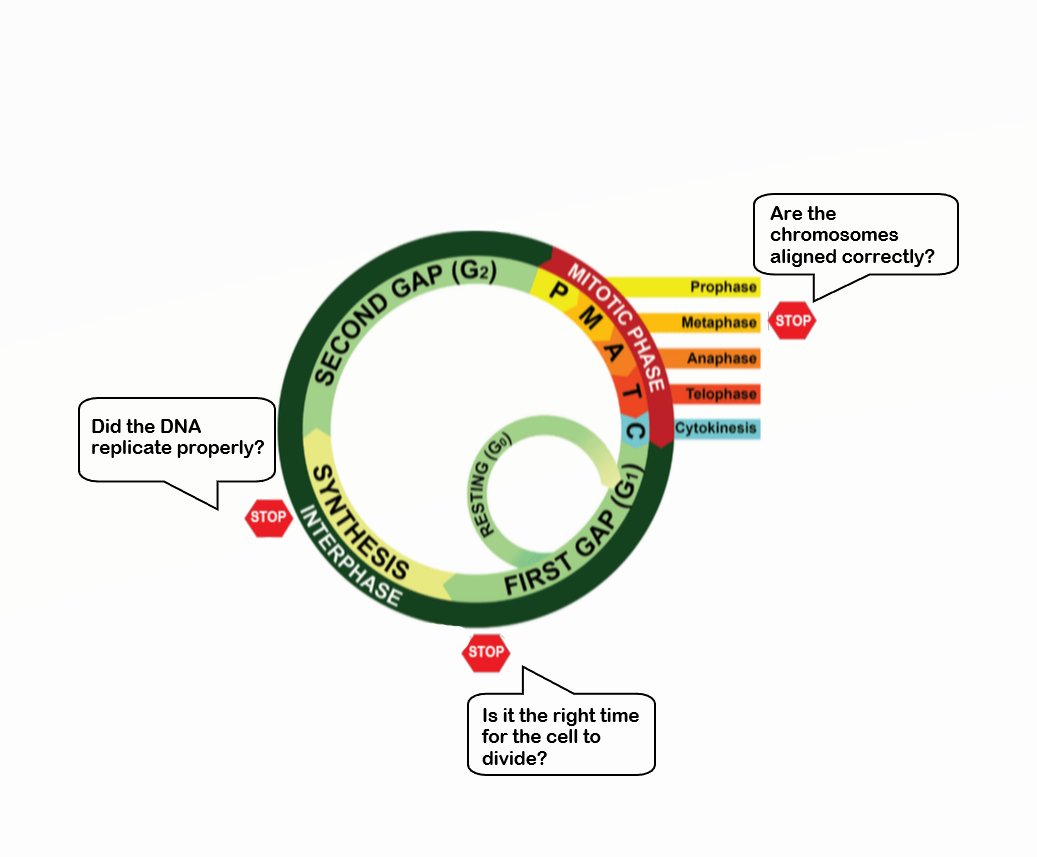
Checkpoints in the eukaryotic cell cycle ensure that the cell is ready to proceed before it moves on to the next phase of the cycle.
- The G1 checkpoint, just before entry into S phase, makes the key decision of whether the cell should divide.
- The S checkpoint determines if the DNA has been replicated properly.
- The mitosis checkpoint ensures that all the chromosomes are properly aligned before the cell is allowed to divide.
Cancer and the Cell Cycle
Cancer is a disease that occurs when the cell cycle is no longer regulated. This happens because a cell’s DNA becomes damaged. Damage can occur due to exposure to hazards, such as radiation or toxic chemicals. Cancerous cells generally divide much faster than normal cells. which may end up forming a mass of abnormal cells called a tumor (see Figure 4.12.4). The rapidly dividing cells take up nutrients and space that normal cells need. This can damage tissues and organs and eventually lead to death.
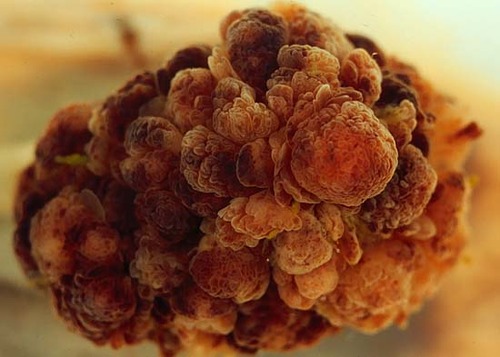
Cell Division
Cell division is the process in which one cell, called the parent cell, divides to form two new cells, referred to as daughter cells. How this happens depends on whether the cell is prokaryotic or eukaryotic. Cell division is simpler in prokaryotes than eukaryotes because prokaryotic cells themselves are simpler. Prokaryotic cells have a single circular chromosome, no nucleus, and few other organelles. Eukaryotic cells, in contrast, have multiple chromosomes contained within a nucleus and many other organelles. All of these cell parts must be duplicated and separated when the cell divides.
Before a eukaryotic cell divides, all of the DNA in the cell’s multiple chromosomes is replicated. Its organelles are also duplicated. Cell division occurs in two major steps, called mitosis and cytokinesis, both of which are described in greater detail in Chapter 5.
- The first step in the division of a eukaryotic cell is mitosis, a multi-phase process in which the nucleus of the cell divides. During mitosis, the nuclear envelope (membrane) breaks down and later reforms. The chromosomes are also sorted and separated to ensure that each daughter cell receives a complete set of chromosomes.
- The second major step is cytokinesis. This step, which also occurs in prokaryotic cells, is when the cytoplasm divides, forming two daughter cells.
Feature: Human Biology in the News
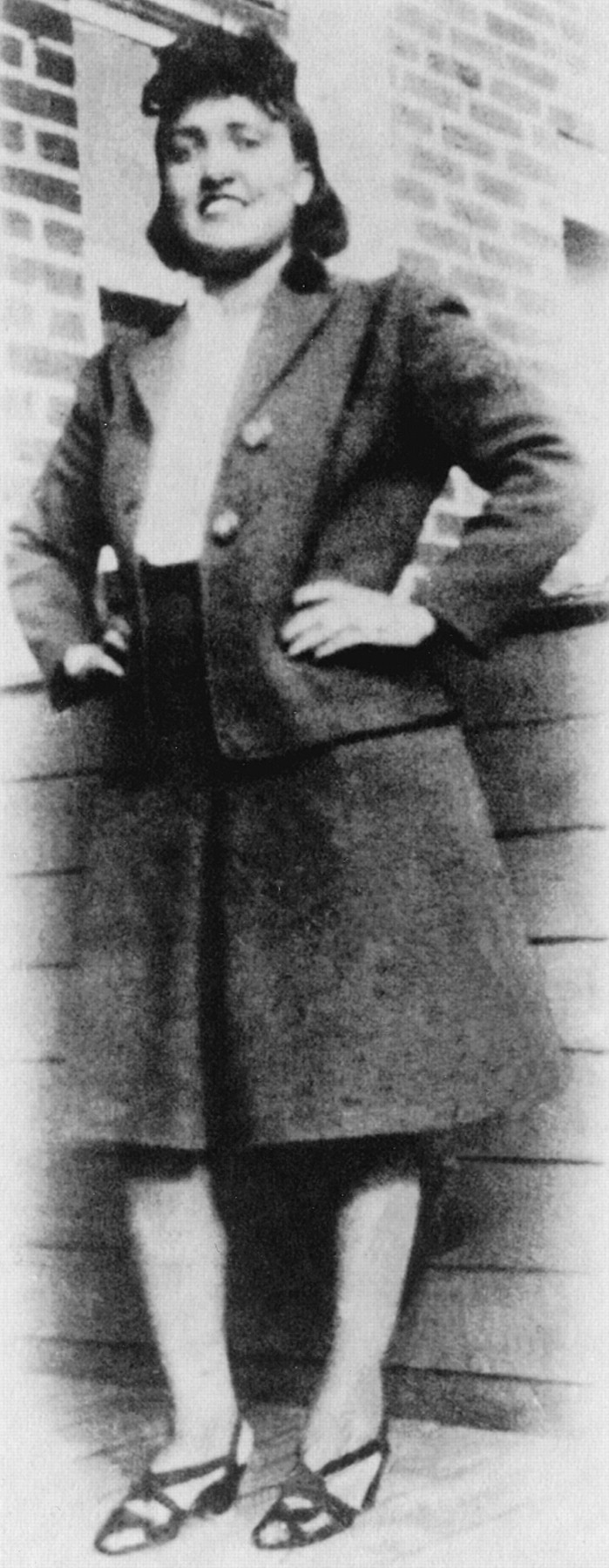
Henrietta Lacks sought treatment for her cancer at Johns Hopkins University Hospital at a time when researchers were trying to grow human cells in the lab for medical testing. Despite many attempts, the cells always died before they had undergone many cell divisions. Mrs. Lacks’s doctor, Howard Jones, took a small sample of cells from her tumor without her knowledge and gave them to a Johns Hopkins researcher, George Gey, who tried to grow them on a culture plate. For the first time in history, human cells grown on a culture plate kept dividing… and dividing and dividing and dividing. Copies of Henrietta’s cells — called HeLa cells, for her name (Henrietta Lacks) — are still alive today. In fact, there are currently billions of HeLa cells in laboratories around the world!
Why Henrietta’s cells lived on when other human cells did not is still something of a mystery, but they are clearly extremely hardy and resilient cells. By 1953, when researchers learned of their ability to keep dividing indefinitely, factories were set up to start producing the cells commercially on a large scale for medical research. Since then, HeLa cells have been used in thousands of studies and have made possible hundreds of medical advances. Jonas Salk, for example, used the cells in the early 1950s to test his polio vaccine. Over the decades since then, HeLa cells have been used to make important discoveries in the study of cancer, AIDS, and many other diseases. The cells were even sent to space on early space missions to learn how human cells respond to zero gravity. HeLa cells were also the first human cells ever cloned, and their genes were some of the first ever mapped. It is almost impossible to overestimate the profound importance of HeLa cells to human biology and medicine.
You would think that Henrietta’s name would be well known in medical history for her unparalleled contributions to biomedical research. However, until 2010, her story was virtually unknown. That year, a science writer named Rebecca Skloot published a nonfiction book, The Immortal Life of Henrietta Lacks. Based on a decade of research, this riveting account became an almost instantaneous best seller. As of 2016, Oprah Winfrey and collaborators planned to make a movie based on the book, and in recent years, numerous articles about Henrietta Lacks have appeared in the press.
Ironically, Henrietta herself never knew her cells had been taken, and neither did her family. While her cells were making a lot of money and building scientific careers, her children were living in poverty, too poor to afford medical insurance. The story of Henrietta Lacks and her immortal cells raises ethical issues about human tissues and who controls them in biomedical research. There is no question that Henrietta Lacks deserves far more recognition for her contribution to the advancement of science and medicine.
If you want to learn more about Henrietta Lacks and her immortal cells, read Rebecca Skloot’s The Immortal Life of Henrietta Lacks (or watch the movie, if it is available). You can also watch the short video below about Henrietta Lacks and her immortal cells by Robin Bulleri:
The immortal cells of Henrietta Lacks – Robin Bulleri, TED-Ed, 2016.
4.12 Summary
- The cell cycle is a repeating series of events that includes growth, DNA synthesis, and cell division. The cycle is more complicated in eukaryotic than prokaryotic cells.
- In a eukaryotic cell, the cell cycle has two major phases: mitotic phase and interphase. During mitotic phase, first the nucleus and then the cytoplasm divide. During interphase, the cell grows, performs routine life processes, and prepares to divide.
- The cell cycle is controlled mainly by regulatory proteins that signal the cell to either start or delay the next phase of the cycle. They ensure that the cell completes the previous phase before moving on. There are a number of main checkpoints in the regulation of the cell cycle.
- Cancer is a disease that occurs when the cell cycle is no longer regulated, often because the cell’s DNA has become damaged. Cancerous cells grow out of control and may form a mass of abnormal cells called a tumor.
- The cell division phase of the cell cycle in a eukaryotic cell occurs in two major steps: mitosis — when the nucleus divides — and cytokinesis, when the cytoplasm divides and two daughter cells form.
4.12 Review Questions
-
- Explain why cell division is more complex in eukaryotic than prokaryotic cells.
- Using a technique called flow cytometry, scientists can distinguish between cells with the normal amount of DNA and those that contain twice the normal amount of DNA as they go through the cell cycle. Which phases of the cell cycle will have cells with twice the amount of DNA? Explain your answer.
- What were scientists trying to do when they took tumor cells from Henrietta Lacks? Why did they specifically use tumor cells to try to achieve their goal?
4.12 Explore More
The Cell Cycle (and cancer) [Updated], The Amoeba Sisters, 2018.
Attributions
Figure 4.12.1
Mom and baby by Taiying Lu on Unsplash is used under the Unsplash License (https://unsplash.com/license).
Figure 4.12.2
Cell Cycle by LadyofHats; CK-12 Foundation is used under a CC BY-NC 3.0 (https://creativecommons.org/licenses/by-nc/3.0/) license.
©CK-12 Foundation Licensed under
• Terms of Use • Attribution
Figure 4.12.3
Cell Cycle Checkpoints by LadyofHats; CK-12 Foundation is used and adapted by Christine Miller under a CC BY-NC 3.0 (https://creativecommons.org/licenses/by-nc/3.0/) license.
©CK-12 Foundation Licensed under
• Terms of Use • Attribution
Figure 4.12.4
Cancer cells forming a tumour by Ed Uthman, MD on Wikimedia Commons is released into the public domain (https://en.wikipedia.org/wiki/Public_domain).
Figure 4.12.5
Henrietta Lacks by Oregon State University on Flickr is used under a CC BY-SA 2.0 (https://creativecommons.org/licenses/by-sa/2.0/) license.
References
Amoeba Sisters. (2018, March 20). The cell cycle (and cancer) [Updated]. YouTube. https://www.youtube.com/watch?v=QVCjdNxJreE&feature=youtu.be
TED-Ed. (2016, February 8). The immortal cells of Henrietta Lacks – Robin Bulleri. YouTube. https://www.youtube.com/watch?v=22lGbAVWhro&feature=youtu.be
Wikipedia contributors. (2020, June 23). Henrietta Lacks. In Wikipedia. https://en.wikipedia.org/w/index.php?title=Henrietta_Lacks&oldid=964020268
Wikipedia contributors. (2020, May 11). Howard W. Jones. In Wikipedia. https://en.wikipedia.org/w/index.php?title=Howard_W._Jones&oldid=956033806
Wikipedia contributors. (2020, July 1). George Otto Gey. In Wikipedia. https://en.wikipedia.org/w/index.php?title=George_Otto_Gey&oldid=965394045
Wikipedia contributors. (2020, July 6). Johns Hopkins Hospital. In ,Wikipedia. https://en.wikipedia.org/w/index.php?title=Johns_Hopkins_Hospital&oldid=966348552
Wikipedia contributors. (2020, June 28). Jonas Salk. In Wikipedia. https://en.wikipedia.org/w/index.php?title=Jonas_Salk&oldid=964883129
Wikipedia contributors. (2020, April 14). Rebecca Skloot. In Wikipedia. https://en.wikipedia.org/w/index.php?title=Rebecca_Skloot&oldid=950837115
Wikipedia contributors. (2020, February 21). The immortal life of Henrietta Lacks. In Wikipedia. https://en.wikipedia.org/w/index.php?title=The_Immortal_Life_of_Henrietta_Lacks&oldid=941942679
Created by: CK-12/Adapted by: Christine Miller
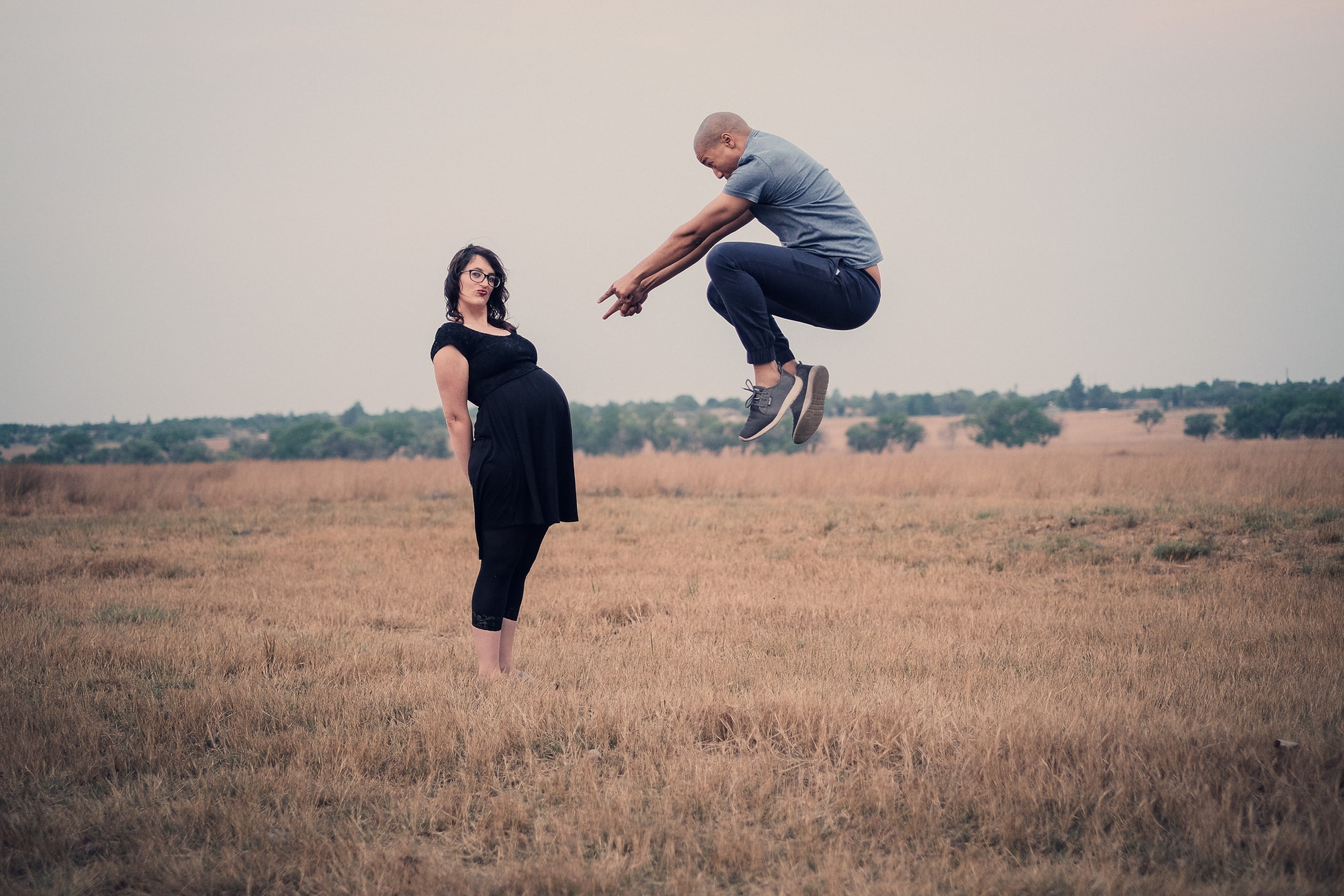
Case Study: To Give a Shot or Not
Samantha and Aki are expecting their first child. They are excited for the baby to arrive, but they are nervous, too. Will the baby be healthy? Will they be good parents? It seems like there are a million decisions to make. Will Samantha breastfeed or will they use formula? Will they buy a crib or let the baby sleep in their bed? Samantha goes online to try to find some answers. She finds a website by an author who writes books about parenting. On this site, she reads an article that argues that children should not be given many standard childhood vaccines, including the measles, mumps, and rubella (MMR) vaccine.
The article claims that the MMR vaccine has been proven to cause autism. It gives examples of three children who came down with autism-like symptoms shortly after their first MMR vaccination at one year of age. The author believes that the recent increase in the incidence of children diagnosed with autism-spectrum disorders is due to the fact that childhood vaccinations have also increased.
Samantha is concerned. She does not want to create lifelong challenges for her child by increasing his risk of autism. Besides, aren’t diseases like measles, mumps, and rubella basically eradicated by now? Why should she endanger the health of her baby by injecting him with vaccines for diseases that are a thing of the past?
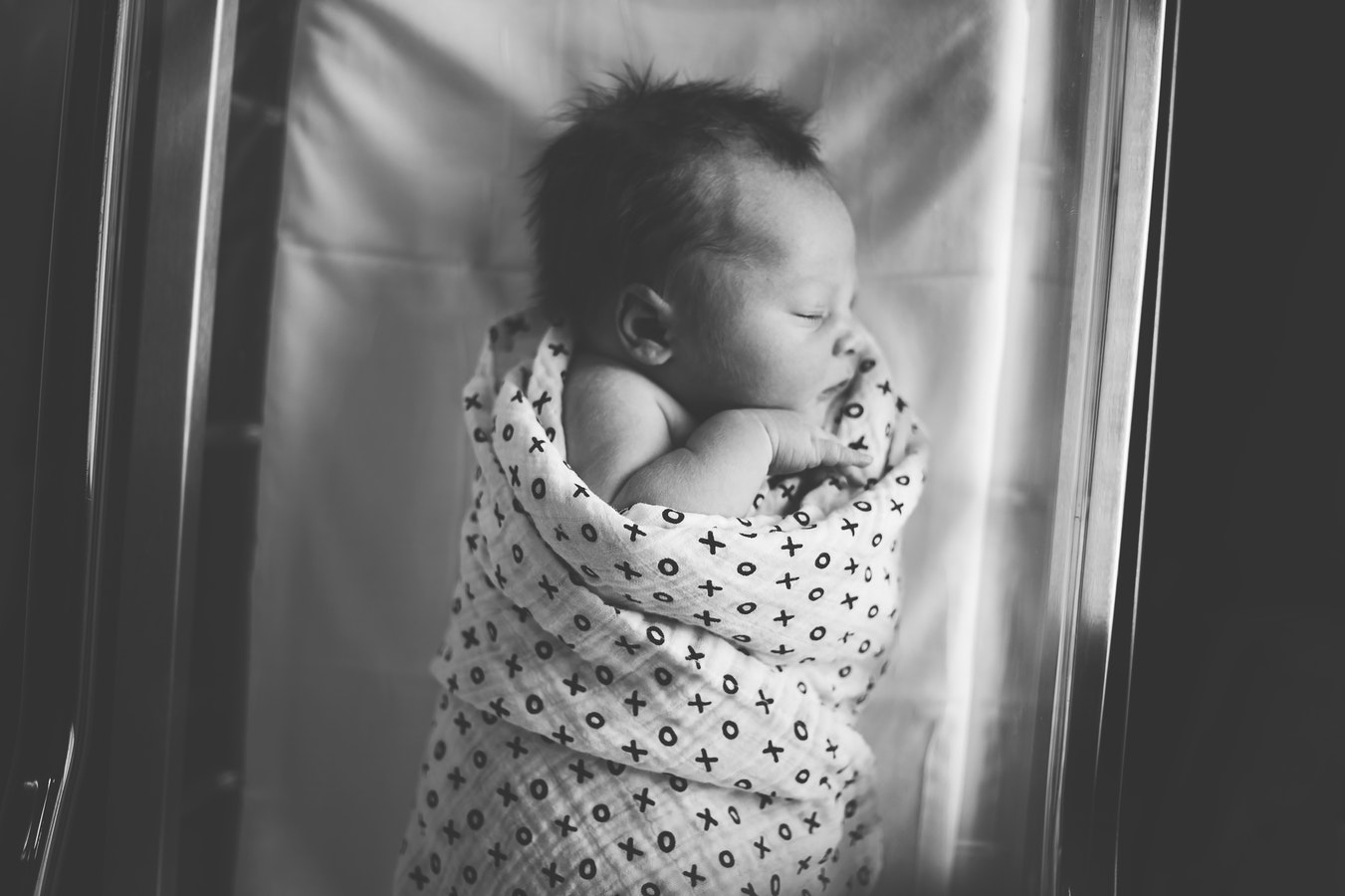
Once baby James is born, Samantha and Aki bring him to the pediatrician’s office. Dr. Rodriguez says James needs some shots. Samantha is reluctant and shares what she read online. Dr. Rodriguez assures Samantha that the study that originally claimed a link between the MMR vaccine and autism has been found to be fraudulent, and that vaccines have repeatedly been demonstrated safe and effective in peer-reviewed studies.
Although Samantha trusts her doctor, she is not fully convinced. What about the increase in the number of children with autism and the cases where symptoms of autism appeared after MMR vaccination? Samantha and Aki have a tough decision to make, but a better understanding of science can help them. In this chapter, you will learn about what science is (and what it is not), how it works, and how it relates to human health. At the end of this chapter, you will learn how Samantha and Aki use scientific evidence and reasoning to help them decide whether they should vaccinate their baby.
As you read this chapter, think about the following questions:
- How do you think the quality of Samantha’s online source of information about vaccines compares to Dr. Rodriguez’s sources?
- Do you think the arguments presented here that claim that the MMR vaccine causes autism are scientifically valid? Could there be alternative explanations for the observations?
- Why do you think diseases like measles, polio, and mumps are rare these days? Why are we still vaccinating for these diseases?
Chapter Overview: Science
In this chapter, you will learn about the nature and process of science. Specifically, you will learn about:
- What science is and the types of questions it can answer.
- How scientific knowledge advances through systematic and repeated experimentation and testing.
- How scientific ideas are open to revision, although sound scientific ideas can withstand repeated testing.
- What a scientific theory is and how it differs from common usage of the word “theory.”
- Examples of scientific breakthroughs in biology, including the development of the first vaccines, Mendel’s laws of inheritance, and the germ theory of disease.
- The scientific method, how it is used to answer scientific questions, and how it is often a nonlinear and iterative process.
- How scientific experiments are designed and carried out, including the use of controls, the manipulation of variables to test the effects on other variables, and ways to minimize sources of error.
- The importance of Traditional Ecological Knowledge and the ways in which knowledge can be collected and handed down through many generations.
- Characteristics of pseudoscience, which is defined as a claim, belief, or practice that is presented as scientific but does not adhere to scientific standards and methods.
Figure 1.1.1
Excitement by Randy Rooibaatjie on Unsplash, used under the Unsplash License (https://unsplash.com/license).
Figure 1.1.2
Baby in black and white swaddle blanket, by Julie Johnson on Unsplash, used under the Unsplash License (https://unsplash.com/license).
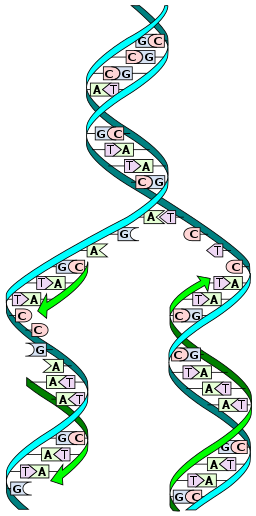
By Christine Miller
DNA Replication: Overview
DNA replication is required for the growth or replication of an organism. You started as one single cell and are now made up of approximately 37 trillion cells! Each and every one of these cells contains the exact same copy of DNA, which originated from the first cell that was you. How did you get from one set of DNA, to 37 million sets, one for each cell? Through DNA replication.
Knowledge of DNA’s structure helped scientists understand DNA replication, the process by which DNA is copied. It occurs during the synthesis (S) phase of the eukaryotic cell cycle. DNA must be copied so that each new daughter cell will have a complete set of chromosomes after cell division occurs.
DNA replication is referred to as "semi-conservative". What this means is when a strand of DNA is replicated, each of the two original strands acts as a template for a new complementary strand. When the replication process is complete, there are two identical sets of DNA, each containing one of the original strands of DNA, and one newly synthesized strand.
DNA replication involves a certain sequence of events. For each event, there is a specific enzyme which facilitates the process. There are four main enzymes that facilitate DNA replication: helicase, primase, DNA polymerase, and ligase.
DNA Replication: The Process
DNA replication begins when an enzyme called helicase unwinds, and unzips the DNA molecule. If you recall the structure of DNA, you may remember that it consists of two long strands of nucleotides held together by hydrogen bonds between complementary nitrogenous bases. This forms a ladder-like structure which is in a coiled shape. In order to start DNA replication, helicase needs to unwind the molecule and break apart the hydrogen bonds holding together complementary nitrogenous bases. This causes the two strands of DNA to separate.
Small molecules called single-stranded binding proteins (SSB) attach to the loose strands of DNA to keep them from re-forming the hydrogen bonds that helicase just broke apart.
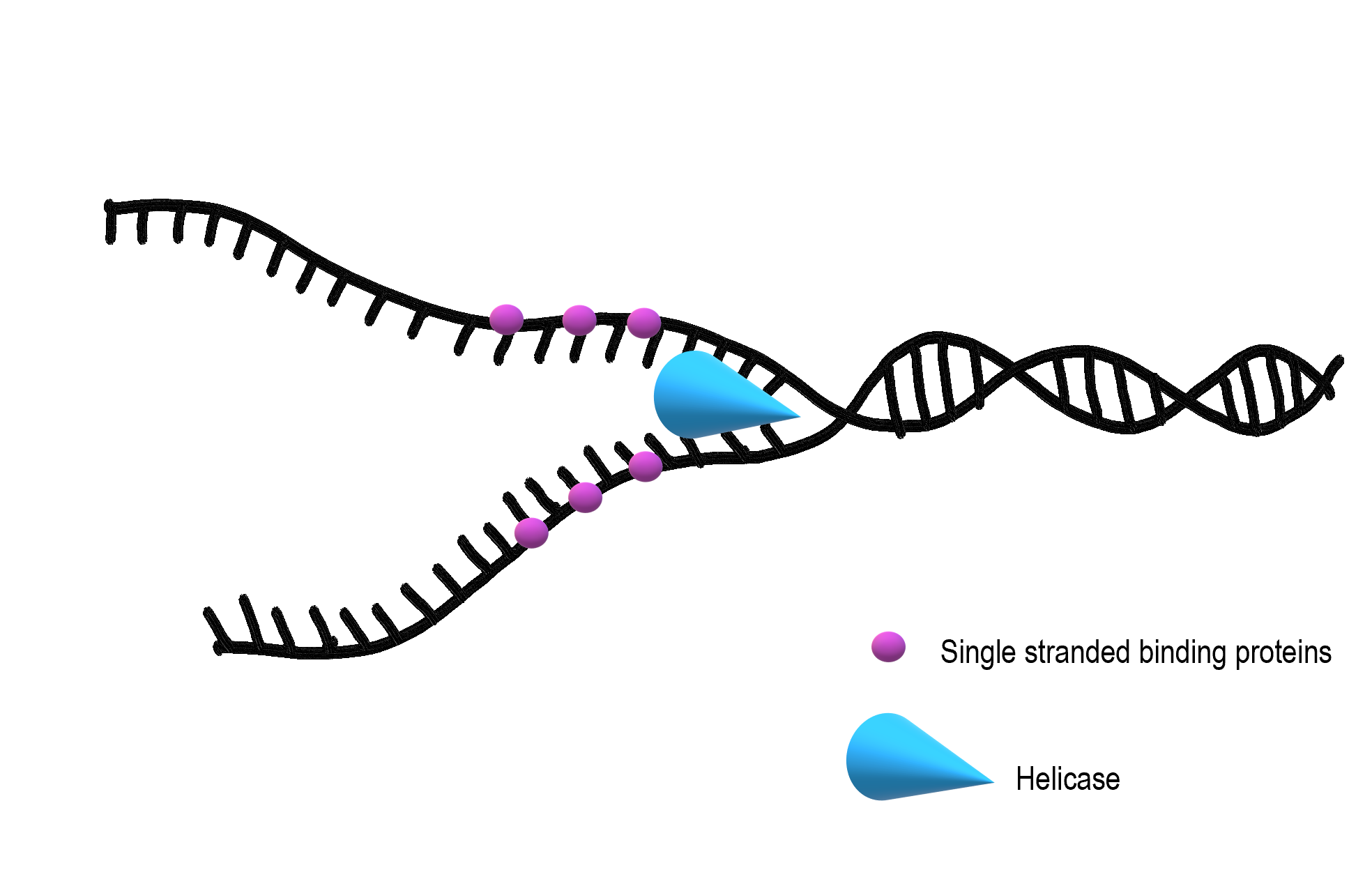
Once the nitrogenous bases from the inside of the DNA molecule are exposed, the creation of a new, complementary strand can begin. DNA polymerase creates the new strand, but it needs some help in finding the correct place to begin, so primase lays down a short section of RNA primer (shown in green in Figure 5.4.3). Once this short section of primer is laid, DNA polymerase can bind to the DNA molecule and start connecting nucleotides in the correct order to match the sequence of nitrogenous bases on the template (original) strand.
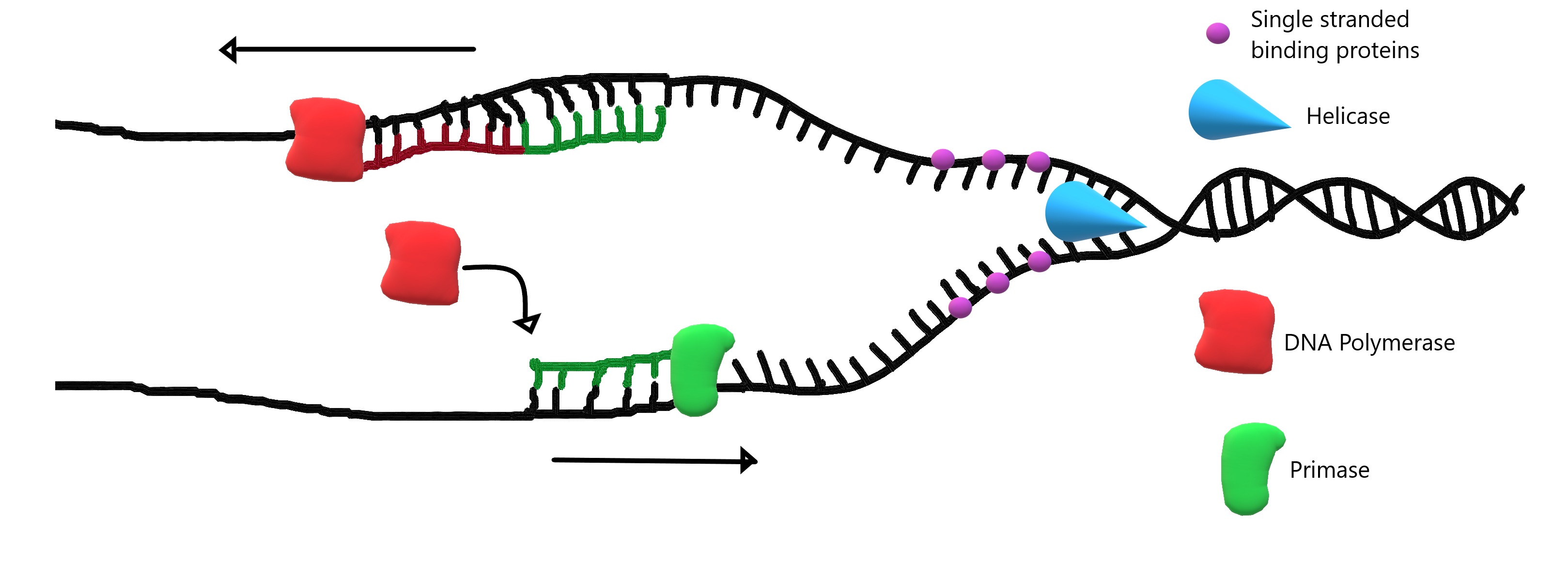
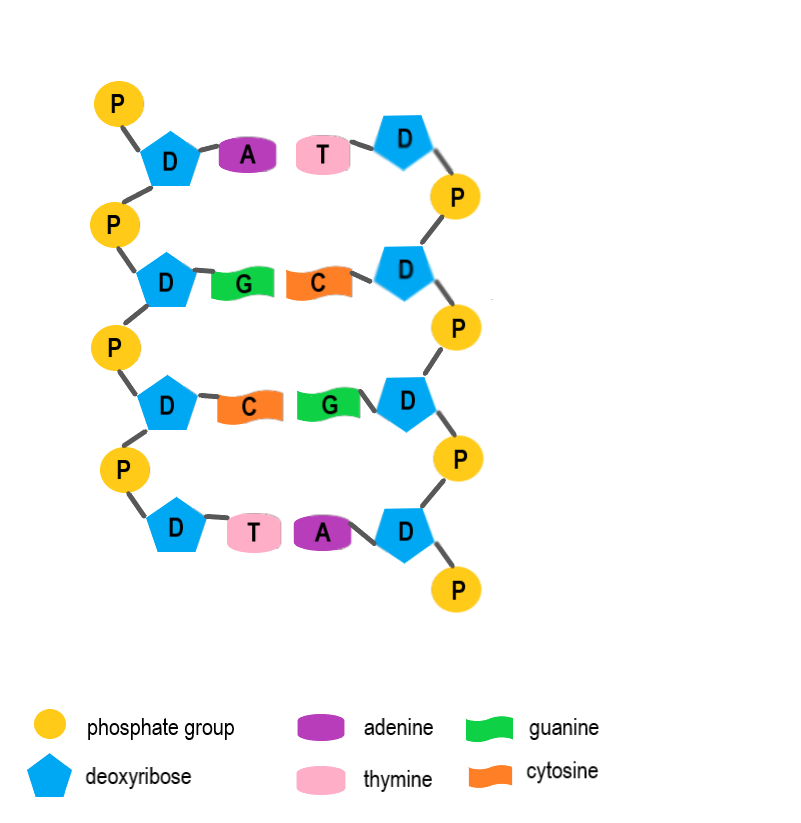
If we think about the DNA molecule, we may remember that the two strands of DNA run antiparallel to one another. This means that in the sugar-phosphate backbone, one strand of the DNA has the sugar oriented in the "up" position, and the other strand has the phosphate oriented in the "up" position (see Figure 5.4.4). DNA polymerase is an enzyme which can only work in one direction on the DNA molecule. This means that one strand of DNA can be replicated in one long string, as DNA polymerase follows helicase as it unzips the DNA molecule. This strand is termed the "leading strand". The other strand, however, can only be replicated in small chunks since the DNA polymerase replicates in the opposite direction that helicase is unzipping. This strand is termed the "lagging strand". These small chunks of replicated DNA on the lagging strand are called Okazaki fragments.
Take a look at Figure 5.4.5 and find the Okazaki fragments, the leading strand and the lagging strand.
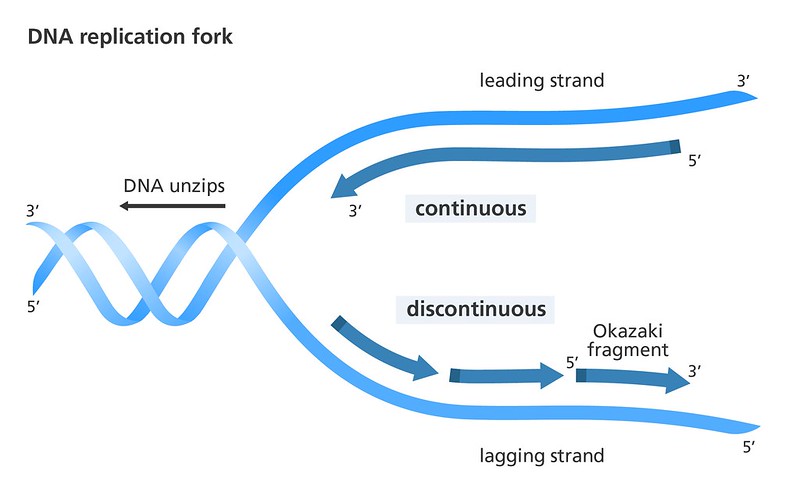
Once DNA polymerase has replicated the DNA, a third enzyme called ligase completes the final stage of DNA replication, which is repairing the sugar-phosphate backbone. This connects the gaps in the backbone between Okazaki fragments. Once this is complete, the DNA coils back into its classic double helix structure.
Semi-Conservative Replication
When DNA replication is complete, there are two identical sets of double stranded DNA, each with one strand from the original, template, DNA molecule, and one strand that was newly synthesized during the DNA replication process. Because each new set of DNA contains one old and one new strand, we describe DNA as being semi-conservative.
Watch this video for a great overview of DNA replication:
https://www.youtube.com/watch?v=Qqe4thU-os8
DNA Replication (Updated), Amoeba Sisters, 2019.
5.4 Summary
- DNA replication requires the action of three main enzymes each with their own specific role:
- Helicase unzips and unwinds the DNA molecule.
- DNA polymerase creates a new complementary strand of DNA on each of the originals halves that were separated by helicase. New nucleotides are added through complementary base pairing: A pairs with T, and C with G.
- Ligase repairs gaps in the sugar-phosphate backbone between Okazaki fragments.
- DNA replication is semi-conservative because each daughter molecule contains one strand from the parent molecule and one new complementary strand.
5.4 Review Questions
2. Why are Okazaki fragments formed?
- Because helicase only unzips DNA in one direction.
- Because DNA is in a double helix.
- Because DNA polymerase only replicates DNA in one direction.
- Because DNA replication is semi-conservative.
3. Drag and drop to label the diagram.
5.4 Explore More
https://www.youtube.com/watch?v=TNKWgcFPHqw
DNA replication - 3D, yourgenome, 2015.
Attributions
Figure 5.4.1
DNA_replication_split.svg by Madprime on Wikimedia Commons is used under a CC0 1.0
Public Domain Dedication license (https://creativecommons.org/publicdomain/zero/1.0/deed.en).
Figure 5.4.2
Helicase and single stranded binding proteins (1) by Christine Miller is used under a CC BY 4.0 (https://creativecommons.org/licenses/by/4.0/) license.
Figure 5.4.3
DNA polymerase and primase by Christine Miller is used under a CC BY 4.0 (https://creativecommons.org/licenses/by/4.0/) license.
Figure 5.4.4
DNA strands run antiparallel by Christine Miller is used under a CC BY 4.0 (https://creativecommons.org/licenses/by/4.0/) license.
Figure 5.4.5
Leading and lagging strand/ DNA Replication/ by yourgenome on Flickr is used under a CC BY-NC-SA 2.0 (https://creativecommons.org/licenses/by-nc-sa/2.0/) license.
References
Amoeba Sisters. (2019, June 28). DNA replication (Updated). YouTube. https://www.youtube.com/watch?v=Qqe4thU-os8&feature=youtu.be
Betts, J. G., Young, K.A., Wise, J.A., Johnson, E., Poe, B., Kruse, D.H., Korol, O., Johnson, J.E., Womble, M., DeSaix, P. (2013, April 25). Figure 3.24 DNA Replication [digital image]. In Anatomy and Physiology. OpenStax. https://openstax.org/books/anatomy-and-physiology/pages/3-3-the-nucleus-and-dna-replication CC BY 4.0 (https://creativecommons.org/licenses/by/4.0/)
yourgenome. (2015, June 26). DNA replication - 3D. YouTube. https://www.youtube.com/watch?v=TNKWgcFPHqw&feature=youtu.be
Created by: CK-12/Adapted by Christine Miller
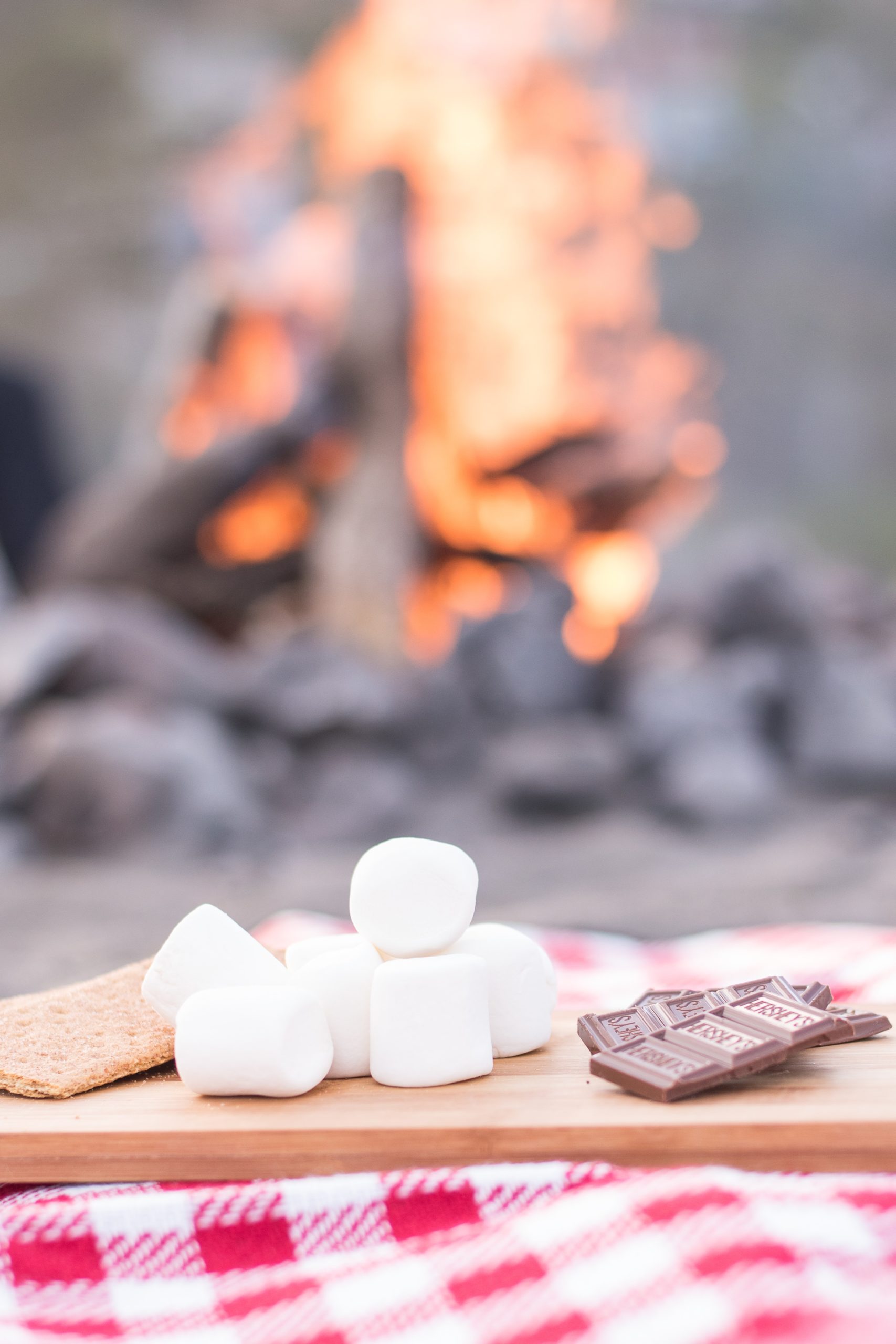
Bring on the S'mores!
This inviting camp fire can be used for both heat and light. Heat and light are two forms of energy that are released when a fuel like wood is burned. The cells of living things also get energy by "burning." They "burn" glucose in a process called cellular respiration.
What Is Cellular Respiration?
Cellular respiration is the process by which living cells break down glucose molecules and release energy. The process is similar to burning, although it doesn’t produce light or intense heat as a campfire does. This is because cellular respiration releases the energy in glucose slowly and in many small steps. It uses the energy released to form molecules of ATP, the energy-carrying molecules that cells use to power biochemical processes. In this way, cellular respiration is an example of energy coupling: glucose is broken down in an exothermic reaction, and then the energy from this reaction powers the endothermic reaction of the formation of ATP. Cellular respiration involves many chemical reactions, but they can all be summed up with this chemical equation:
C6H12O6 6O2 → 6CO2 6H2O Chemical Energy (in ATP)
In words, the equation shows that glucose (C6H12O6) and oxygen (O2) react to form carbon dioxide (CO2) and water (H2O), releasing energy in the process. Because oxygen is required for cellular respiration, it is an aerobic process.
Cellular respiration occurs in the cells of all living things, both autotrophs and heterotrophs. All of them burn glucose to form ATP. The reactions of cellular respiration can be grouped into three stages: glycolysis, the citric acid cycle (also called the Kreb's cycle), and electron transport. Figure 4.10.2 gives an overview of these three stages, which are also described in detail below.
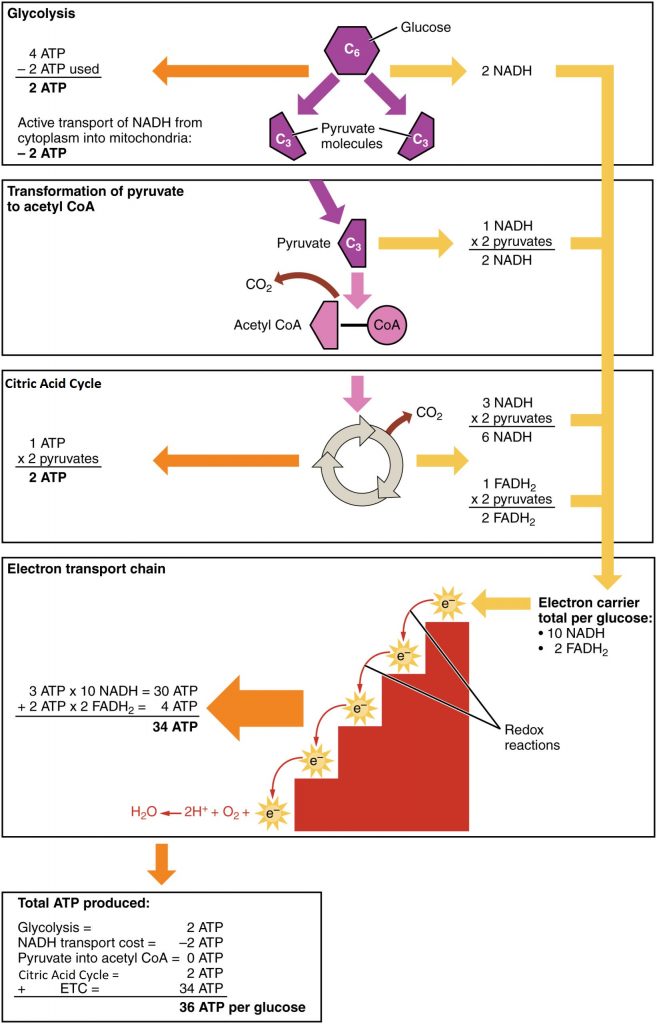
Cellular Respiration Stage I: Glycolysis
The first stage of cellular respiration is glycolysis, which happens in the cytosol of the cytoplasm.
Splitting Glucose
The word glycolysis literally means “glucose splitting,” which is exactly what happens in this stage. Enzymes split a molecule of glucose into two molecules of pyruvate (also known as pyruvic acid). This occurs in several steps; the result is summarized in the following diagram.
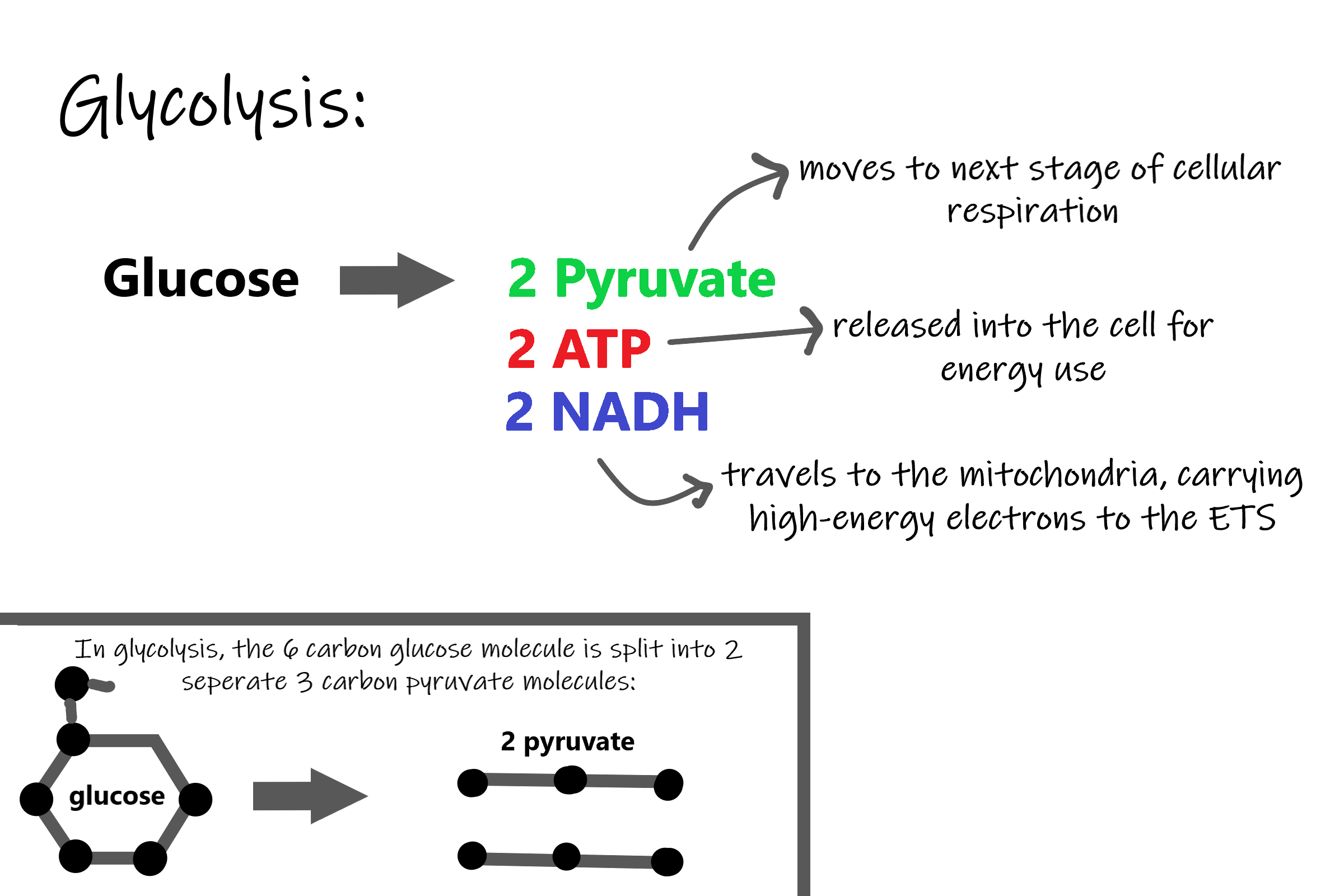
Results of Glycolysis
Energy is needed at the start of glycolysis to split the glucose molecule into two pyruvate molecules which go on to stage II of cellular respiration. The energy needed to split glucose is provided by two molecules of ATP; this is called the energy investment phase. As glycolysis proceeds, energy is released, and the energy is used to make four molecules of ATP; this is the energy harvesting phase. As a result, there is a net gain of two ATP molecules during glycolysis. During this stage, high-energy electrons are also transferred to molecules of NAD to produce two molecules of NADH, another energy-carrying molecule. NADH is used in stage III of cellular respiration to make more ATP.
Transition Reaction
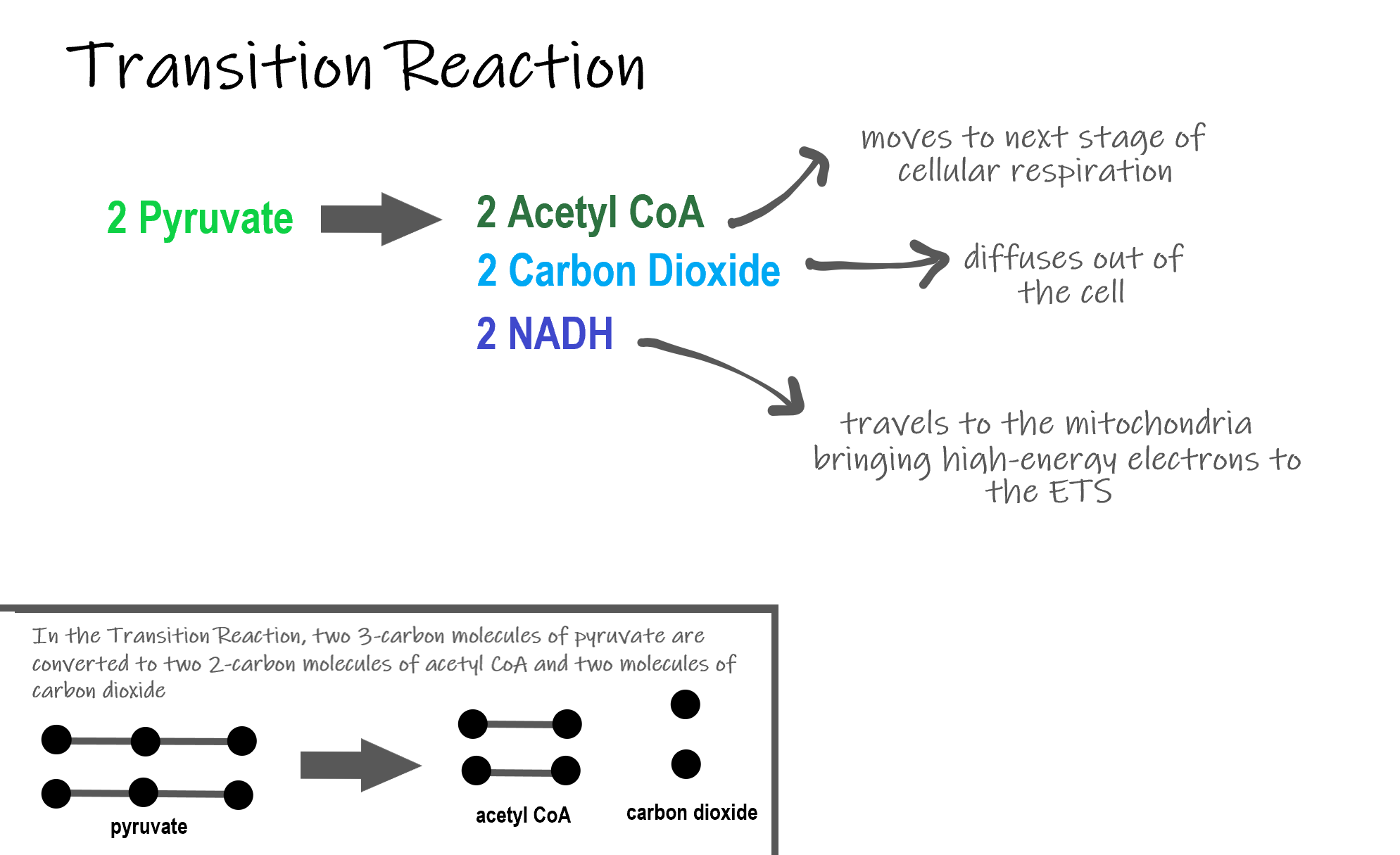
Before pyruvate can enter the next stage of aerobic cellular respiration in the mitochondrion it needs to be modified slightly. The transition reaction is a very short reaction which converts the two molecules of pyruvate to two molecules of acetyl CoA, carbon dioxide, and two high energy electron pairs convert NAD to NADH. The carbon dioxide is released, the acetyl CoA enters the Kreb's Cycle (stage II), and the NADH carries the high energy electrons to the Electron Transport System (stage III).
Structure of the Mitochondrion
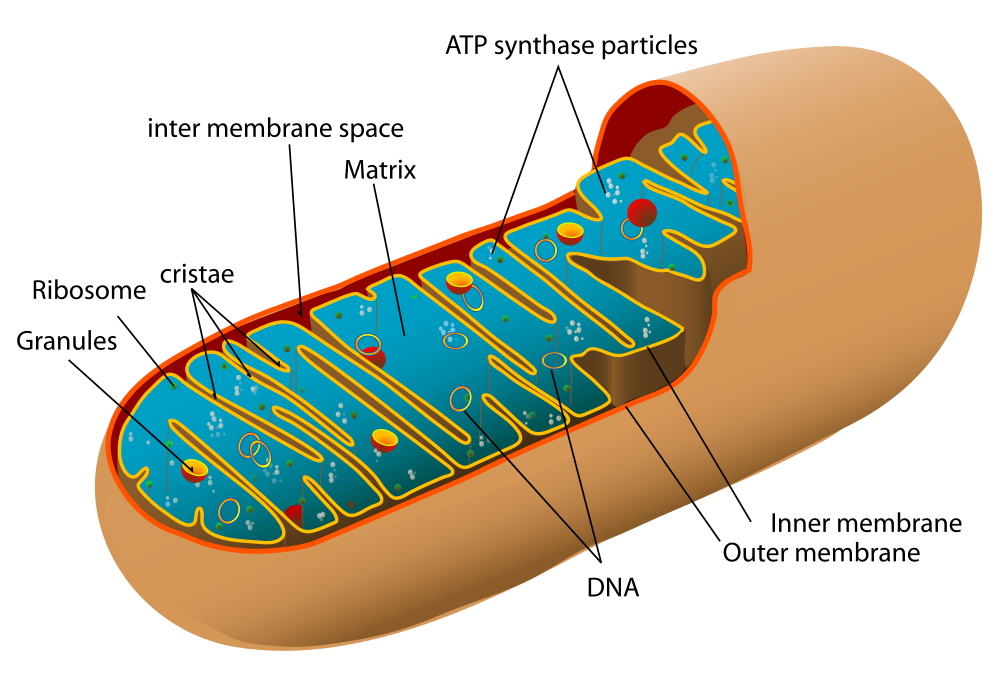
Before you read about the last two stages of cellular respiration, you need to know more about the mitochondrion, where these two stages take place. A diagram of a mitochondrion is shown in Figure 4.10.5.
The structure of a mitochondrion is defined by an inner and outer membrane. This structure plays an important role in aerobic respiration.
As you can see from the figure, a mitochondrion has an inner and outer membrane. The space between the inner and outer membrane is called the intermembrane space. The space enclosed by the inner membrane is called the matrix. The second stage of cellular respiration (the Krebs cycle) takes place in the matrix. The third stage (electron transport) happens on the inner membrane.
Cellular Respiration Stage II: The Krebs Cycle
Recall that glycolysis produces two molecules of pyruvate (pyruvic acid), which are then converted to acetyl CoA during the short transition reaction. These molecules enter the matrix of a mitochondrion, where they start the Krebs cycle (also known as the Citric Acid Cycle). The reason this stage is considered a cycle is because a molecule called oxaloacetate is present at both the beginning and end of this reaction and is used to break down the two molecules of acetyl CoA. The reactions that occur next are shown in Figure 4.10.6.
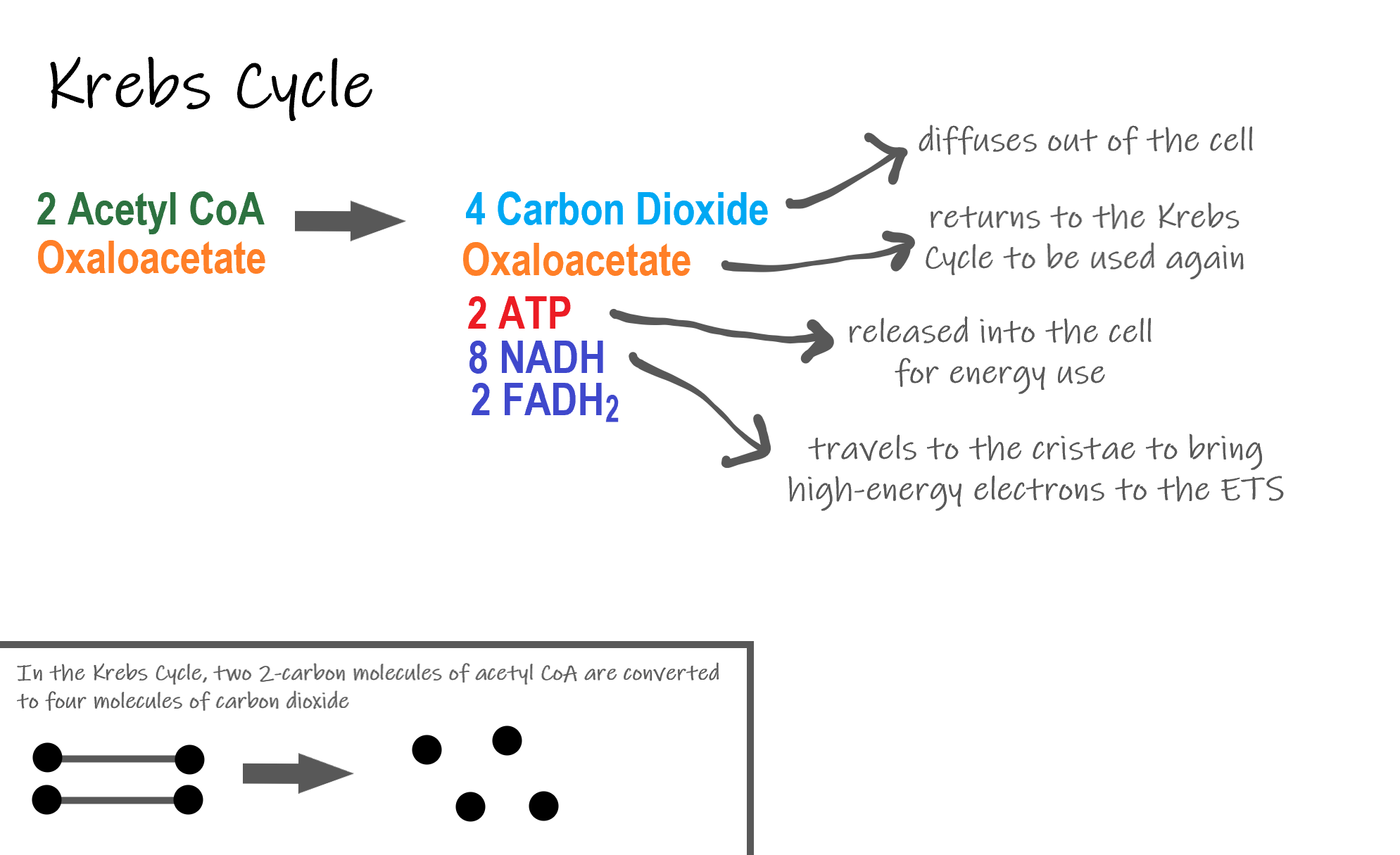
Steps of the Krebs Cycle
The Krebs cycle itself actually begins when acetyl-CoA combines with a four-carbon molecule called OAA (oxaloacetate) (see Figure 4.10.6). This produces citric acid, which has six carbon atoms. This is why the Krebs cycle is also called the citric acid cycle.
After citric acid forms, it goes through a series of reactions that release energy. The energy is captured in molecules of NADH, ATP, and FADH2, another energy-carrying coenzyme. Carbon dioxide is also released as a waste product of these reactions.
The final step of the Krebs cycle regenerates OAA, the molecule that began the Krebs cycle. This molecule is needed for the next turn through the cycle. Two turns are needed because glycolysis produces two pyruvic acid molecules when it splits glucose.
Results of the Glycolysis, Transition Reaction and Krebs Cycle
After glycolysis, transition reaction, and the Krebs cycle, the glucose molecule has been broken down completely. All six of its carbon atoms have combined with oxygen to form carbon dioxide. The energy from its chemical bonds has been stored in a total of 16 energy-carrier molecules. These molecules are:
- 4 ATP (2 from glycolysis, 2 from Krebs Cycle)
- 12 NADH (2 from glycolysis, 2 from transition reaction, and 8 from Krebs cycle)
- 2 FADH2 (both from the Krebs cycle)
The events of cellular respiration up to this point are exergonic reactions- they are releasing energy that had been stored in the bonds of the glucose molecule. This energy will be transferred to the third and final stage of cellular respiration: the Electron Transport System, which is an endergonic reaction. Using an exothermic reaction to power an endothermic reaction is known as energy coupling.
Cellular Respiration Stage III: Electron Transport Chain
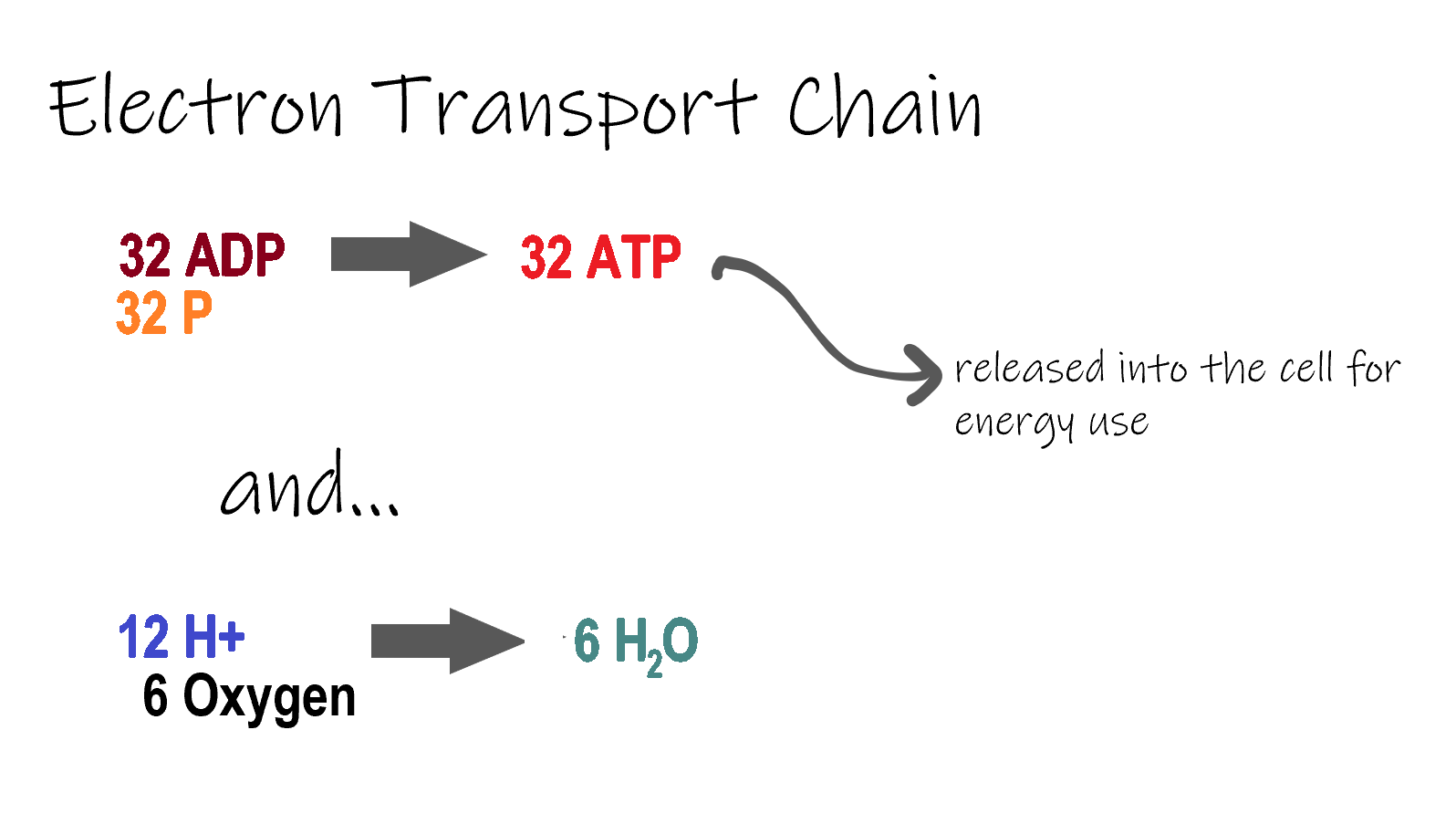
ETC, the final stage in cellular respiration produces 32 ATP. The Electron Transport Chain is the final stage of cellular respiration. In this stage, energy being transported by NADH and FADH2 is transferred to ATP. In addition, oxygen acts as the final proton acceptor for the hydrogens released from all the NADH and FADH2, forming water. Figure 4.10.8 shows the reactants and products of the ETC.
Transporting Electrons
The Electron transport chain is the third stage of cellular respiration and is illustrated in Figure 4.10.8. During this stage, high-energy electrons are released from NADH and FADH2, and they move along electron-transport chains on the inner membrane of the mitochondrion. An electron-transport chain is a series of molecules that transfer electrons from molecule to molecule by chemical reactions. Some of the energy from the electrons is used to pump hydrogen ions (H ) across the inner membrane, from the matrix into the intermembrane space. This ion transfer creates an electrochemical gradient that drives the synthesis of ATP.
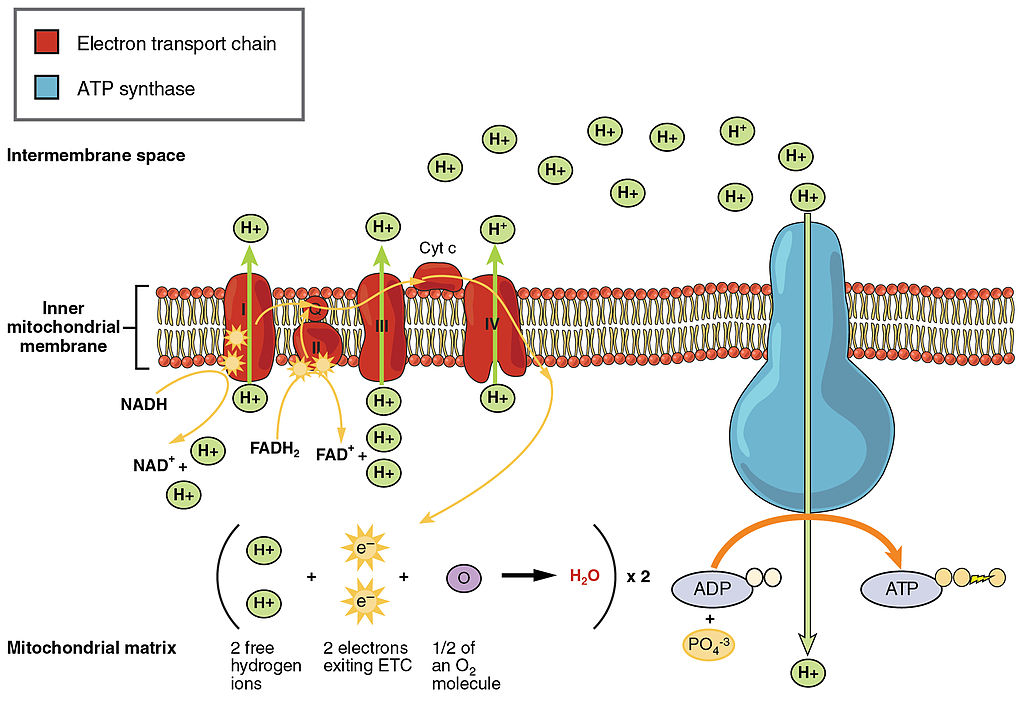
Making ATP
As shown in Figure 4.10.8, the pumping of hydrogen ions across the inner membrane creates a greater concentration of the ions in the intermembrane space than in the matrix. This gradient causes the ions to flow back across the membrane into the matrix, where their concentration is lower. ATP synthase acts as a channel protein, helping the hydrogen ions cross the membrane. It also acts as an enzyme, forming ATP from ADP and inorganic phosphate in a process called oxidative phosphorylation. After passing through the electron-transport chain, the “spent” electrons combine with oxygen to form water.
How Much ATP?
You have seen how the three stages of aerobic respiration use the energy in glucose to make ATP. How much ATP is produced in all three stages combined? Glycolysis produces two ATP molecules, and the Krebs cycle produces two more. Electron transport begins with several molecules of NADH and FADH2 from the Krebs cycle and transfers their energy into as many as 34 more ATP molecules. All told, then, up to 38 molecules of ATP can be produced from just one molecule of glucose in the process of cellular respiration.
4.10 Summary
- Cellular respiration is the aerobic process by which living cells break down glucose molecules, release energy, and form molecules of ATP. Generally speaking, this three-stage process involves glucose and oxygen reacting to form carbon dioxide and water.
- The first stage of cellular respiration, called glycolysis, takes place in the cytoplasm. In this step, enzymes split a molecule of glucose into two molecules of pyruvate, which releases energy that is transferred to ATP. Following glycolysis, a short reaction called the transition reaction converts the pyruvate into two molecules of acetyl CoA.
- The organelle called a mitochondrion is the site of the other two stages of cellular respiration. The mitochondrion has an inner and outer membrane separated by an intermembrane space, and the inner membrane encloses a space called the matrix.
- The second stage of cellular respiration, called the Krebs cycle, takes place in the matrix of a mitochondrion. During this stage, two turns through the cycle result in all of the carbon atoms from the two pyruvate molecules forming carbon dioxide and the energy from their chemical bonds being stored in a total of 16 energy-carrying molecules (including two from glycolysis and two from transition reaction).
- The third and final stage of cellular respiration, called electron transport, takes place on the inner membrane of the mitochondrion. Electrons are transported from molecule to molecule down an electron-transport chain. Some of the energy from the electrons is used to pump hydrogen ions across the membrane, creating an electrochemical gradient that drives the synthesis of many more molecules of ATP.
- In all three stages of cellular respiration combined, as many as 38 molecules of ATP are produced from just one molecule of glucose.
4.10 Review Questions
- What is the purpose of cellular respiration? Provide a concise summary of the process.
- State what happens during glycolysis.
- Describe the structure of a mitochondrion.
- What molecule is present at both the beginning and end of the Krebs cycle?
- What happens during the electron transport stage of cellular respiration?
- How many molecules of ATP can be produced from one molecule of glucose during all three stages of cellular respiration combined?
- Do plants undergo cellular respiration? Why or why not?
- Explain why the process of cellular respiration described in this section is considered aerobic.
- Name three energy-carrying molecules involved in cellular respiration.
-
- Which stage of aerobic cellular respiration produces the most ATP?
-
4.10 Explore More
https://www.youtube.com/watch?time_continue=2&v=00jbG_cfGuQ&feature=emb_logo
ATP & Respiration: Crash Course Biology #7, CrashCourse, 2012.
https://www.youtube.com/watch?v=4Eo7JtRA7lg&t=3s
Cellular Respiration and the Mighty Mitochondria, The Amoeba Sisters, 2014.
Attributions
Figure 4.10.1
Smores by Jessica Ruscello on Unsplash is used under the Unsplash License (https://unsplash.com/license).
Figure 4.10.2
Carbohydrate_Metabolism by OpenStax College on Wikimedia Commons is used under a CC BY 3.0 (https://creativecommons.org/licenses/by/3.0) license.
Figure 4.10.3
Glycolysis by Christine Miller is used under a CC BY 4.0 (https://creativecommons.org/licenses/by/4.0/) license.
Figure 4.10.4
Transition Reaction by Christine Miller is used under a CC BY 4.0 (https://creativecommons.org/licenses/by/4.0/) license.
Figure 4.10.5
Mitochondrion by Mariana Ruiz Villarreal [LadyofHats] on Wikimedia Commons is released into the public domain (https://en.wikipedia.org/wiki/Public_domain).
Figure 4.10.6
Krebs cycle by Christine Miller is used under a CC BY 4.0 (https://creativecommons.org/licenses/by/4.0/) license.
Figure 4.10.7
Electron Transport Chain (ETC) by Christine Miller is used under a CC BY 4.0 (https://creativecommons.org/licenses/by/4.0/) license.
Figure 4.10.8
The_Electron_Transport_Chain by OpenStax College on Wikimedia Commons is used under a CC BY 3.0 (https://creativecommons.org/licenses/by/3.0) license.
References
CrashCourse. (2012, March 12). ATP & Respiration: Crash Course Biology #7. YouTube. https://www.youtube.com/watch?time_continue=2&v=00jbG_cfGuQ&feature=emb_logo
Betts, J. G., Young, K.A., Wise, J.A., Johnson, E., Poe, B., Kruse, D.H., Korol, O., Johnson, J.E., Womble, M., DeSaix, P. (2013, April 25). Figure 24.8 Electron Transport Chain [digital image]. In Anatomy & Physiology, Connexions (Section ). OpenStax. https://openstax.org/books/anatomy-and-physiology/pages/24-2-carbohydrate-metabolism
Betts, J. G., Young, K.A., Wise, J.A., Johnson, E., Poe, B., Kruse, D.H., Korol, O., Johnson, J.E., Womble, M., DeSaix, P. (2013, April 25). Figure 24.9 Carbohydrate Metabolism [digital image]. In Anatomy & Physiology, Connexions (Section 24.2). OpenStax. https://openstax.org/books/anatomy-and-physiology/pages/24-2-carbohydrate-metabolism
The Amoeba Sisters. (2014, October 22). Cellular Respiration and the Mighty Mitochondria. YouTube. https://www.youtube.com/watch?v=4Eo7JtRA7lg&t=3s
Created by: CK-12/Adapted by: Christine Miller
"Doing" Science
Science is as much about doing as knowing. Scientists are always trying to learn more and gain a better understanding of the natural world. There are basic methods of gaining knowledge that are common to all of science. At the heart of science is the scientific investigation. A scientific investigation is a systematic approach to answering questions about the physical and natural world. Scientific investigations can be observational — for example, observing a cell under a microscope and recording detailed descriptions. Other scientific investigations are experimental — for example, treating a cell with a drug while recording changes in the behavior of the cell.
The flow chart below shows the typical steps followed in an experimental scientific investigation. The series of steps shown in the flow chart is frequently referred to as the scientific method. Science textbooks often present this simple, linear "recipe" for a scientific investigation. This is an oversimplification of how science is actually done, but it does highlight the basic plan and purpose of an experimental scientific investigation: testing ideas with evidence. Each of the steps in the flow chart is discussed in greater detail below.
![By Thebiologyprimer [CC0], from Wikimedia Commons Diagram shows the scientific cycle arranged in a circular formation: Observation, questions, hypothesis, experiment, analysis, conclusion and then returning to observation again.](https://pressbooks.bccampus.ca/humanbiology053/wp-content/uploads/sites/1414/2019/06/The_Scientific_Method_simple-300x280.png)
Science is actually a complex endeavor that cannot be reduced to a single, linear sequence of steps, like the instructions on a package of cake mix. Real science is nonlinear, iterative (repetitive), creative, unpredictable, and exciting. Scientists often undertake the steps of an investigation in a different sequence, or they repeat the same steps many times as they gain more information and develop new ideas. Scientific investigations often raise new questions as old ones are answered. Successive investigations may address the same questions, but at ever deeper levels. Alternatively, an investigation might lead to an unexpected observation that sparks a new question and takes the research in a completely different direction.
Knowing how scientists "do" science can help you in your everyday life, even if you aren't a scientist. Some steps of the scientific process — such as asking questions and evaluating evidence — can be applied to answering real-life questions and solving practical problems.
Making Observations
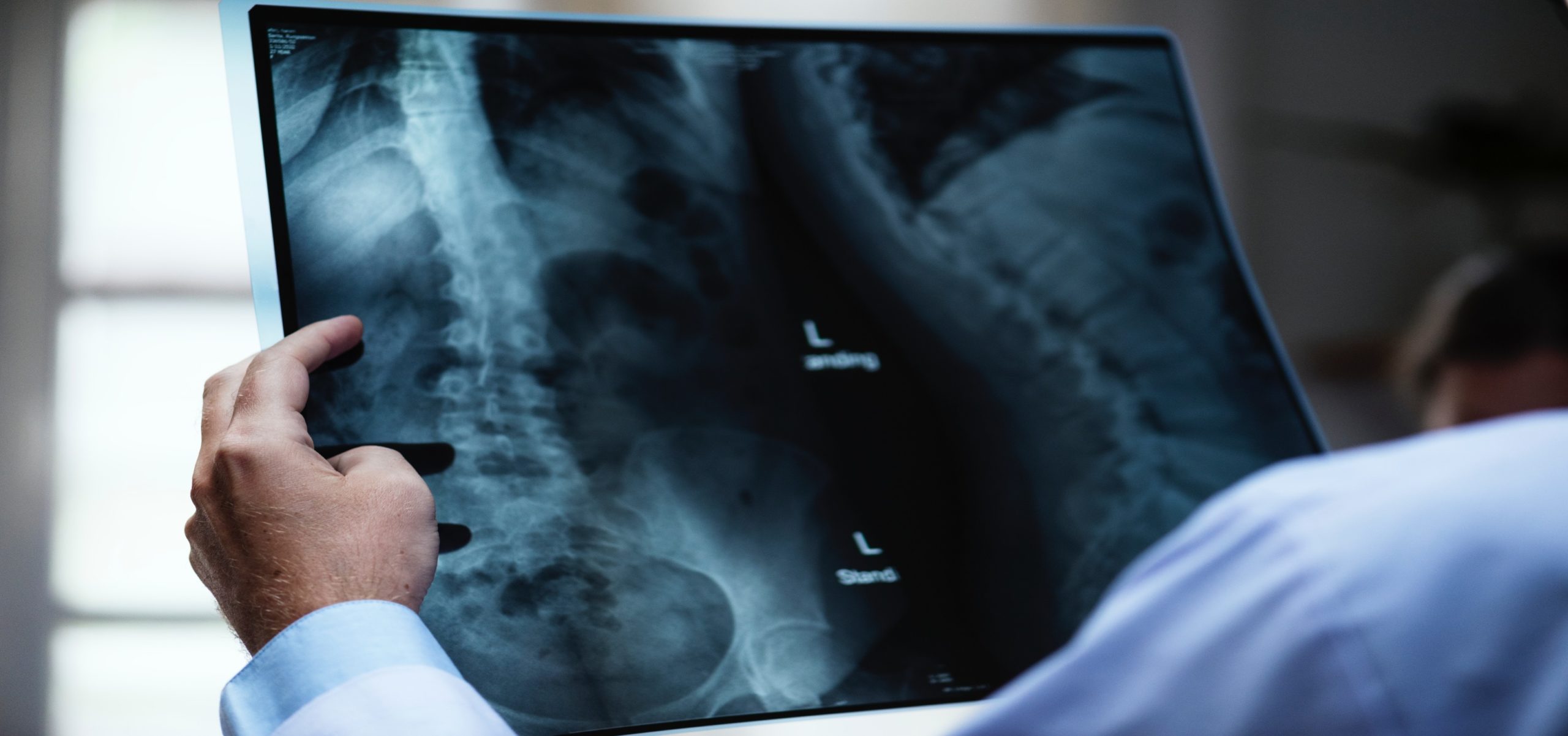
Testing an idea typically begins with observations. An observation is anything that is detected through human senses or with instruments or measuring devices that enhance human senses. We usually think of observations as things we see with our eyes, but we can also make observations with our sense of touch, smell, taste, or hearing. In addition, we can extend and improve our own senses with instruments such as thermometers and microscopes. Other instruments can be used to sense things that human senses cannot detect at all, such as ultraviolet light or radio waves.
![Photo by Ministry of Information Photo Division Photographer [Public domain], via Wikimedia Commons A black and white photo of Alexander Fleming examining bacterial growth on a petri dish.](https://pressbooks.bccampus.ca/humanbiology053/wp-content/uploads/sites/1414/2021/06/Alexander-Flemming-300x251.jpg)
Sometimes, chance observations lead to important scientific discoveries. One such observation was made by the Scottish biologist Alexander Fleming (pictured below) in the 1920s. Fleming's name may sound familiar to you because he is famous for a major discovery. Fleming had been growing a certain type of bacteria on glass plates in his lab when he noticed that one of the plates was contaminated with mold. On closer examination, Fleming observed that the area around the mold was free of bacteria.
Asking Questions
Observations often lead to interesting questions. This is especially true if the observer is thinking like a scientist. Having scientific training and knowledge is also useful. Relevant background knowledge and logical thinking help make sense of observations so the observer can form particularly salient questions. Fleming, for example, wondered whether the mold — or some substance it produced — had killed bacteria on the plate. Fortunately for us, Fleming didn't just throw out the mold-contaminated plate. Instead, he investigated his question and in so doing, discovered the antibiotic penicillin.
Hypothesis Formation
Typically, the next step in a scientific investigation is to form a hypothesis. A hypothesis is a possible answer to a scientific question. But it isn’t just any answer. A hypothesis must be based on scientific knowledge. In other words, it shouldn't be at odds with what is already known about the natural world. A hypothesis also must be logical, and it is beneficial if the hypothesis is relatively simple. In addition, to be useful in science, a hypothesis must be testable and falsifiable. In other words, it must be possible to subject the hypothesis to a test that generates evidence for or against it. It must also be possible to make observations that would disprove the hypothesis if it really is false.
For example, Fleming's hypothesis might have been: “A particular kind of bacteria growing on a plate will die when exposed to a particular kind of mold.” The hypothesis is logical and based directly on observations. The hypothesis is also simple, involving just one type each of mold and bacteria growing on a plate. In addition, hypotheses are subject to "if/then" conditions. Thus, Fleming might have stated, "If a certain type of mold is introduced to a particular kind of bacteria growing on a plate, then the bacteria will die." This makes the hypothesis easy to test and ensures that it is falsifiable. If the bacteria were to grow in the presence of the mold, it would disprove the hypothesis (assuming the hypothesis is really false).
Hypothesis Testing
Hypothesis testing is at the heart of the scientific method. How would Fleming test his hypothesis? He would gather relevant data as evidence. Evidence is any type of data that may be used to test a hypothesis. Data (singular, datum) are essentially just observations. The observations may be measurements in an experiment or just something the researcher notices. Testing a hypothesis then involves using the data to answer two basic questions:
- If my hypothesis is true, what would I expect to observe?
- Does what I actually observe match what I expected to observe?
A hypothesis is supported if the actual observations (data) match the expected observations. A hypothesis is refuted if the actual observations differ from the expected observations.
The scientific method is employed by scientists around the world, but it is not always conducted in the order above. Sometimes, hypothesis are formulated before observations are collected; sometimes observations are made before hypothesis are created. Regardless, it is important that scientists record their procedures carefully, allowing others to reproduce and verify the experimental data and results. After many experiments provide results supporting a hypothesis, the hypothesis becomes a theory. Theories remain theories forever, and are constantly being retested with every experiment and observation. Theories can never become fact or law.
In science, a law is a mathematical relationship that exists between observations under a given set of conditions. There is a fundamental difference between observations of the physical world and explanations of the nature of the physical world. Hypotheses and theories are explanations, whereas laws and measurements are observational
1.4 Summary
- The scientific method consists of making observations, formulating a hypothesis, testing the hypothesis with new observations, making a new hypothesis if the new observations contradict the old hypothesis, or continuing to test the hypothesis if the observations agree.
- A hypothesis is a tentative explanation that can be tested by further observation.
- A theory is a hypothesis that has been supported with repeated testing.
- A scientific law is a statement that summarizes the results of many observations.
- Experimental data must be verified by reproduction from other scientists.
- Theories must agree with all observations made on the phenomenon under study.
- Theories are continually tested, forever.
1.4 Review Questions
1.4 Explore More
https://www.youtube.com/watch?v=F8UFGu2M2gM
How simple ideas lead to scientific discoveries, TED-Ed, 2012.
Attributions
Figure 1.4.1
The Scientific Method (simple), by Thebiologyprimer on Wikimedia Commons is used under a CC0 1.0 Universal Public Domain Dedication license (https://creativecommons.org/publicdomain/zero/1.0/deed.en).
Figure 1.4.2
Anatomy Bone Bones Check Doctor Examine Film, by rawpixel on Pixabay, used under the Pixabay License (https://pixabay.com/de/service/license/).
Figure 1.4.3
Penicillin Past, Present and Future- the Development and Production of Penicillin, England, 1944, by Ministry of Information Photo Division Photographer. This photograph was scanned and released by the Imperial War Museum on the IWM Non Commercial Licence. It is now in the public domain (https://en.wikipedia.org/wiki/Public_domain).
References
TED-Ed. (2012, Mar 13). How simple ideas lead to scientific discoveries. YouTube. https://www.youtube.com/watch?v=F8UFGu2M2gM
Wikipedia contributors. (2020, July 7). Alexander Fleming. In Wikipedia. https://en.wikipedia.org/w/index.php?title=Alexander_Fleming&oldid=966489433