4.8 Active Transport
Created by: CK-12/Adapted by Christine Miller
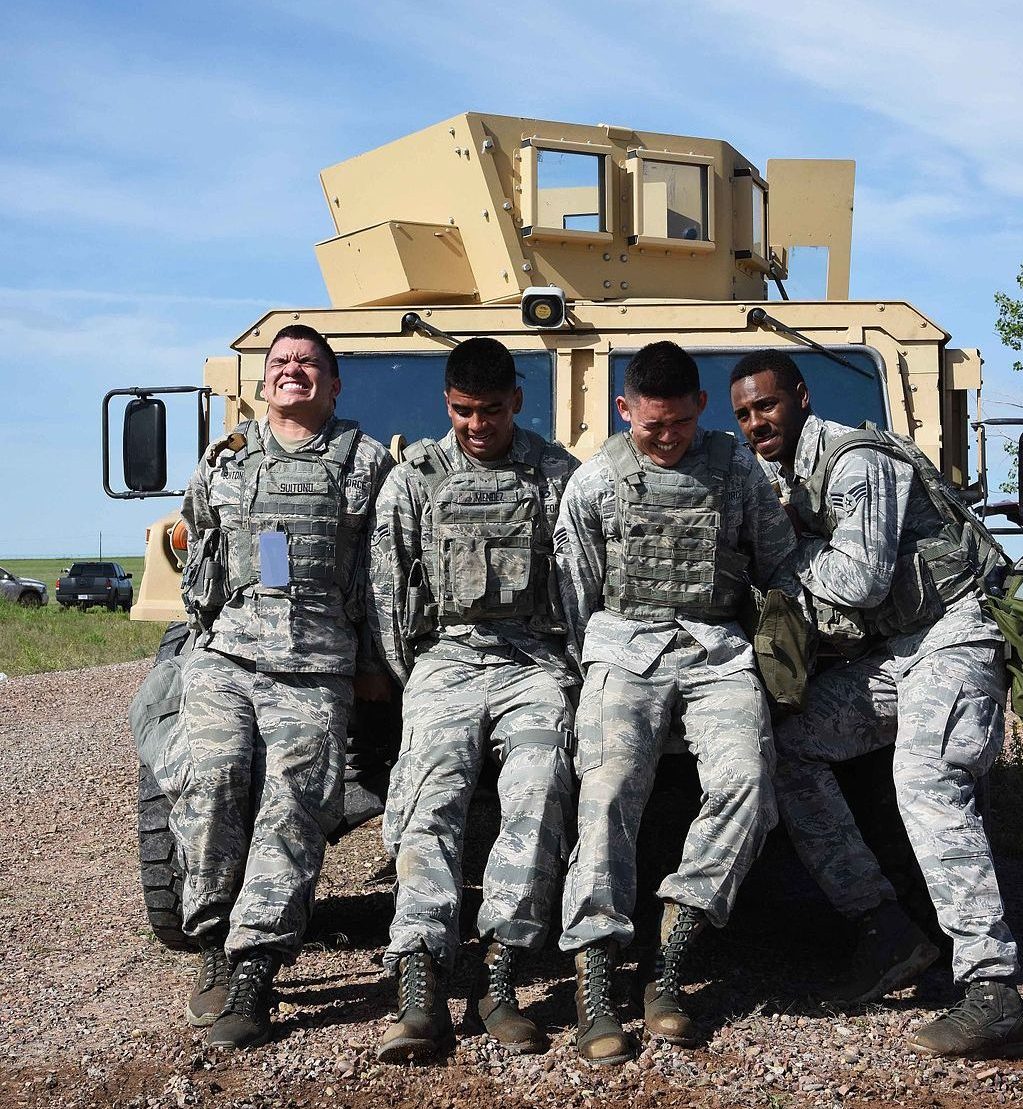
Like Pushing a Humvee Uphill
You can tell by their faces that these airmen (Figure 4.8.1) are expending a lot of energy trying to push this Humvee up a slope. The men are participating in a competition that tests their brute strength against that of other teams. The Humvee weighs about 13 thousand pounds (about 5,897 kilograms), so it takes every ounce of energy they can muster to move it uphill against the force of gravity. Transport of some substances across a plasma membrane is a little like pushing a Humvee uphill — it can’t be done without adding energy.
What Is Active Transport?
Some substances can pass into or out of a cell across the plasma membrane without any energy required because they are moving from an area of higher concentration to an area of lower concentration. This type of transport is called passive transport. Other substances require energy to cross a plasma membrane, often because they are moving from an area of lower concentration to an area of higher concentration, against the concentration gradient. This type of transport is called active transport. The energy for active transport comes from the energy-carrying molecule called ATP (adenosine triphosphate). Active transport may also require proteins called pumps, which are embedded in the plasma membrane. Two types of active transport are membrane pumps (such as the sodium-potassium pump) and vesicle transport.
The Sodium-Potassium Pump
The sodium-potassium pump is a mechanism of active transport that moves sodium ions out of the cell and potassium ions into the cells — in all the trillions of cells in the body! Both ions are moved from areas of lower to higher concentration, so energy is needed for this “uphill” process. The energy is provided by ATP. The sodium-potassium pump also requires carrier proteins. Carrier proteins bind with specific ions or molecules, and in doing so, they change shape. As carrier proteins change shape, they carry the ions or molecules across the membrane. Figure 4.8.2 shows in greater detail how the sodium-potassium pump works, as well as the specific roles played by carrier proteins in this process.
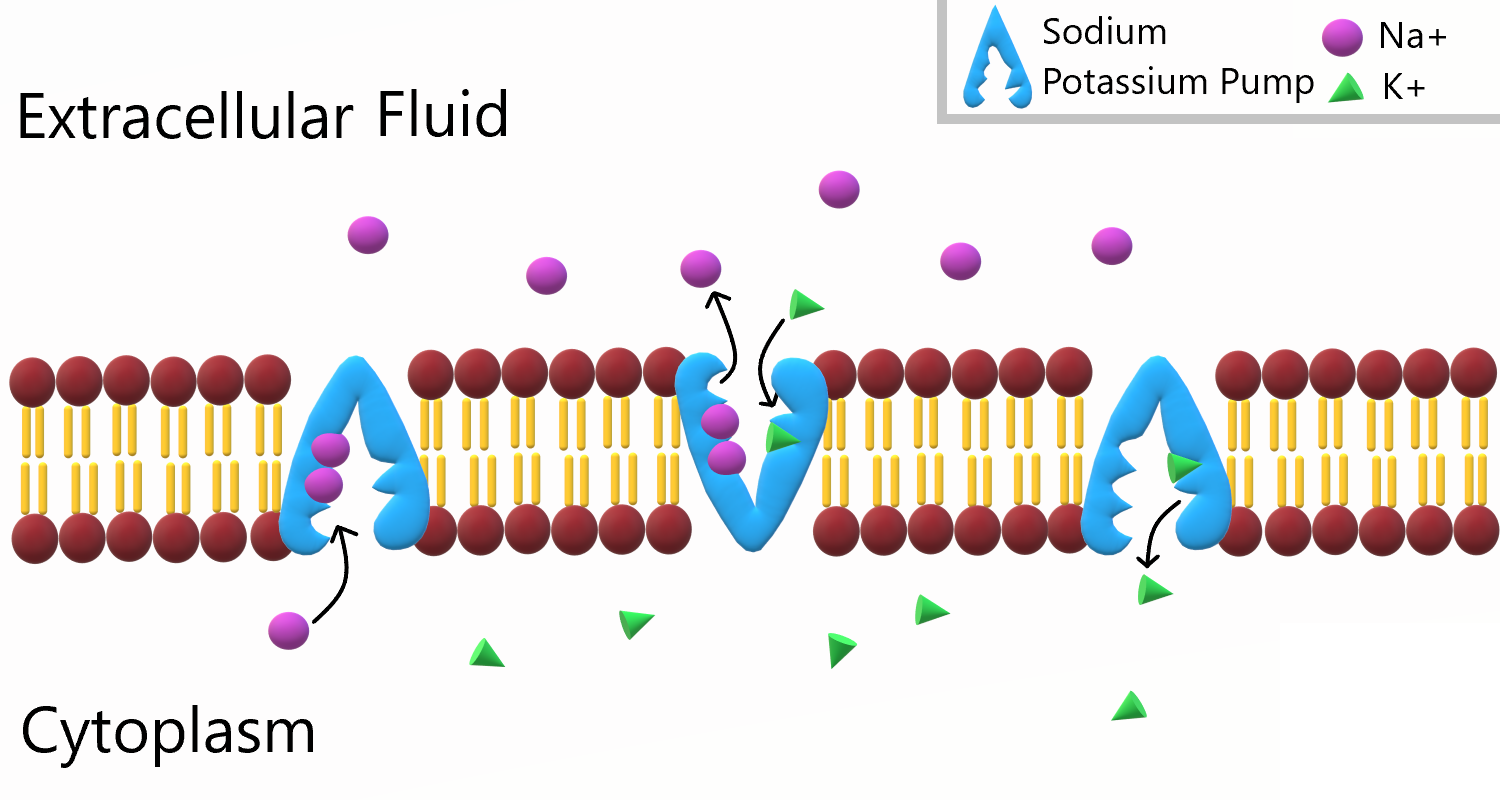
To appreciate the importance of the sodium-potassium pump, you need to know more about the roles of sodium and potassium in the body. Both are essential dietary minerals. You need to get them from the foods you eat. Both sodium and potassium are also electrolytes, which means they dissociate into ions (charged particles) in solution, allowing them to conduct electricity. Normal body functions require a very narrow range of concentrations of sodium and potassium ions in body fluids, both inside and outside of cells.
- Sodium is the principal ion in the fluid outside of cells. Normal sodium concentrations are about ten times higher outside of cells than inside of cells. To move sodium out of the cell is moving it against the concentration gradient
- Potassium is the principal ion in the fluid inside of cells. Normal potassium concentrations are about 30 times higher inside of cells than outside of cells. To move potassium into the cell is moving it against the concentration gradient.
These differences in concentration create an electrical and chemical gradient across the cell membrane, called the membrane potential. Tightly controlling the membrane potential is critical for vital body functions, including the transmission of nerve impulses and contraction of muscles. A large percentage of the body’s energy goes to maintaining this potential across the membranes of its trillions of cells with the sodium-potassium pump.
Vesicle Transport
Some molecules, such as proteins, are too large to pass through the plasma membrane, regardless of their concentration inside and outside the cell. Very large molecules cross the plasma membrane with a different sort of help, called vesicle transport. Vesicle transport requires energy input from the cell, so it is also a form of active transport. There are two types of vesicle transport: endocytosis and exocytosis. Both types are shown in Figure 4.8.3.
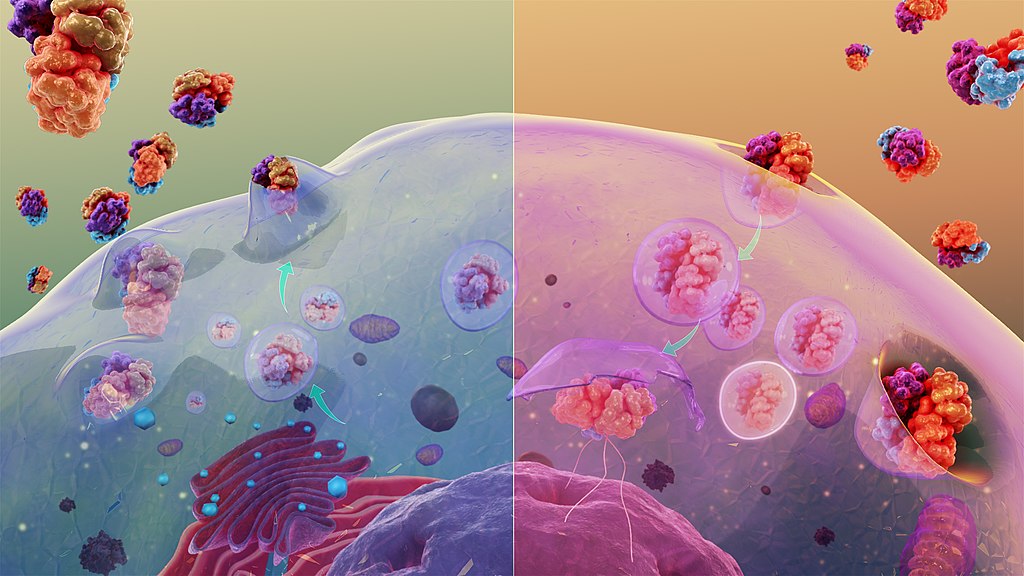
Endocytosis
Endocytosis is a type of vesicle transport that moves a substance into the cell. The plasma membrane completely engulfs the substance, a vesicle pinches off from the membrane, and the vesicle carries the substance into the cell. When an entire cell or other solid particle is engulfed, the process is called phagocytosis. When fluid is engulfed, the process is called pinocytosis.
Exocytosis
Exocytosis is a type of vesicle transport that moves a substance out of the cell (exo-, like “exit”). A vesicle containing the substance moves through the cytoplasm to the cell membrane. Because the vesicle membrane is a phospholipid bilayer like the plasma membrane, the vesicle membrane fuses with the cell membrane, and the substance is released outside the cell.
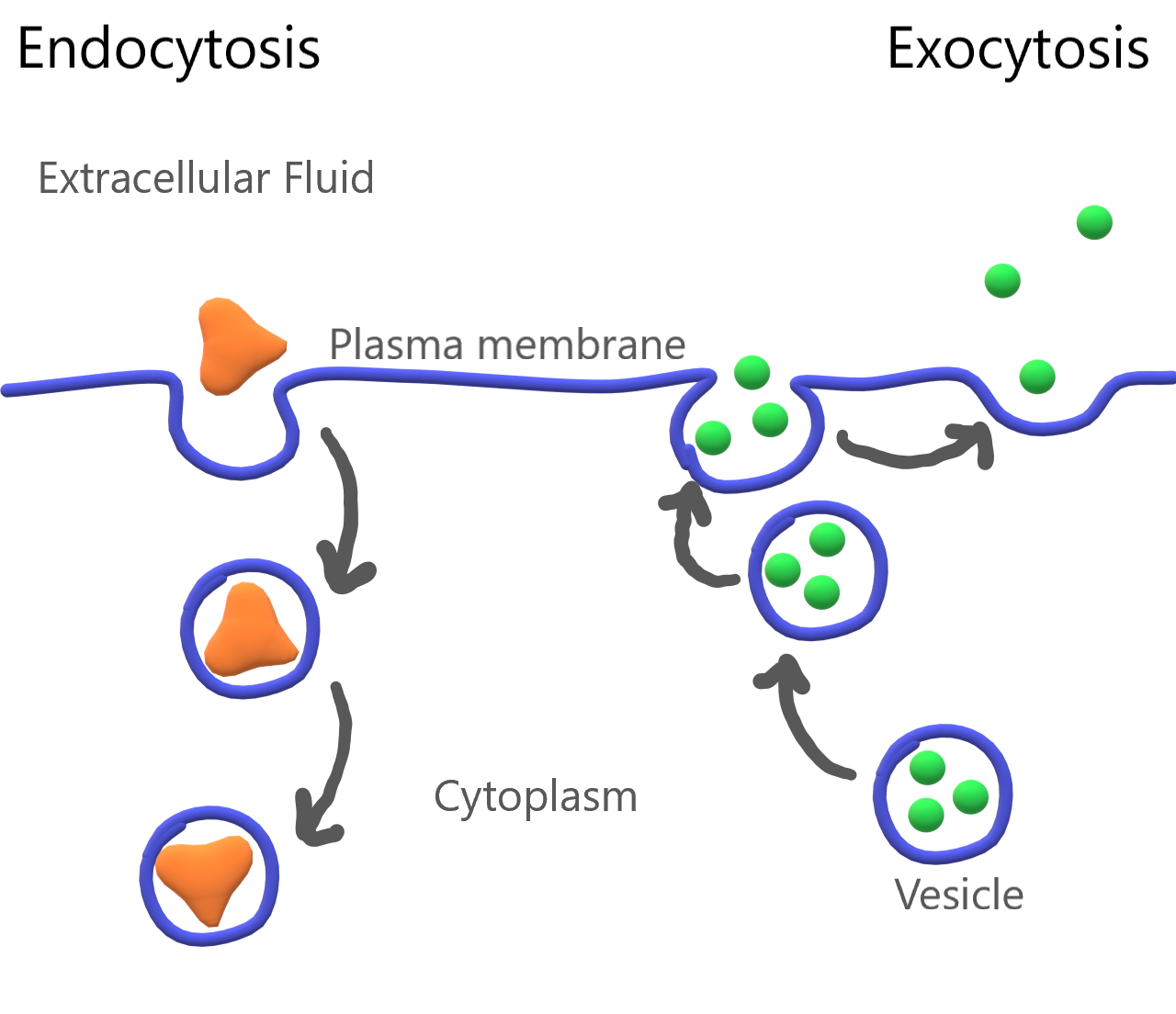
Feature: My Human Body
Maintaining the proper balance of sodium and potassium in body fluids by active transport is necessary for life itself, so it’s no surprise that getting the right balance of sodium and potassium in the diet is important for good health. Imbalances may increase the risk of high blood pressure, heart disease, diabetes, and other disorders.
If you are like the majority of North Americans, sodium and potassium are out of balance in your diet. You are likely to consume too much sodium and too little potassium. Follow these guidelines to help ensure that these minerals are balanced in the foods you eat:
- Total sodium intake should be less than 2,300 mg/day. Most salt in the diet is found in processed foods, or added with a salt shaker. Stop adding salt and start checking food labels for sodium content. Foods considered low in sodium have less than 140 mg/serving (or 5 per cent daily value).
- Total potassium intake should be 4,700 mg/day. It’s easy to add potassium to the diet by choosing the right foods — and there are plenty of choices! Most fruits and vegetables are high in potassium. Potatoes, bananas, oranges, apricots, plums, leafy greens, tomatoes, lima beans, and avocado are especially good sources. Other foods with substantial amounts of potassium are fish, meat, poultry, and whole grains. The collage below shows some of these potassium-rich foods.
Figure 4.8.5 Potassium power!
4.8 Summary
- Active transport requires energy to move substances across a plasma membrane, often because the substances are moving from an area of lower concentration to an area of higher concentration, or because of their large size. Two types of active transport are membrane pumps (such as the sodium-potassium pump) and vesicle transport.
- The sodium-potassium pump is a mechanism of active transport that moves sodium ions out of the cell and potassium ions into the cell against a concentration gradient, in order to maintain the proper concentrations of ions, both inside and outside the cell, and to thereby control membrane potential.
- Vesicle transport is a type of active transport that uses vesicles to move large molecules into or out of cells.
4.8 Review Questions
- Define active transport.
-
- What is the sodium-potassium pump? Why is it so important?
- The drawing below shows the fluid inside and outside of a cell. The dots represent molecules of a substance needed by the cell. Explain which type of transport — active or passive — is needed to move the molecules into the cell.
Figure 4.8.6 Use this image to answer question #4 - What are the similarities and differences between phagocytosis and pinocytosis?
- What is the functional significance of the shape change of the carrier protein in the sodium-potassium pump after the sodium ions bind?
- A potentially deadly poison derived from plants called ouabain blocks the sodium-potassium pump and prevents it from working. What do you think this does to the sodium and potassium balance in cells? Explain your answer.
4.8 Explore More
Neutrophil Phagocytosis – White Blood Cell Eats Staphylococcus Aureus Bacteria,
ImmiflexImmuneSystem, 2013.
Cell Transport, The Amoeba Sisters, 2016.
Attributions
Figure 4.8.1
Humvee challenge by Airman 1st Class Collin Schmidt on Wikimedia Commons is released into the public domain (https://en.wikipedia.org/wiki/Public_domain).
Figure 4.8.2
Sodium Potassium Pump by Christine Miller is used under a CC BY 4.0 (https://creativecommons.org/licenses/by/4.0/) license.
Figure 4.8.3
Cytosis by Manu5 on Wikimedia Commons is used under a CC BY-SA 4.0 (https://creativecommons.org/licenses/by-sa/4.0) license.
Figure 4.8.4
Endocytosis and Exocytosis by Christine Miller is used under a CC BY 4.0 (https://creativecommons.org/licenses/by/4.0/) license.
Figure 4.8.5
- Canteloupes. Image Number K7355-11 by Scott Bauer/ USDA on Wikimedia Commons is in the public domain (https://en.wikipedia.org/wiki/Public_domain).
- Spinach by chiara conti on Unsplash is used under the Unsplash license (https://unsplash.com/license).
- Eleven long purple eggplants by JVRKPRASAD on Wikimedia commons is used under a CC BY-SA 4.0 (https://creativecommons.org/licenses/by-sa/4.0/deed.en) license.
- Bananas by Marco Antonio Victorino on Pexels is used under the Pexels license (https://www.pexels.com/license/).
- Potato picking by Nic D on Unsplash is used under the Unsplash license (https://unsplash.com/license).
- Maldives by Sebastian Pena Lambarri on Unsplash is used under the Unsplash license (https://unsplash.com/license).
Figure 4.8.6
Active Transport by Christine Miller is released into the public domain (https://en.wikipedia.org/wiki/Public_domain).
References
Amoeba Sisters. (2016, June 24). Cell transport [digital image]. YouTube. https://www.youtube.com/watch?v=Ptmlvtei8hw&feature=youtu.be
ImmiflexImmuneSystem. (2013). Neutrophil phagocytosis – White blood cell eats staphylococcus aureus bacteria. YouTube. https://www.youtube.com/watch?v=Z_mXDvZQ6dU
Mayo Clinic Staff. (n.d.). Diabetes [online]. MayoClinic.org. https://www.mayoclinic.org/diseases-conditions/diabetes/symptoms-causes/syc-20371444
Mayo Clinic Staff. (n.d.). High blood pressure (hypertension) [online]. MayoClinic.org. https://www.mayoclinic.org/diseases-conditions/high-blood-pressure/symptoms-causes/syc-20373410
Mayo Clinic Staff. (n.d.). Heart disease [online]. MayoClinic.org. https://www.mayoclinic.org/diseases-conditions/heart-disease/symptoms-causes/syc-20353118
Wikipedia contributors. (2020, June 19). Ouabain. In Wikipedia. https://en.wikipedia.org/w/index.php?title=Ouabain&oldid=963440756
Diagram shows examples of the shapes of different types of fatty acids. Saturated fatty acids form long straight chains. Monounsaturated fatty acids have a slight curve and saturated fatty acids can have multiple curves or bends.
List of types of mutagens. Radiation includes UV radiation and X rays. Chemicals include cigarette smoke a vaping vapor, nitrite and nitrate preservatives, barbecuing, and benzoyl peroxide. Infectious agents include HPV and H. Pylori.
Created by: CK-12/Adapted by Christine Miller
Of Peas and People
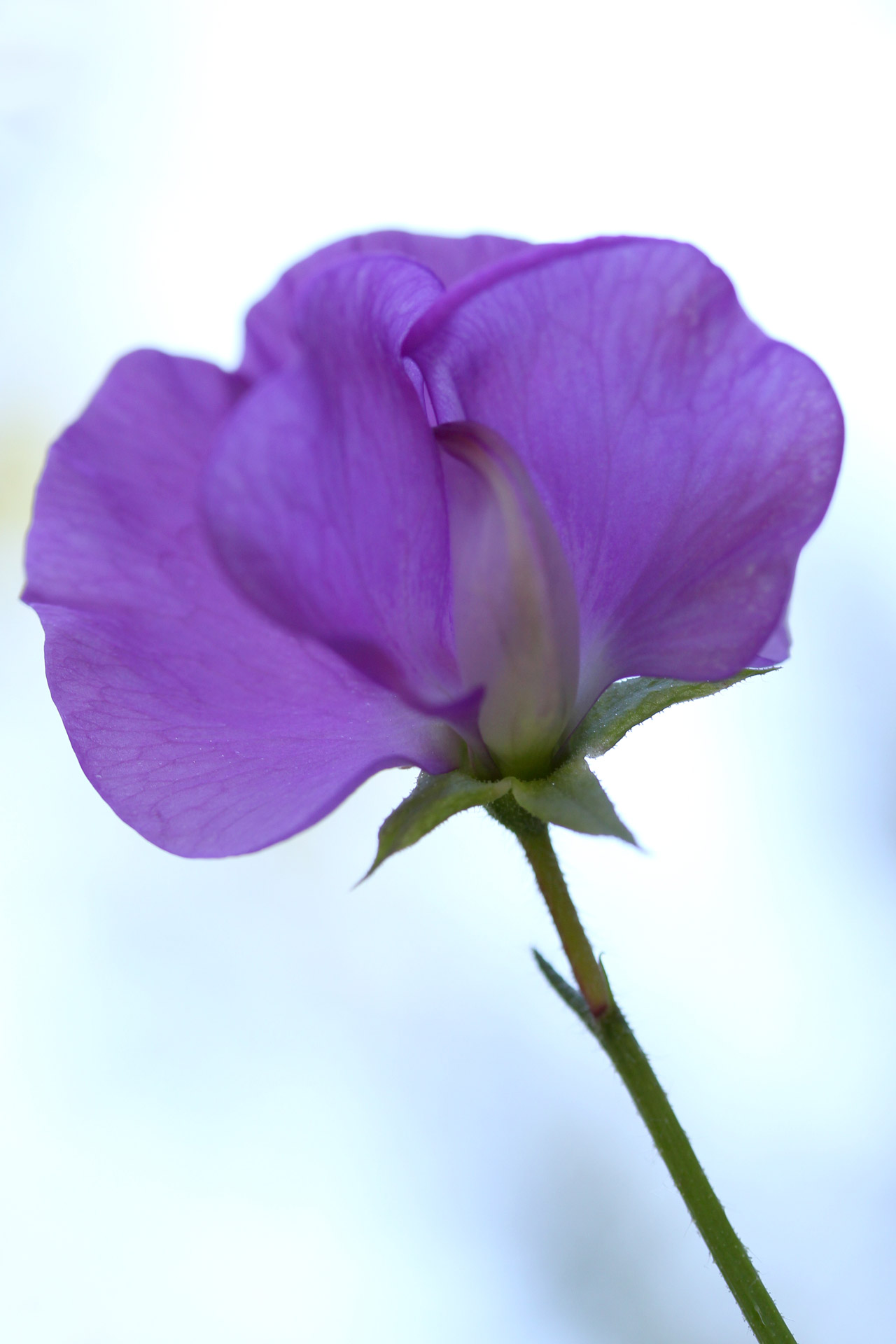
These purple-flowered plants are not just pretty to look at. Plants like these led to a huge leap forward in biology. They're common garden peas, and they were studied in the mid-1800s by an Austrian monk named Gregor Mendel. Through careful experimentation, Mendel uncovered the secrets of heredity, or how parents pass characteristics to their offspring. You may not care much about heredity in pea plants, but you probably care about your own heredity. Mendel's discoveries apply to people, as well as to peas — and to all other living things that reproduce sexually. In this concept, you will read about Mendel's experiments and the secrets of heredity that he discovered.
Mendel and His Pea Plants
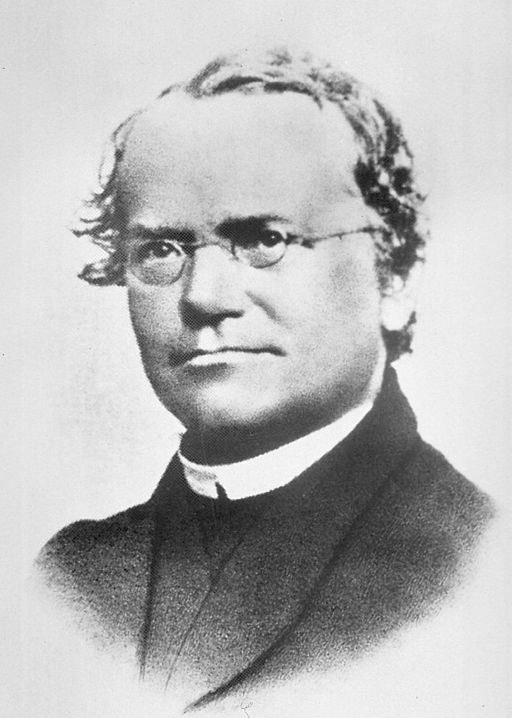
Gregor Mendel (Figure 5.10.2) was born in 1822. He grew up on his parents’ farm in Austria. He did well in school and became a friar (and later an abbot) at St. Thomas' Abbey. Through sponsorship from the monastery, he went on to the University of Vienna, where he studied science and math. His professors encouraged him to learn science through experimentation, and to use math to make sense of his results. Mendel is best known for his experiments with pea plants (like the purple flower pictured in Figure 5.10.1).
Blending Theory of Inheritance

During Mendel's time, the blending theory of inheritance was popular. According to this theory, offspring have a blend (or mix) of their parents' characteristics. Mendel, however, noticed plants in his own garden that weren’t a blend of the parents. For example, a tall plant and a short plant had offspring that were either tall or short — not medium in height. Observations such as these led Mendel to question the blending theory. He wondered if there was a different underlying principle that could explain how characteristics are inherited. He decided to experiment with pea plants to find out. In fact, Mendel experimented with almost 30 thousand pea plants over the next several years!
Why Study Pea Plants?
Why did Mendel choose common, garden-variety pea plants for his experiments? Pea plants are a good choice because they are fast-growing and easy to raise. They also have several visible characteristics that can vary. These characteristics — some of which are illustrated in Figure 5.10.4 — include seed form and colour, flower colour, pod form and colour, placement of pods and flowers on stems, and stem length. Each of these characteristics has two common values. For example, seed form may be round or wrinkled, and flower colour may be white or purple (violet).
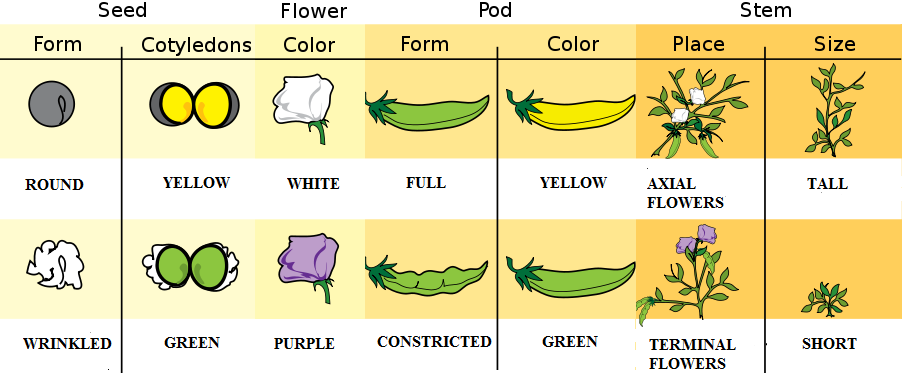
Controlling Pollination
To research how characteristics are passed from parents to offspring, Mendel needed to control pollination, which is the fertilization step in the sexual reproduction of plants. Pollen consists of tiny grains that are the male sex cells (or gametes) of plants. They are produced by a male flower part called the anther. Pollination occurs when pollen is transferred from the anther to the stigma of the same or another flower. The stigma is a female part of a flower, and it passes pollen grains to female gametes in the ovary.
Pea plants are naturally self-pollinating. In self-pollination, pollen grains from anthers on one plant are transferred to stigmas of flowers on the same plant. Mendel was interested in the offspring of two different parent plants, so he had to prevent self-pollination. He removed the anthers from the flowers of some of the plants in his experiments. Then he pollinated them by hand using a small paintbrush with pollen from other parent plants of his choice.
When pollen from one plant fertilizes another plant of the same species, it is called cross-pollination. The offspring that result from such a cross are called hybrids. When the term hybrid is used in this context, it refers to any offspring resulting from the breeding of two genetically distinct individuals.
Mendel's First Set of Experiments
At first, Mendel experimented with just one characteristic at a time. He began with flower colour. As shown in Figure 5.10.5, Mendel cross-pollinated purple- and white-flowered parent plants. The parent plants in the experiments are referred to as the P (for parent) generation.
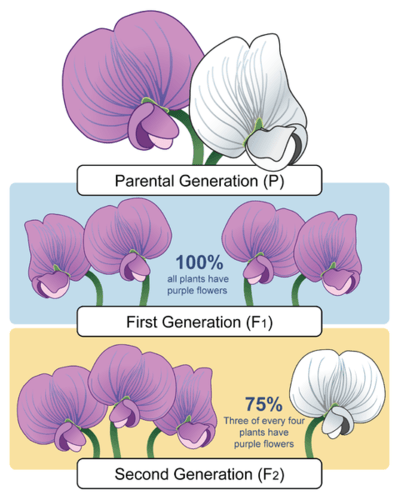
Figure 5.10.5 shows Mendel's first experiment with pea plants. The F1 generation results from the cross-pollination of two parent (P) plants, and it contains all purple flowers. The F2 generation results from the self-pollination of F1 plants, and contains 75% purple flowers and 25% white flowers.
F1 and F2 Generations
The offspring of the P generation are called the F1 (for filial, or “offspring”) generation. As shown in Figure 5.10.5, all of the plants in the F1 generation had purple flowers — none of them had white flowers. Mendel wondered what had happened to the white-flower characteristic. He assumed that some type of inherited factor produces white flowers and some other inherited factor produces purple flowers. Did the white-flower factor just disappear in the F1 generation? If so, then the offspring of the F1 generation — called the F2 generation — should all have purple flowers like their parents.
To test this prediction, Mendel allowed the F1 generation plants to self-pollinate. He was surprised by the results. Some of the F2 generation plants had white flowers. He studied hundreds of F2 generation plants, and for every three purple-flowered plants, there was an average of one white-flowered plant.
Law of Segregation
Mendel did the same experiment for all seven characteristics. In each case, one value of the characteristic disappeared in the F1 plants, later showing up again in the F2 plants. In each case, 75 per cent of F2 plants had one value of the characteristic, while 25 per cent had the other value. Based on these observations, Mendel formulated his first law of inheritance. This law is called the law of segregation. It states that there are two factors controlling a given characteristic, one of which dominates the other, and these factors separate and go to different gametes when a parent reproduces.
Mendel's Second Set of Experiments
Mendel wondered whether different characteristics are inherited together. For example, are purple flowers and tall stems always inherited together, or do these two characteristics show up in different combinations in offspring? To answer these questions, Mendel next investigated two characteristics at a time. For example, he crossed plants with yellow round seeds and plants with green wrinkled seeds. The results of this cross are shown in Figure 5.10.6.
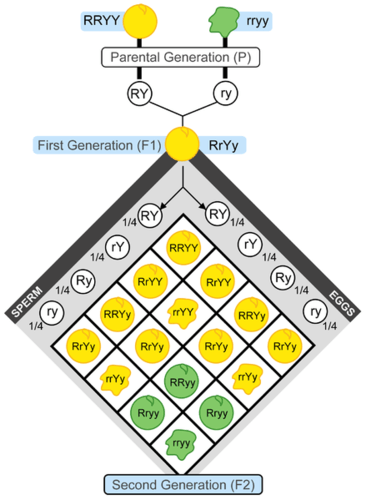
Figure 5.10.6 shows the outcome of a cross between plants that differ in seed colour (yellow or green) and seed form (shown here with a smooth round appearance or wrinkled appearance). The letters R, r, Y, and y represent genes for the characteristics Mendel was studying. Mendel didn’t know about genes, however, because genes would not be discovered until several decades later. This experiment demonstrates that, in the F2 generation, nine out of 16 were round yellow seeds, three out of 16 were wrinkled yellow seeds, three out of 16 were round green seeds, and one out of 16 was wrinkled green seeds.
F1 and F2 Generations
In this set of experiments, Mendel observed that plants in the F1 generation were all alike. All of them had yellow round seeds like one of the two parents. When the F1 generation plants self-pollinated, however, their offspring — the F2 generation — showed all possible combinations of the two characteristics. Some had green round seeds, for example, and some had yellow wrinkled seeds. These combinations of characteristics were not present in the F1 or P generations.
Law of Independent Assortment
Mendel repeated this experiment with other combinations of characteristics, such as flower colour and stem length. Each time, the results were the same as those shown in Figure 5.10.6. The results of Mendel's second set of experiments led to his second law. This is the law of independent assortment. It states that factors controlling different characteristics are inherited independently of each other.
Mendel's Legacy
You might think that Mendel's discoveries would have made a big impact on science as soon as he made them, but you would be wrong. Why? Because Mendel's work was largely ignored. Mendel was far ahead of his time, and he was working from a remote monastery. He had no reputation in the scientific community and had only published sparingly in the past. Additionally, he published this research in an obscure scientific journal. As a result, when Charles Darwin published his landmark book on evolution in 1869, although Mendel's work had been published just a few years earlier, Darwin was unaware of it. Consequently, Darwin knew nothing about Mendel's laws, and didn’t understand heredity. This made Darwin's arguments about evolution less convincing to many.
Then, in 1900, three different European scientists — Hugo de DeVries, Carl Correns, and Erich von Tschermak — arrived independently at Mendel's laws. All three had done experiments similar to Mendel's and come to the same conclusions that he had drawn several decades earlier. Only then was Mendel's work rediscovered, so that Mendel himself could be given the credit he was due. Although Mendel knew nothing about genes, which were discovered after his death, he is now considered the father of genetics.
5.10 Cultural Connection
Corn is the world's most produced crop. Canada produces 13,000-14,000 metric Kilo tonnes of corn annually, mostly in fields in Ontario, Quebec and Manitoba. Approximately 1.5 million hectares are devoted to this crop which is critically important for both humans and livestock as a food source. Despite these high numbers of output, Canada is still only 11th on the list of world corn producers, with USA, China and Brazil claiming the top three places. How did corn become such an important part of modern agriculture?
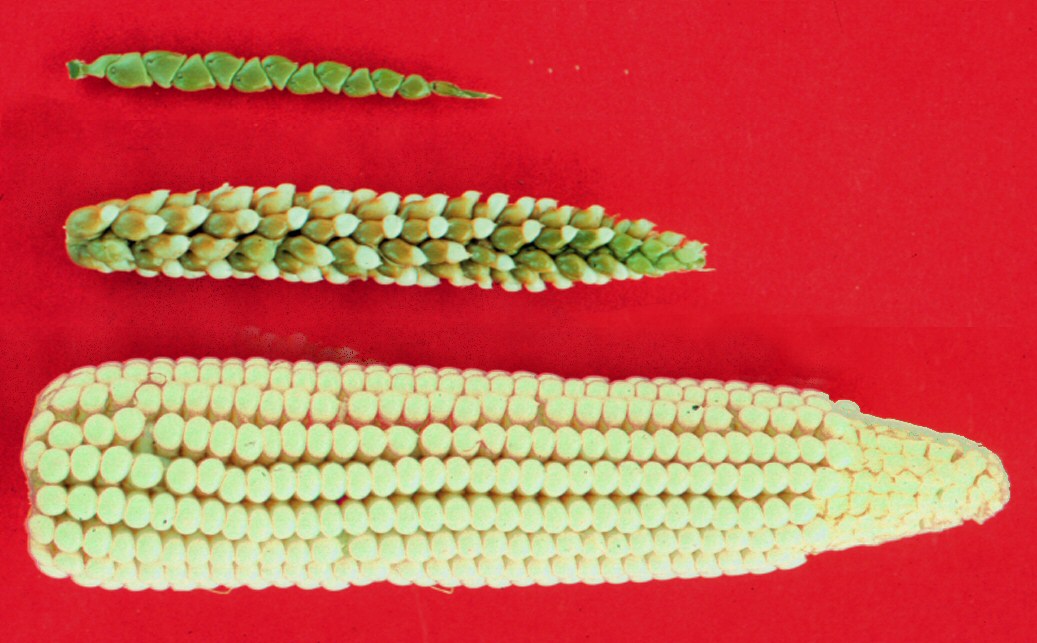
We didn't always have corn as we know it. Modern corn is descended from a type of grass called teosinte (Figure 5.10.7) native to Mesoamerica (southern part of North America). It is estimated that Indigenous people have been harvesting corn and corn ancestors for over 9000 years. Excavations of the Xihuatoxtla Shelter in southwestern Mexico revealed our earliest evidence of domesticated corn: maize remains on tools dating back 8,700 years.
Ancient Indigenous peoples of southern Mexico developed corn from grass plants using a process we now call selective breeding, also known as artificial selection. Teosinte doesn't resemble the corn we have today- it had only a few kernels individually encased on very hard shells, and yet today we have multiple varieties of corn with row upon row of bare kernels. This means that ancient agriculturalists among the Indigenous people of Mexico were intentionally cross-breeding strains of teosinte, and later, early maize to create plants which had more kernels, and reduced seed casings. Watch the TED Ed video in the Explore More section to see what other changes agriculturalists have made to modern-day corn.
5.10 Summary
- Mendel experimented with the inheritance of traits in pea plants at a time when the blending theory of inheritance was popular. This is the theory that offspring have a blend of the characteristics of their parents.
- Pea plants were good choices for this research, largely because they have several visible characteristics that exist in two different forms. By controlling pollination, Mendel was able to cross pea plants with different forms of the traits.
- In Mendel's first set of experiments, he experimented with just one characteristic at a time. The results of this set of experiments led to Mendel's first law of inheritance, called the law of segregation. This law states that there are two factors controlling a given characteristic, one of which dominates the other, and these factors separate and go to different gametes when a parent reproduces.
- In Mendel's second set of experiments, he experimented with two characteristics at a time. The results of this set of experiments led to Mendel's second law of inheritance, called the law of independent assortment. This law states that the factors controlling different characteristics are inherited independently of each other.
- Mendel's work was largely ignored during his own lifetime. However, when other researchers arrived at the same laws in 1900, Mendel's work was rediscovered, and he was given the credit he was due. He is now considered the father of genetics.
5.10 Review Questions
- Why were pea plants a good choice for Mendel's experiments?
-
- How did the outcome of Mendel's second set of experiments lead to his second law?
- Discuss the development of Mendel's legacy.
- If Mendel’s law of independent assortment was not correct, and characteristics were always inherited together, what types of offspring do you think would have been produced by crossing plants with yellow round seeds and green wrinkled seeds? Explain your answer.
5.10 Explore More
https://www.youtube.com/watch?v=Mehz7tCxjSE&feature=emb_logo
How Mendel's pea plants helped us understand genetics - Hortensia Jiménez Díaz, TED-Ed, 2013.
https://www.youtube.com/watch?v=ogc367xyzfk&feature=emb_logo
10 Strange Hybrid Fruits, Junkyboss, 2016.
https://www.youtube.com/watch?v=i6teBcfKpik
The history of the world according to corn - Chris A. Kniesly, TED-Ed, 2019.
Attributions
Figure 5.10.1
Purple sweet pea flower by unknown on Yana Ray on publicdomainpictures.net is used under a CC0 1.0 public domain dedication license (https://creativecommons.org/publicdomain/zero/1.0/deed.en).
Figure 5.10.2
Gregor_Mendel by unknown from National Institutes of Health, Health & Human Services on Wikimedia Commons is in the public domain (https://en.wikipedia.org/wiki/Public_domain).
Figure 5.10.3
Gregor Mendel in Lego by Alan on Flickr is used under a CC BY-NC-SA 2.0 (https://creativecommons.org/licenses/by-nc-sa/2.0/) license.
Figure 5.10.4
Mendels_peas by Mariana Ruiz [LadyofHats] on Wikimedia Commons is used under a CC0 1.0 public domain dedication license (https://creativecommons.org/publicdomain/zero/1.0/deed.en).
Figure 5.10.5
Mendel's first experiment with pea plants by CK-12 Foundation is used under a CC BY-NC 3.0 (https://creativecommons.org/licenses/by-nc/3.0/) license.
©CK-12 Foundation Licensed under
• Terms of Use • Attribution
Figure 5.10.6
Mendel's Second Experiment by by CK-12 Foundation is used under a CC BY-NC 3.0 (https://creativecommons.org/licenses/by-nc/3.0/) license.
©CK-12 Foundation Licensed under
• Terms of Use • Attribution
Figure 5.10.7
Maize-teosinte by John Doebley on Wikimedia Commons is used under a CC BY 3.0 (https://creativecommons.org/licenses/by/3.0/deed.en) license.
References
Brainard, J/ CK-12 Foundation. (2016). Figure 5 Mendel's first experiment [digital image]. In CK-12 College Human Biology (Section 5.9) [online Flexbook]. CK12.org. https://www.ck12.org/book/ck-12-human-biology/section/5.9/
Brainard, J/ CK-12 Foundation. (2016). Figure 6 Mendel's second experiment [digital image]. In CK-12 College Human Biology (Section 5.9) [online Flexbook]. CK12.org. https://www.ck12.org/book/ck-12-human-biology/section/5.9/
Junkyboss. (2016, March 31). 10 Strange hybrid fruits. YouTube. https://www.youtube.com/watch?v=ogc367xyzfk&feature=youtu.be
TED-Ed. (2013, March 12). How Mendel's pea plants helped us understand genetics - Hortensia Jiménez Díaz. YouTube. https://www.youtube.com/watch?v=Mehz7tCxjSE&feature=youtu.be
TED-Ed. (2019, November 26). The history of the world according to corn - Chris A. Kniesly. YouTube. https://www.youtube.com/watch?v=i6teBcfKpik&feature=youtu.be
Wikipedia contributors. (2020, June 1). Carl Correns. In Wikipedia. https://en.wikipedia.org/w/index.php?title=Carl_Correns&oldid=960172546
Wikipedia contributors. (2020, July 8). Charles Darwin. In Wikipedia. https://en.wikipedia.org/w/index.php?title=Charles_Darwin&oldid=966652322
Wikipedia contributors. (2020, March 9). Erich von Tschermak. In Wikipedia. https://en.wikipedia.org/w/index.php?title=Erich_von_Tschermak&oldid=944695823
Wikipedia contributors. (2020, July 7). Hugo de Vries. In Wikipedia. https://en.wikipedia.org/w/index.php?title=Hugo_de_Vries&oldid=966513671
Created by: CK-12/Adapted by Christine Miller
Divide and Split
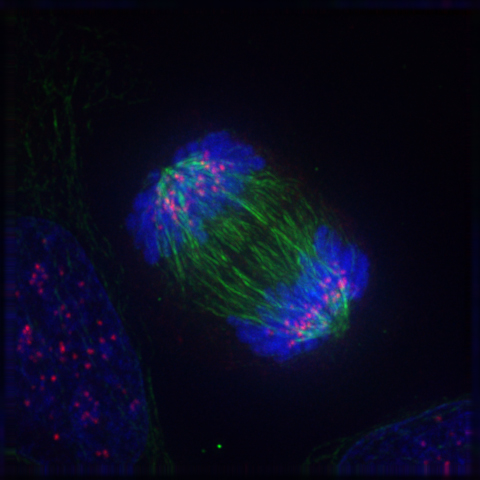
Can you guess what the colourful image in Figure 4.13.1 represents? It shows a eukaryotic cell during the process of cell division. In particular, the image shows the cell in a part of cell division called anaphase, where the DNA is being pulled to opposite ends of the cell. Normally, DNA is located in the nucleus of most human cells. The nucleus divides before the cell itself splits in two, and before the nucleus divides, the cell’s DNA is replicated (or copied). There must be two copies of the DNA so that each daughter cell will have a complete copy of the genetic material from the parent cell. How is the replicated DNA sorted and separated so that each daughter cell gets a complete set of the genetic material? To answer that question, you first need to know more about DNA and the forms it takes.
The Forms of DNA
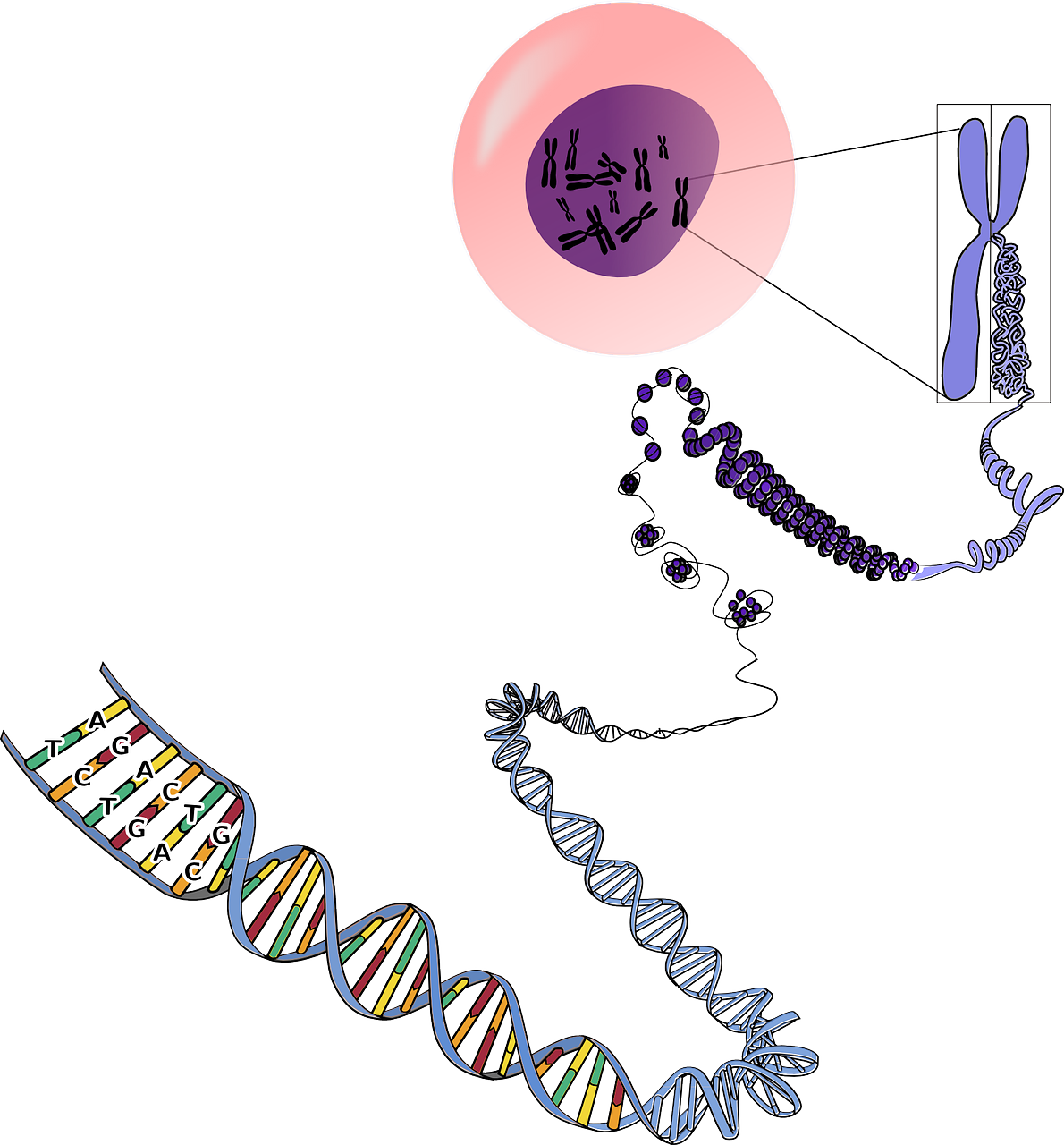
Except when a eukaryotic cell divides, its nuclear DNA exists as a grainy material called chromatin. Only once a cell is about to divide and its DNA has replicated does DNA condense and coil into the familiar X-shaped form of a chromosome, like the one shown below.
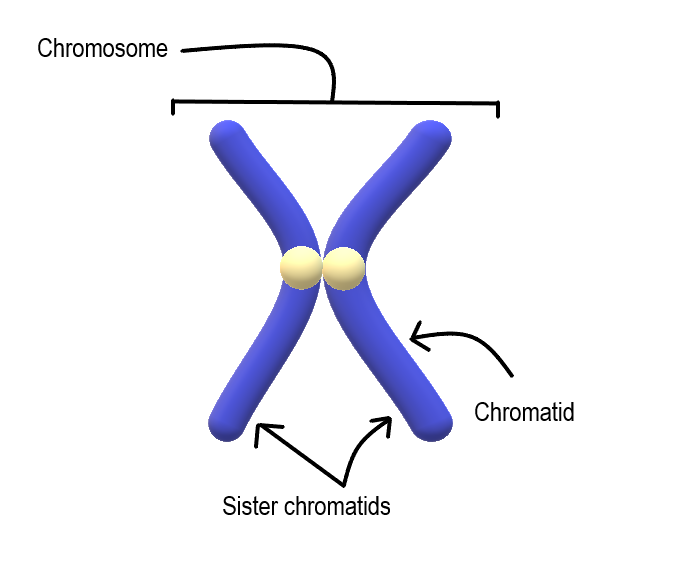
Most cells in the human body have two pairs of 23 different chromosomes, for a total of 46 chromosomes. Cells that have two pairs of chromosomes are called diploid. Because DNA has already replicated when it coils into a chromosome, each chromosome actually consists of two identical structures called sister chromatids. Sister chromatids are joined together at a region called a centromere.
Mitosis
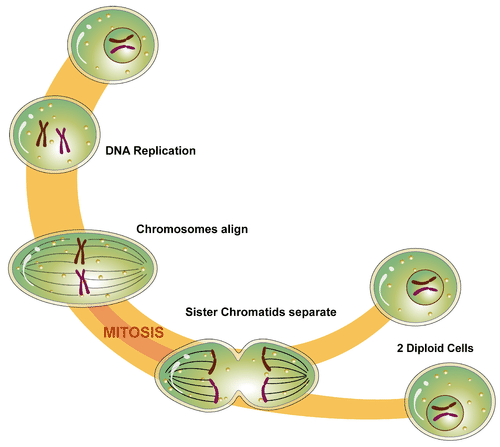
The process in which the nucleus of a eukaryotic cell divides is called mitosis. During mitosis, the two sister chromatids that make up each chromosome separate from each other and move to opposite poles of the cell. This is shown in the figure below.
Mitosis actually occurs in four phases. The phases are called prophase, metaphase, anaphase, and telophase.
Prophase

The first and longest phase of mitosis is prophase. During prophase, chromatin condenses into chromosomes, and the nuclear envelope (the membrane surrounding the nucleus) breaks down. In animal cells, the centrioles near the nucleus begin to separate and move to opposite poles of the cell. Centrioles are small organelles found only in eukaryotic cells. They help ensure that the new cells that form after cell division each contain a complete set of chromosomes. As the centrioles move apart, a spindle starts to form between them. The spindle consists of fibres made of microtubules.
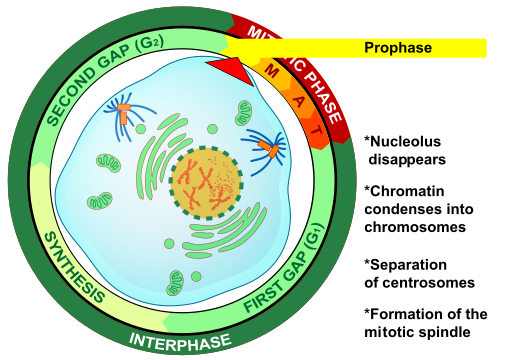
Metaphase
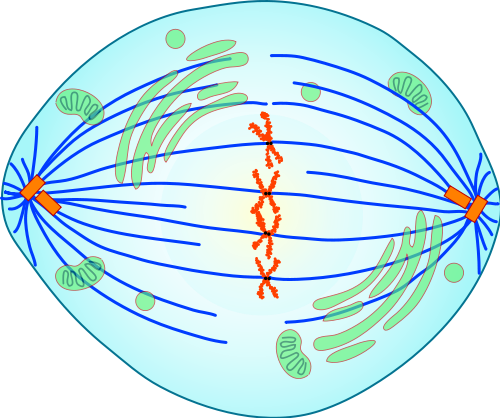
During metaphase, spindle fibres attach to the centromere of each pair of sister chromatids. As you can see in Figure 4.13.7, the sister chromatids line up at the equator (or center) of the cell. The spindle fibres ensure that sister chromatids will separate and go to different daughter cells when the cell divides.
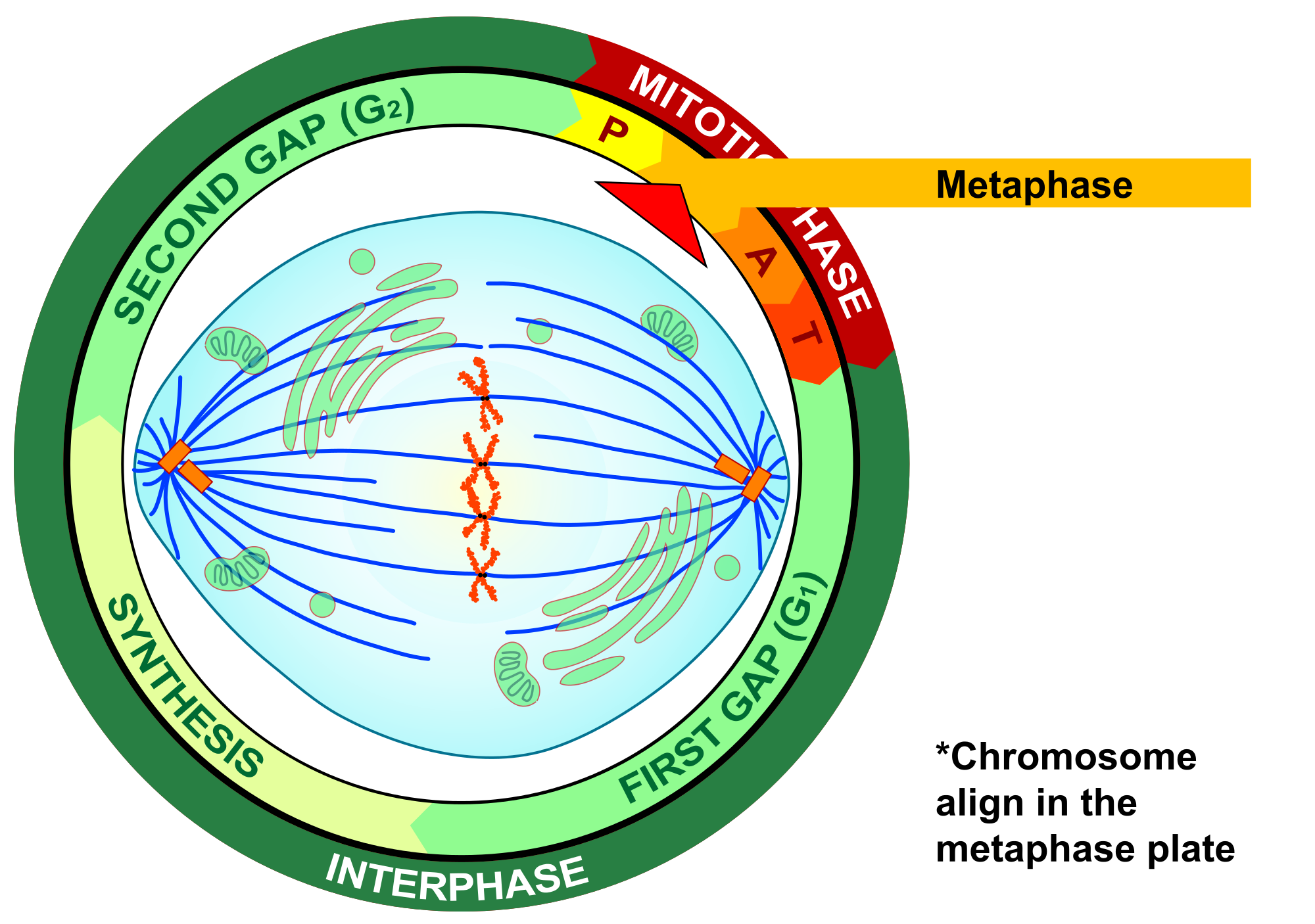
Anaphase
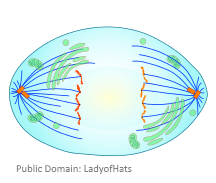
During anaphase, sister chromatids separate and the centromeres divide. The sister chromatids are pulled apart by the shortening of the spindle fibres. This is a little like reeling in a fish by shortening the fishing line. One sister chromatid moves to one pole of the cell, and the other sister chromatid moves to the opposite pole. At the end of anaphase, each pole of the cell has a complete set of chromosomes.
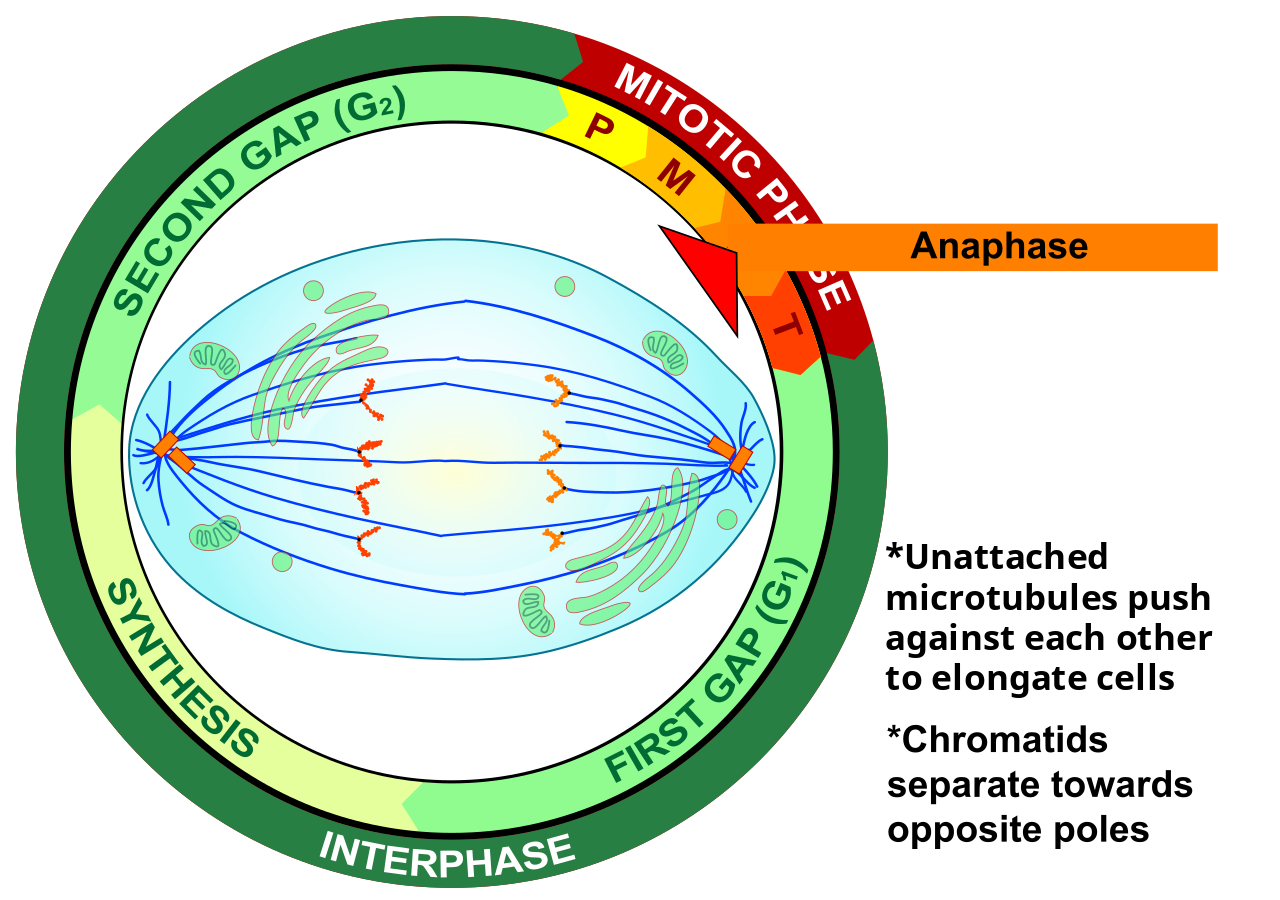
Telophase

During telophase, the chromosomes begin to uncoil and form chromatin. This prepares the genetic material for directing the metabolic activities of the new cells. The spindle also breaks down, and new nuclear envelopes form.
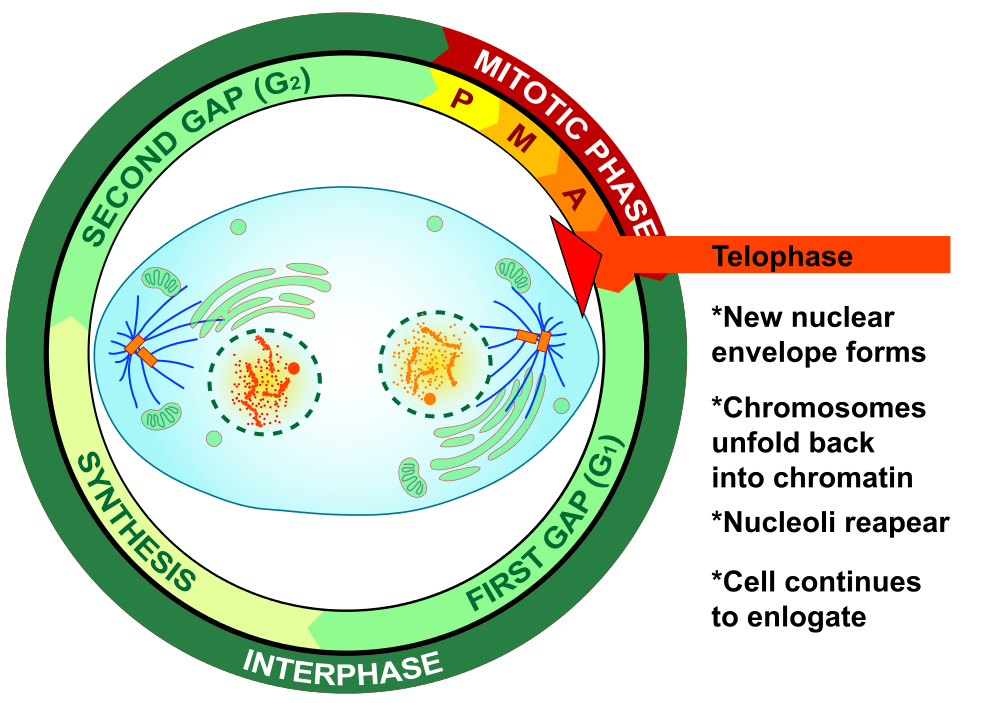
Cytokinesis
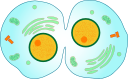
Cytokinesis is the final stage of cell division. During cytokinesis, the cytoplasm splits in two and the cell divides, as shown below. In animal cells, the plasma membrane of the parent cell pinches inward along the cell’s equator until two daughter cells form. Thus, the goal of mitosis and cytokinesis is now complete, because one parent cell has given rise to two daughter cells. The daughter cells have the same chromosomes as the parent cell.
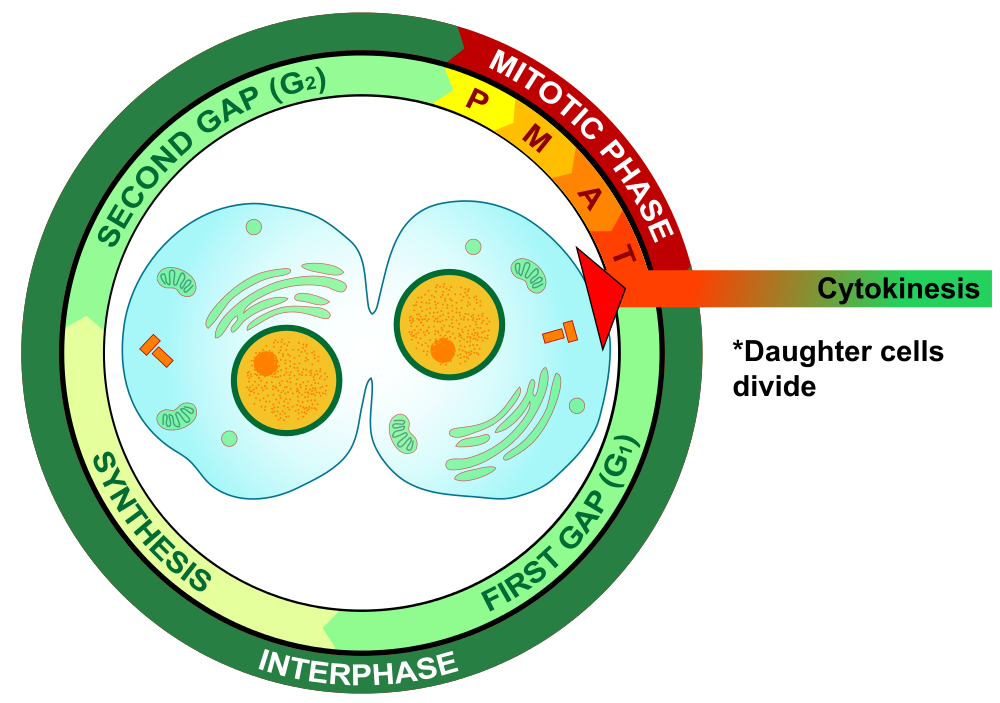
4.13 Summary
- Until a eukaryotic cell divides, its nuclear DNA exists as a grainy material called chromatin. After DNA replicates and the cell is about to divide, the DNA condenses and coils into the X-shaped form of a chromosome. Each chromosome actually consists of two sister chromatids, which are joined together at a centromere.
- Mitosis is the process during which the nucleus of a eukaryotic cell divides. During this process, sister chromatids separate from each other and move to opposite poles of the cell. This happens in four phases: prophase, metaphase, anaphase, and telophase.
- Cytokinesis is the final stage of cell division, during which the cytoplasm splits in two and two daughter cells form.
4.13 Review Questions
- Describe the different forms that DNA takes before and during cell division in a eukaryotic cell.
- Identify the four phases of mitosis in an animal cell, and summarize what happens during each phase.
- Order the diagrams of the stages of mitosis:
- Explain what happens during cytokinesis in an animal cell.
- What do you think would happen if the sister chromatids of one of the chromosomes did not separate during mitosis?
- True or False:
4.13 Explore More
https://www.youtube.com/watch?time_continue=3&v=C6hn3sA0ip0&feature=emb_logo
Mitosis, NDSU Virtual Cell Animations project (ndsuvirtualcell), 2012.
https://www.youtube.com/watch?time_continue=19&v=EA0qxhR2oOk&feature=emb_logo
Nondisjunction (Trisomy 21) - An Animated Tutorial, Kristen Koprowski, 2012.
Attributions
Figure 4.13.1
Anaphase_IF by Roy van Heesbeen on Wikimedia Commons is released into the public domain (https://en.wikipedia.org/wiki/Public_domain).
Figure 4.13.2
Chromosomes by OpenClipArt-Vectors on Pixabay is used under the Pixabay License (https://pixabay.com/service/license/).
Figure 4.13.3
Chromosome/ Chromatid/ Sister Chromatid by Christine Miller is released into the public domain (https://en.wikipedia.org/wiki/Public_domain).
Figure 4.13.4
Simple Mitosis by Mariana Ruiz Villarreal [LadyofHats] via CK-12 Foundation is used under a CC BY-NC 3.0 (https://creativecommons.org/licenses/by-nc/3.0/) license.
©CK-12 Foundation Licensed under
• Terms of Use • Attribution
Figure 4.13.5
Mitotic Prophase [tiny] by Mariana Ruiz Villarreal [LadyofHats] on Wikimedia Commons is released into the public domain (https://en.wikipedia.org/wiki/Public_domain).
Figure 4.13.6
Prophase Eukaryotic Mitosis by Mariana Ruiz Villarreal [LadyofHats] on Wikimedia Commons is released into the public domain (https://en.wikipedia.org/wiki/Public_domain).
Figure 4.13.7
Mitotic_Metaphase by Mariana Ruiz Villarreal [LadyofHats] on Wikimedia Commons is released into the public domain (https://en.wikipedia.org/wiki/Public_domain).
Figure 4.13.8
Metaphase Eukaryotic Mitosis by Mariana Ruiz Villarreal [LadyofHats] on Wikimedia Commons is released into the public domain (https://en.wikipedia.org/wiki/Public_domain).
Figure 4.13.9
Anaphase [adapted] by Mariana Ruiz Villarreal [LadyofHats] on Wikimedia Commons is released into the public domain (https://en.wikipedia.org/wiki/Public_domain).
Figure 4.13.10
Anaphase_eukaryotic_mitosis.svg by Mariana Ruiz Villarreal [LadyofHats] on Wikimedia Commons is released into the public domain (https://en.wikipedia.org/wiki/Public_domain).
Figure 4.13.11
Mitotic Telophase by Mariana Ruiz Villarreal [LadyofHats] on Wikimedia Commons is released into the public domain (https://en.wikipedia.org/wiki/Public_domain).
Figure 4.13.12
Telophase Eukaryotic Mitosis by Mariana Ruiz Villarreal [LadyofHats] on Wikimedia Commons is released into the public domain (https://en.wikipedia.org/wiki/Public_domain).
Figure 4.13.13
Mitotic Cytokinesis by Mariana Ruiz Villarreal [LadyofHats] on Wikimedia Commons is released into the public domain (https://en.wikipedia.org/wiki/Public_domain).
Figure 4.13.14
Cytokinesis Eukaryotic Mitosis by Mariana Ruiz Villarreal [LadyofHats] on Wikimedia Commons is released into the public domain (https://en.wikipedia.org/wiki/Public_domain).
References
Koprowski, K., Cabey, R. [Kristen Koprowski]. (2012). Nondisjunction (Trisomy 21) - An Animated Tutorial. YouTube. https://www.youtube.com/watch?v=EA0qxhR2oOk&feature=youtu.be
NDSU Virtual Cell Animations project [ndsuvirtualcell]. (2012). Mitosis. YouTube. https://www.youtube.com/watch?v=C6hn3sA0ip0&t=21s
Image shows the human life cycle. Haploid sperm and egg fuse to form a diploid zygote. From there, the fetus grows through mitosis, until is is born and is an infant. The infant grows through adolescence and into adulthood. Once in adulthood, gametes are created through meiosis and the cycle begins again.
Created by: CK-12/Adapted by Christine Miller
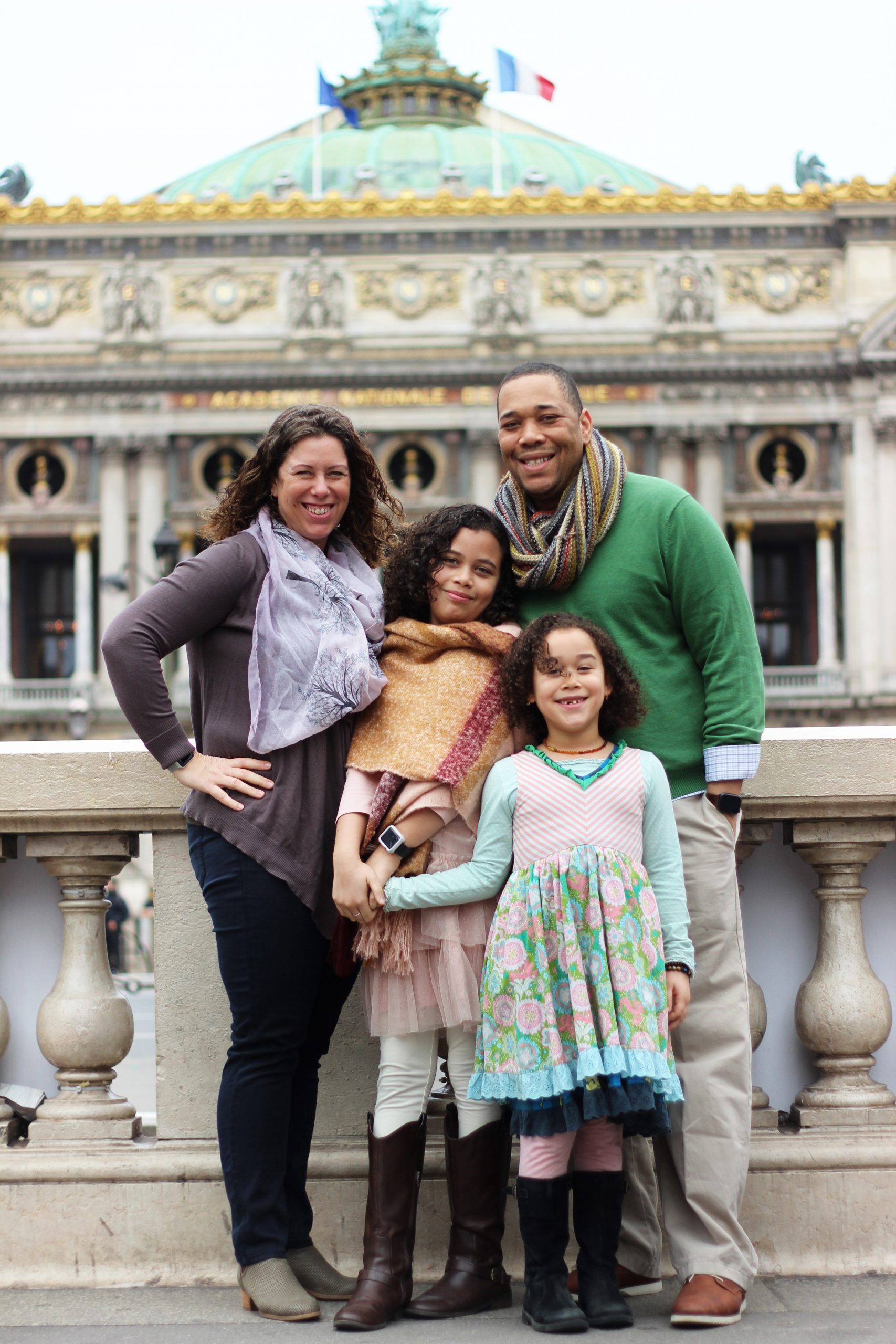
All in the Family
This family photo (Figure 5.12.1) clearly illustrates an important point: children in a family resemble their parents and each other, but the children never look exactly the same, unless they are identical twins. Each of the daughters in the photo have inherited a unique combination of traits from the parents. In this concept, you will learn how this happens. It all begins with sex — sexual reproduction, that is.
Sexual Reproduction
Reproduction is the process by which organisms give rise to offspring. It is one of the defining characteristics of living things. Like many other organisms, human beings reproduce sexually. Sexual reproduction involves two parents. As you can see from Figure 5.12.2, in sexual reproduction, parents produce reproductive (sex) cells — called gametes — that unite to form an offspring. Gametes are haploid (or 1N) cells. This means they contain one copy of each chromosome in the nucleus. Gametes are produced by a type of cell division called meiosis, which is described in detail below. The process in which two gametes unite is called fertilization. The fertilized cell that results is referred to as a zygote. A zygote is a diploid (or 2N) cell, which means it contains two copies of each chromosome. Thus, it has twice the number of chromosomes as a gamete.
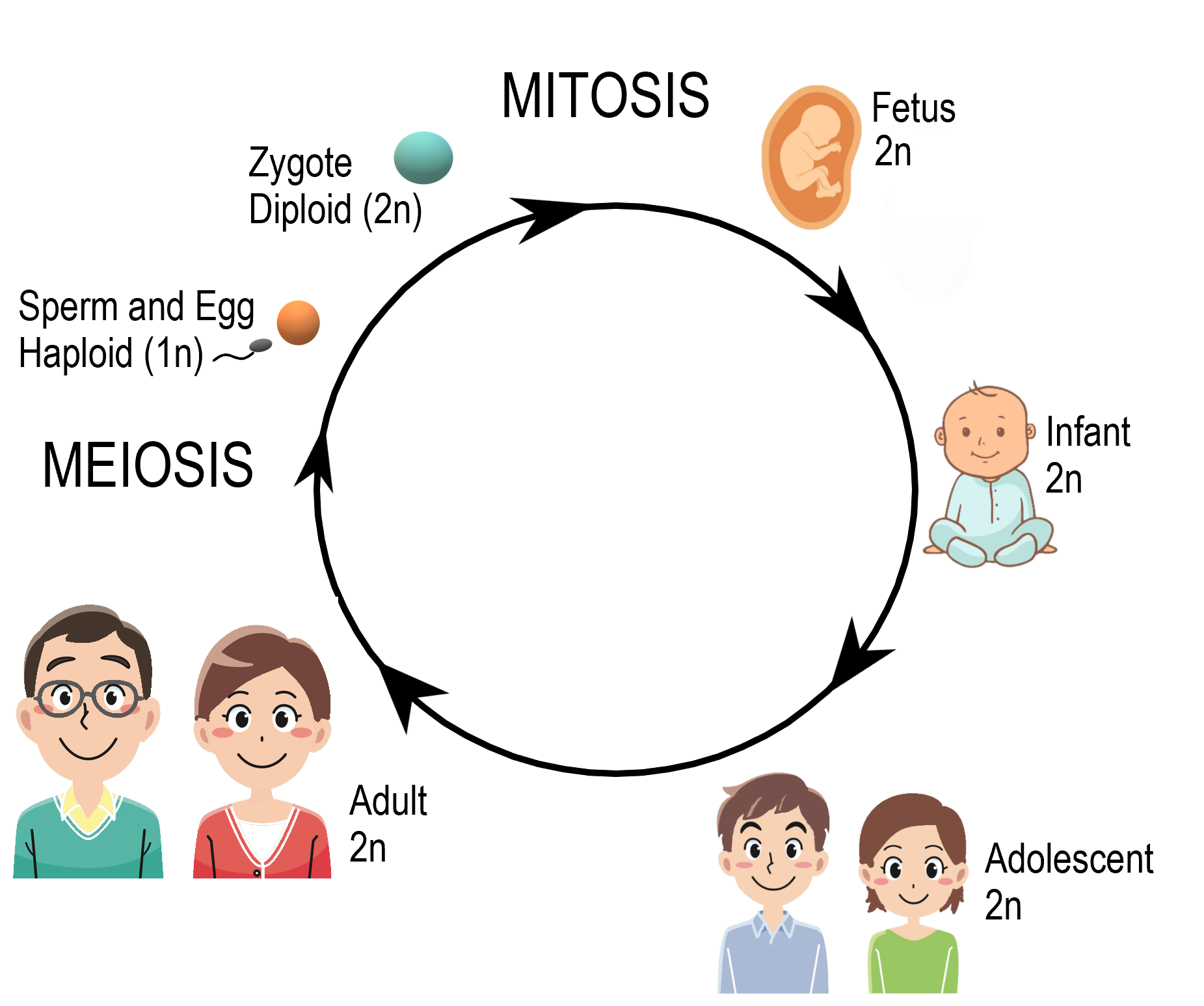
Meiosis
The process that produces haploid gametes is called meiosis. Meiosis is a type of cell division in which the number of chromosomes is reduced by half. It occurs only in certain special cells of an organism. During meiosis, homologous (paired) chromosomes separate, and four haploid cells form that have only one chromosome from each pair. The diagram (Figure 5.12.3) gives an overview of meiosis.
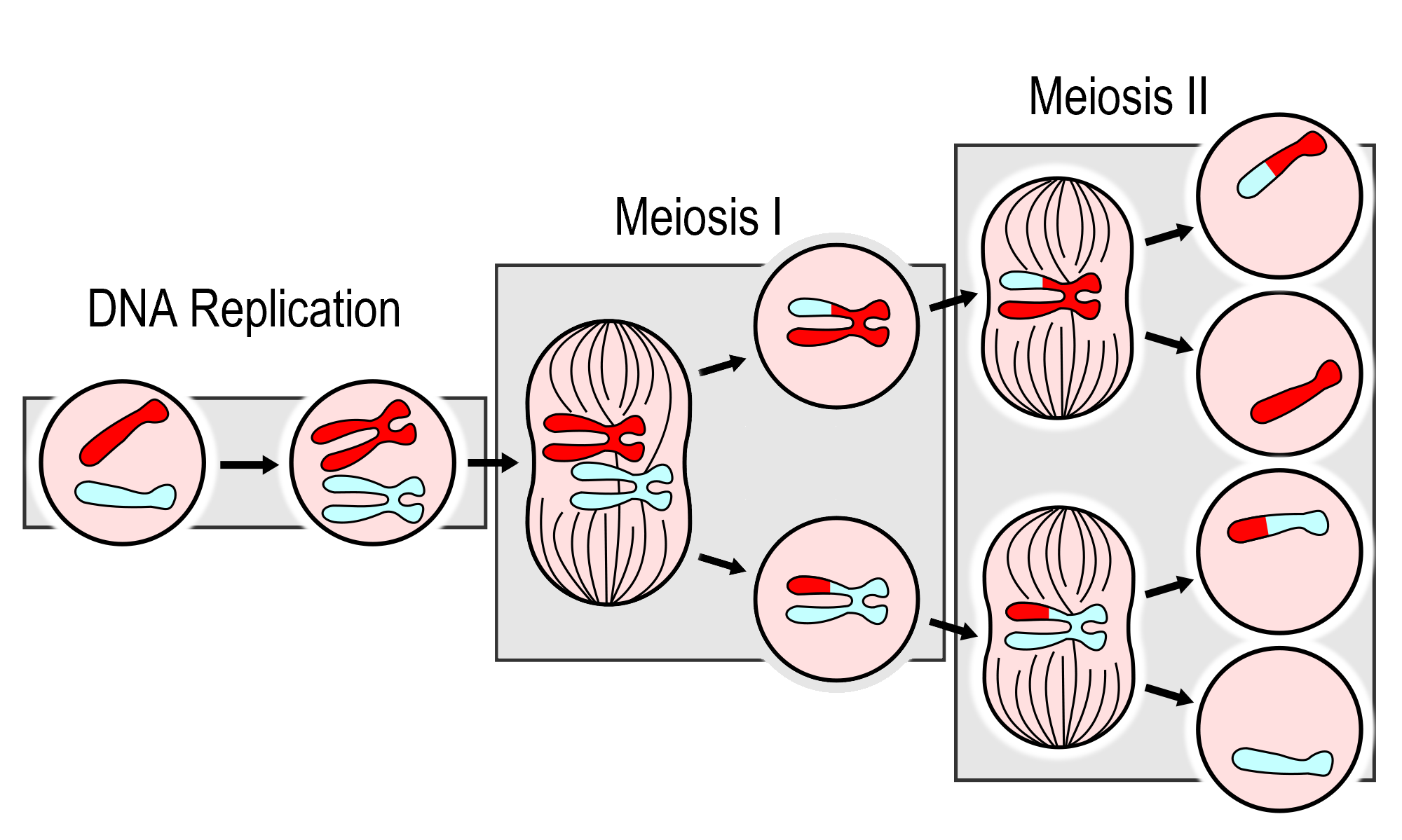
As you can see in the meiosis diagram, two cell divisions occur during the overall process, producing a total of four haploid cells from one parent cell. The two cell divisions are called meiosis I and meiosis II. Meiosis I begins after DNA replicates during interphase. Meiosis II follows meiosis I without DNA replicating again. Both meiosis I and meiosis II occur in four phases, called prophase, metaphase, anaphase, and telophase. You may recognize these four phases from mitosis, the division of the nucleus that takes place during routine cell division of eukaryotic cells.
Meiosis I- Increasing genetic variation
The phases of Meiosis I are:
- Prophase I: The nuclear envelope begins to break down, and the chromosomes condense. Centrioles start moving to opposite poles of the cell, and a spindle begins to form. Importantly, homologous chromosomes pair up, which is unique to prophase I. In prophase of mitosis and meiosis II, homologous chromosomes do not form pairs in this way. During prophase I, crossing-over occurs. The significance of crossing-over is discussed below.
- Metaphase I: Spindle fibres attach to the paired homologous chromosomes. The paired chromosomes line up along the equator of the cell, randomly aligning in a process called independent alignment. The significance of independent alignment is discussed below. This occurs only in metaphase I. In metaphase of mitosis and meiosis II, it is sister chromatids that line up along the equator of the cell.
- Anaphase I: Spindle fibres shorten, and the chromosomes of each homologous pair start to separate from each other. One chromosome of each pair moves toward one pole of the cell, and the other chromosome moves toward the opposite pole.
- Telophase I and Cytokinesis: The spindle breaks down, and new nuclear membranes form. The cytoplasm of the cell divides, and two haploid daughter cells result. The daughter cells each have a random assortment of chromosomes, with one from each homologous pair. Both daughter cells go on to meiosis II.
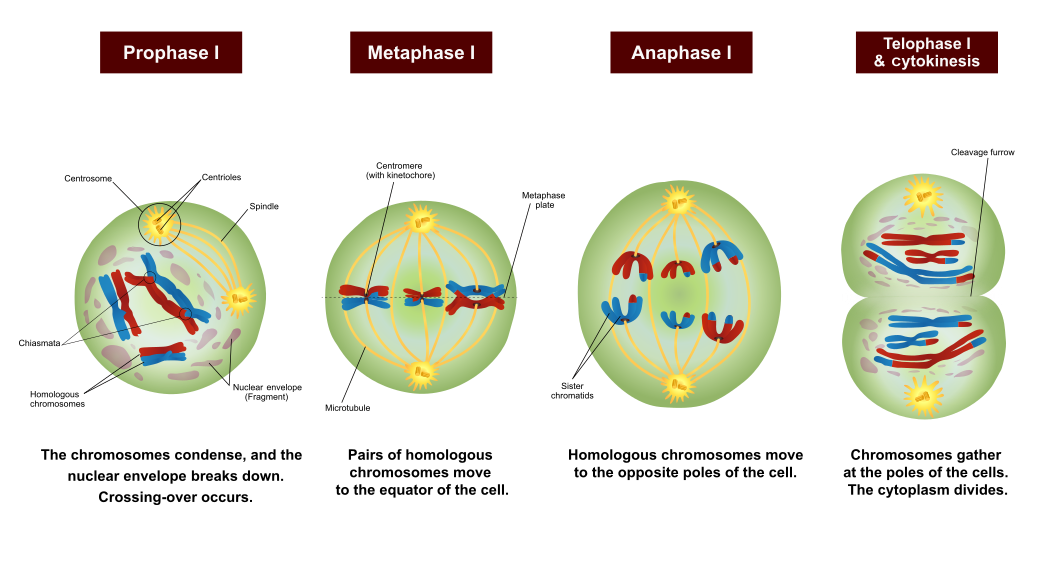
Meiosis II- Halfing the DNA
The phases of Meiosis II are:
- Prophase II: The nuclear envelope breaks down, and the spindle begins to form in each haploid daughter cell from meiosis I. The centrioles also start to separate.
- Metaphase II: Spindle fibres line up the sister chromatids of each chromosome along the equator of the cell.
- Anaphase II: Sister chromatids separate and move to opposite poles.
- Telophase II and Cytokinesis: The spindle breaks down, and new nuclear membranes form. The cytoplasm of each cell divides, and four haploid cells result. Each cell has a unique combination of chromosomes.
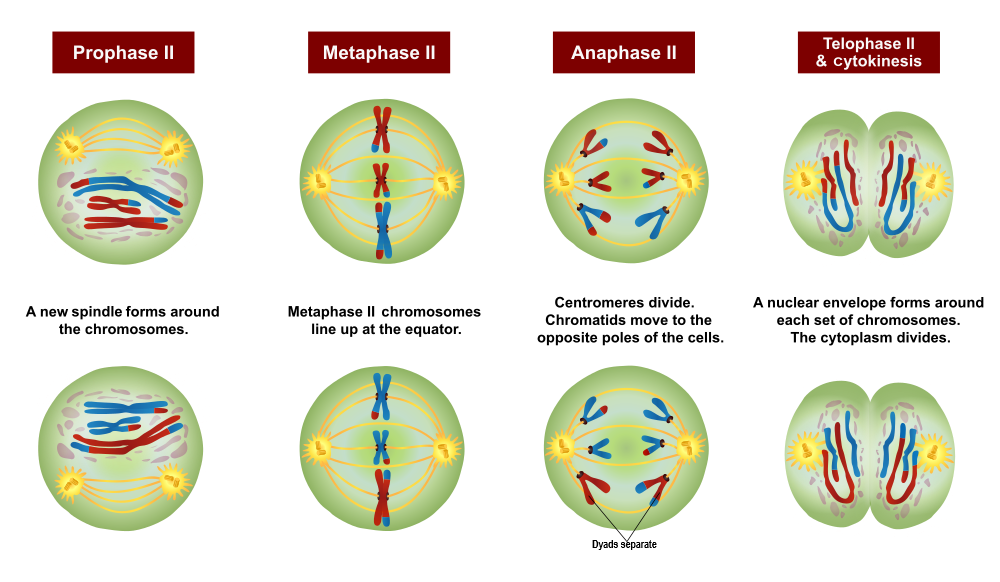
Sexual Reproduction and Genetic Variation
"It takes two to tango" might be a euphemism for sexual reproduction. Requiring two individuals to produce offspring, however, is also the main drawback of this way of reproducing, because it requires extra steps — and often a certain amount of luck — to successfully reproduce with a partner. On the other hand, sexual reproduction greatly increases the potential for genetic variation in offspring, which increases the likelihood that the resulting offspring will have genetic advantages. In fact, each offspring produced is almost guaranteed to be genetically unique, differing from both parents and from any other offspring. Sexual reproduction increases genetic variation in a number of ways:
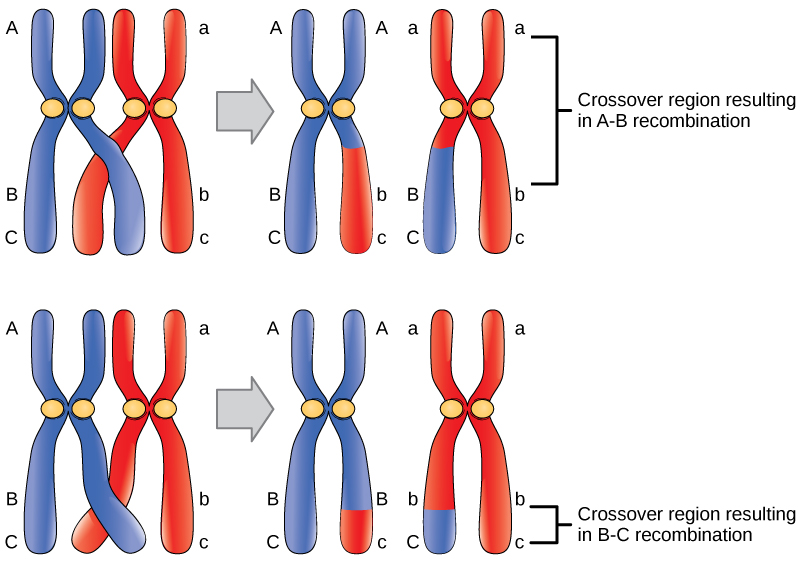
- When homologous chromosomes pair up during meiosis I, crossing-over can occur. Crossing-over is the exchange of genetic material between non-sister chromatids of homologous chromosomes. It results in new combinations of genes on each chromosome. This is called recombination. You can see how it happens in the figure to the right.
- When cells divide during meiosis, homologous chromosomes are randomly distributed to daughter cells, and different chromosomes segregate independently of each other. This is called independent alignment. It results in gametes that have unique combinations of chromosomes. You can see how it happens in Figure 5.12.7.
- In sexual reproduction, two gametes unite to produce an offspring. But which two of the millions of possible gametes will it be? This is a matter of chance, and it's obviously another source of genetic variation in offspring.
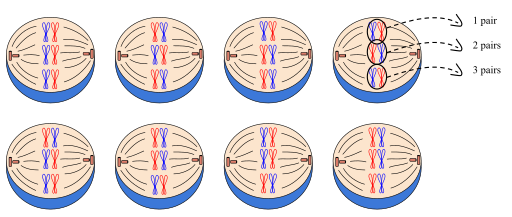
With all of this recombination of genes, there is a need for a new set of vocabulary. Remember, that sister chromatids are two identical pieces of DNA connected at a centromere. Once crossing over has occured, we can no longer call them sister chromatids since they are no longer identical; we term them dyads. In addition, once crossing over has occurred, the pair of homologous chromosomes can be referred to as tetrads.
All of these mechanisms — crossing over, independent assortment, and the random union of gametes — work together to result in an amazing range of potential genetic variation. Each human couple, for example, has the potential to produce more than 64 trillion genetically unique children. No wonder we are all different!
https://www.youtube.com/watch?v=VzDMG7ke69g
Meiosis (updated), Amoeba Sisters, 2017.
Gametogenesis
At the end of meiosis, four haploid cells have been produced, but the cells are not yet gametes. The cells need to develop before they become mature gametes capable of fertilization. The development of haploid cells into gametes is called gametogenesis. It differs between males and females.
- A gamete produced by a male is called a sperm, and the process that produces a mature sperm is called spermatogenesis. During this process, a sperm cell grows a tail and gains the ability to “swim,” like the human sperm cell shown in Figure 5.12.8.
- A gamete produced by a female is called anegg or ovum, and the process that produces a mature egg is called oogenesis, during which just one functional egg is produced. The other three haploid cells that result from meiosis are called polar bodies, and they disintegrate. The single egg is a very large cell, as you can see from the human egg also shown in Figure 5.12.8.
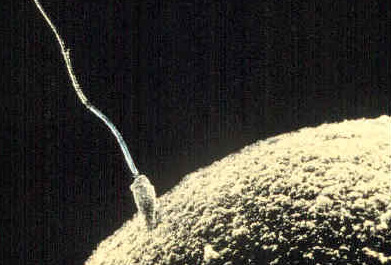
5.12 Summary
- In sexual reproduction, two parents produce gametes that unite in the process of fertilization to form a single-celled zygote. Gametes are haploid cells with one copy of each of the 23 chromosomes, and the zygote is a diploid cell with two copies of each of the 23 chromosomes.
- Meiosis is the type of cell division that produces four haploid daughter cells that may become gametes. Meiosis occurs in two stages, called meiosis I and meiosis II, each of which occurs in four phases (prophase, metaphase, anaphase, and telophase).
- Meiosis is followed by gametogenesis, the process during which the haploid daughter cells change into mature gametes. Males produce gametes called sperm in a process known as spermatogenesis, and females produce gametes called eggs in the process known as oogenesis.
- Sexual reproduction produces genetically unique offspring. Crossing-over, independent alignment, and the random union of gametes work together to result in an amazing range of potential genetic variation.
5.12 Review Questions
-
- Explain how sexual reproduction happens at the cellular level.
- Summarize what happens during Meiosis.
- Compare and contrast gametogenesis in males and females.
- Explain the mechanisms that increase genetic variation in the offspring produced by sexual reproduction.
- Why do gametes need to be haploid? What would happen to the chromosome number after fertilization if they were diploid?
- Describe one difference between Prophase I of Meiosis and Prophase of Mitosis.
- Do all of the chromosomes that you got from your mother go into one of your gametes? Why or why not?
5.12 Explore More
https://www.youtube.com/watch?v=qCLmR9-YY7o&feature=emb_logo
Meiosis: Where the Sex Starts - Crash Course Biology #13, CrashCourse, 2012.
https://www.youtube.com/watch?v=zrKdz93WlVk
Mitosis vs Meiosis Comparison, Amoeba Sisters, 2018.
Attributions
Figure 5.12.1
Family portrait by loly galina on Unsplash is used under the Unsplash License (https://unsplash.com/license).
Figure 5.12.2
Human Life Cycle by Christine Miller is used under a CC BY-NC-SA 4.0 (https://creativecommons.org/licenses/by-nc-sa/4.0/) license.
Figure 5.12.3
MajorEventsInMeiosis_variant_int by PatríciaR (internationalization) on Wikimedia Commons is used and adapted by Christine Miller. This image in the public domain. (Original image from NCBI; original vector version by Jakov.)
Figure 5.12.4
Meiosis 1/ Meiosis Stages by Ali Zifan on Wikimedia Commons is used and adapted by Christine Miller under a CC BY-SA 4.0 (https://creativecommons.org/licenses/by-sa/4.0) license.
Figure 5.12.5
Meiosis 2/ Meiosis Stages by Ali Zifan on Wikimedia Commons is used and adapted by Christine Miller under a CC BY-SA 4.0 (https://creativecommons.org/licenses/by-sa/4.0) license.
Figure 5.12.6
Crossover/ Figure 17 02 01 by CNX OpenStax on Wikimedia Commons is used under a CC BY 4.0 (https://creativecommons.org/licenses/by/4.0) license.
Figure 5.12.7
Independent_assortment by Mtian20 on Wikimedia Commons is used under a CC BY-SA 4.0 (https://creativecommons.org/licenses/by-sa/4.0) license.
Figure 5.12.8
sperm fertilizing egg by AndreaLaurel on Flickr is used under a CC BY 2.0 (https://creativecommons.org/licenses/by/2.0/) license.
References
Amoeba Sisters. (2017, July 11). Meiosis (updated). YouTube. https://www.youtube.com/watch?v=VzDMG7ke69g&feature=youtu.be
Amoeba Sisters. (2018, May 31). Mitosis vs meiosis comparison. YouTube. https://www.youtube.com/watch?v=zrKdz93WlVk&feature=youtu.be
CrashCourse, (2012, April 23). Meiosis: Where the sex starts - Crash Course Biology #13. YouTube. https://www.youtube.com/watch?v=qCLmR9-YY7o&feature=youtu.be
OpenStax CNX. (2016, May 27). Figure 1 Crossover may occur at different locations on the chromosome. In OpenStax, Biology (Section 17.2). http://cnx.org/contents/185cbf87-c72e-48f5-b51e-f14f21b5eabd@10.53.
Image shows the four stages of Meiosis II: In Prophase II, the dyads condense, the nuclear envelope fragments and spindle fibers form. In Metaphase II, the dyads align at the center of the cell. In Anaphase II, the dyads are split pulled to opposite ends of the cell. In Telophase II the chromatids decondense, nuclear envelope reforms and spindle fibers fragment.
Image shows the process of crossing over. A pair of homologous chromosomes are side by side. They then overlap the ends of their structures and trades these sections, attaching some maternal DNA onto the paternal chromosome, and some paternal DNA onto the maternal chromosome. The result is that, within the homologous pair of chromosomes, each of the four pieces of DNA, destined to enter 4 separate cells, is now consists of a unique mix of genes.
Image illustrates how independent alignment greatly increases the genetic diversity among gametes produced. In a single cell undergoing Meiosis, independent alignment means that whether the paternal or maternal chromosome for each homologous pair ends up on the left or right is totally random, and is random for each pair of homologous chromosomes. In a cell which has 3 pairs of homologous chromosomes, there are 8 possible random alignments, which would each result in a different set of gametes being produced.
Image shows a sperm fertilizing an egg. The Sperm is much smaller than the egg.
Created by: CK-12/Adapted by Christine Miller
Case Study: Cancer in the Family
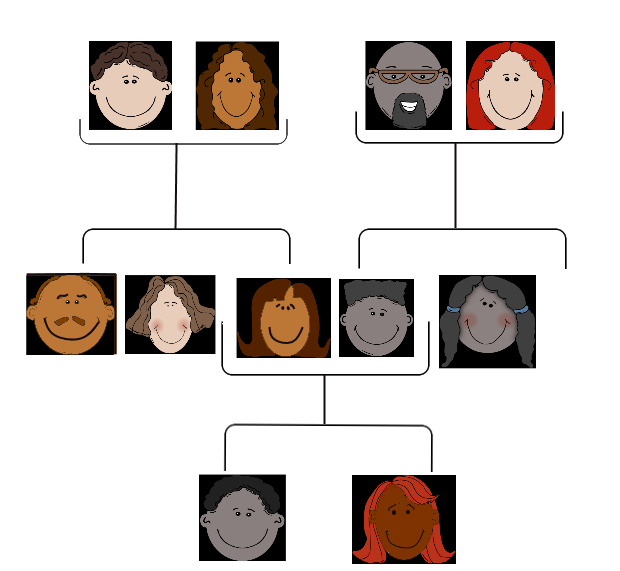
People tend to carry similar traits to their biological parents, as illustrated by the family tree. Beyond just appearance, you can also inherit traits from your parents that you can’t see.
Rebecca becomes very aware of this fact when she visits her new doctor for a physical exam. Her doctor asks several questions about her family medical history, including whether Rebecca has or had relatives with cancer. Rebecca tells her that her grandmother, aunt, and uncle — who have all passed away — had cancer. They all had breast cancer, including her uncle, and her aunt also had ovarian cancer. Her doctor asks how old they were when they were diagnosed with cancer. Rebecca is not sure exactly, but she knows that her grandmother was fairly young at the time, probably in her forties.
Rebecca’s doctor explains that while the vast majority of cancers are not due to inherited factors, a cluster of cancers within a family may indicate that there are mutations in certain genes that increase the risk of getting certain types of cancer, particularly breast and ovarian cancer. Some signs that cancers may be due to these genetic factors are present in Rebecca’s family, such as cancer with an early age of onset (e.g., breast cancer before age 50), breast cancer in men, and breast cancer and ovarian cancer within the same person or family.
Based on her family medical history, Rebecca’s doctor recommends that she see a genetic counselor, because these professionals can help determine whether the high incidence of cancers in her family could be due to inherited mutations in their genes. If so, they can test Rebecca to find out whether she has the particular variations of these genes that would increase her risk of getting cancer.
When Rebecca sees the genetic counselor, he asks how her grandmother, aunt, and uncle with cancer are related to her. She says that these relatives are all on her mother’s side — they are her mother’s mother and siblings. The genetic counselor records this information in the form of a specific type of family tree, called a pedigree, indicating which relatives had which type of cancer, and how they are related to each other and to Rebecca.
He also asks her ethnicity. Rebecca says that her family on both sides are Ashkenazi Jews (Jews whose ancestors came from central and eastern Europe). “But what does that have to do with anything?” she asks. The counselor tells Rebecca that mutations in two tumor-suppressor genes called BRCA1 and BRCA2, located on chromosome 17 and 13, respectively, are particularly prevalent in people of Ashkenazi Jewish descent and greatly increase the risk of getting cancer. About one in 40 Ashkenazi Jewish people have one of these mutations, compared to about one in 800 in the general population. Her ethnicity, along with the types of cancer, age of onset, and the specific relationships between her family members who had cancer, indicate to the counselor that she is a good candidate for genetic testing for the presence of these mutations.
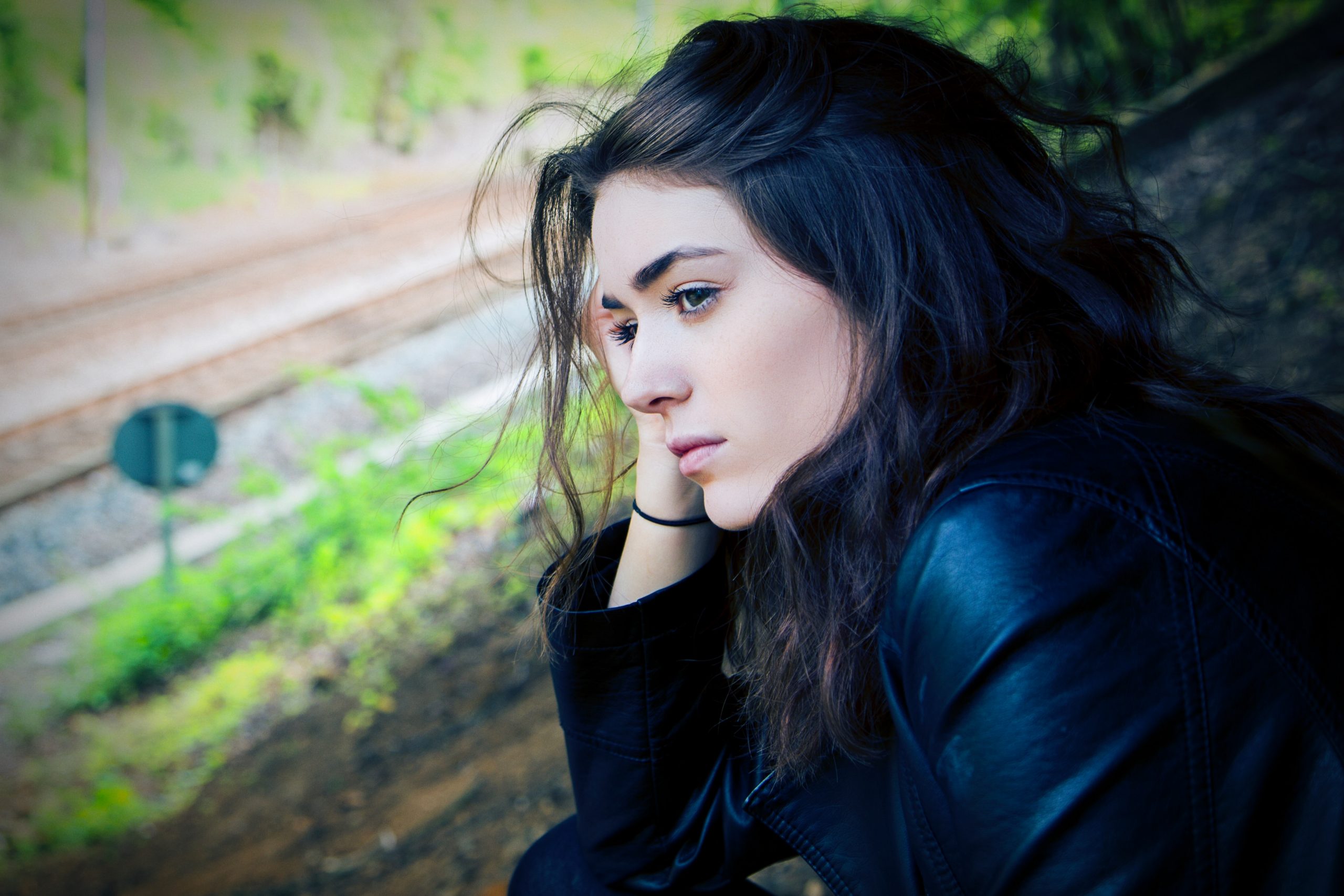
Rebecca says that her 72-year-old mother never had cancer, nor had many other relatives on that side of the family. How could the cancers be genetic? The genetic counselor explains that the mutations in the BRCA1 and BRCA2 genes, while dominant, are not inherited by everyone in a family. Also, even people with mutations in these genes do not necessarily get cancer — the mutations simply increase their risk of getting cancer. For instance, 55 to 65 per cent of women with a harmful mutation in the BRCA1 gene will get breast cancer before age 70, compared to 12 per cent of women in the general population who will get breast cancer sometime over the course of their lives.
Rebecca is not sure she wants to know whether she has a higher risk of cancer. The genetic counselor understands her apprehension, but explains that if she knows that she has harmful mutations in either of these genes, her doctor will screen her for cancer more often and at earlier ages. Therefore, any cancers she may develop are likely to be caught earlier when they are often much more treatable. Rebecca decides to go through with the testing, which involves taking a blood sample, and nervously waits for her results.
Chapter Overview: Genetics
At the end of this chapter, you will find out Rebecca’s test results. By then, you will have learned how traits are inherited from parents to offspring through genes, and how mutations in genes such as BRCA1 and BRCA2 can be passed down and cause disease. Specifically, you will learn about:
- The structure of DNA.
- How DNA replication occurs.
- How DNA was found to be the inherited genetic material.
- How genes and their different alleles are located on chromosomes.
- The 23 pairs of human chromosomes, which include autosomal and sex chromosomes.
- How genes code for proteins using codons made of the sequence of nitrogen bases within RNA and DNA.
- The central dogma of molecular biology, which describes how DNA is transcribed into RNA, and then translated into proteins.
- The structure, functions, and possible evolutionary history of RNA.
- How proteins are synthesized through the transcription of RNA from DNA and the translation of protein from RNA, including how RNA and proteins can be modified, and the roles of the different types of RNA.
- What mutations are, what causes them, different specific types of mutations, and the importance of mutations in evolution and to human health.
- How the expression of genes into proteins is regulated and why problems in this process can cause diseases, such as cancer.
- How Gregor Mendel discovered the laws of inheritance for certain types of traits.
- The science of heredity, known as genetics, and the relationship between genes and traits.
- How gametes, such as eggs and sperm, are produced through meiosis.
- How sexual reproduction works on the cellular level and how it increases genetic variation.
- Simple Mendelian and more complex non-Mendelian inheritance of some human traits.
- Human genetic disorders, such as Down syndrome, hemophilia A, and disorders involving sex chromosomes.
- How biotechnology — which is the use of technology to alter the genetic makeup of organisms — is used in medicine and agriculture, how it works, and some of the ethical issues it may raise.
- The human genome, how it was sequenced, and how it is contributing to discoveries in science and medicine.
As you read this chapter, keep Rebecca’s situation in mind and think about the following questions:
- BCRA1 and BCRA2 are also called Breast cancer type 1 and 2 susceptibility proteins. What do the BRCA1 and BRCA2 genes normally do? How can they cause cancer?
- Are BRCA1 and BRCA2 linked genes? Are they on autosomal or sex chromosomes?
- After learning more about pedigrees, draw the pedigree for cancer in Rebecca’s family. Use the pedigree to help you think about why it is possible that her mother does not have one of the BRCA gene mutations, even if her grandmother, aunt, and uncle did have it.
- Why do you think certain gene mutations are prevalent in certain ethnic groups?
Attributions
Figure 5.1.1
Family Tree [all individual face images] from Clker.com used and adapted by Christine Miller under a CC0 1.0 public domain dedication license (https://creativecommons.org/publicdomain/zero/1.0/).
Figure 5.1.2
Rebecca by Kyle Broad on Unsplash is used under the Unsplash License (https://unsplash.com/license).
References
Wikipedia contributors. (2020, June 27). Ashkenazi Jews. In Wikipedia. https://en.wikipedia.org/w/index.php?title=Ashkenazi_Jews&oldid=964691647
Wikipedia contributors. (2020, June 22). BRCA1. In Wikipedia. https://en.wikipedia.org/w/index.php?title=BRCA1&oldid=963868423
Wikipedia contributors. (2020, May 25). BRCA2. In Wikipedia. https://en.wikipedia.org/w/index.php?title=BRCA2&oldid=958722957