Gold Leaching
Gold Leaching
Gold occurs in nature as the element (more commonly as an alloy of Au and Ag, or Au and Te, etc.). Ores may contain as little as 1-10 g Au/t (only 1-10 parts per million!). Gold metal is insoluble in water, regardless of pH. Can it be oxidized? There are two common oxidation states. Au(I) and Au(III). The simple cations Au+ and Au+3 are both powerfully oxidizing:
[latex]Au^{+}\:_{aq}\:+\:e^{-}\:=\:Au_{s}\:\:\:\:\:\:\:\:\:\:E^{\circ}\:=\:1.69\:V \tag{16}[/latex]
[latex]Au^{+3}\:_{aq}\:+\:3e^{-}\:=\:Au\:_{s}\:\:\:\:\:\:\:\:\:\:E^{\circ}\:=\:1.41\:V \tag{17}[/latex]
Compare this with the stability of water:
[latex]O_{2\:g}\:+\:4H^{+}\:_{aq}\:+\:4e^{-}\:=\:2H_{2}O\:_{l}\:\:\:\:\:\:\:\:\:\:E^{\circ}\:=\:1.23\:V \tag{18}[/latex]
Even in strongly acidic solution (aH+ = 1 m) either cation is thermodynamically able to oxidize water to form O2. An Eh-pH diagram for the Au-H2O system would show only one species within the bounds of stability of water at all pH – Au metal. What about the kinetics? After all, some powerful oxidizing agents can be dissolved in water even though thermodynamics says they should oxidize it to O2. Two examples are cerric sulfate, i.e., Ce(IV) in sulfuric acid solution (E° = 1.44 V) and KMnO4 (E° = 1.51 V). However, experience confirms that both Au+ and Au+3 are rapidly reduced by water at all pH. Leaching gold by simple oxidation is not an option.
There are no known stable uncharged complexes of Au so complexation alone is not an option either. This leaves only the possibilities of routes 4 in
Figure 1. There are a number of known Au(I) complexes, including [Au(CN)2]–, [Au(S2O3)2]3-, [Au(SC(NH2))2]+, etc. and some Au(III) complexes, e.g. [AuCl4]–. The most stable is [Au(CN)2]–. An Eh-pH diagram for the Au-H2O-CN system is shown in Figure 5. The gold-cyanide complex is seen to be exceedingly stable. The complex can be formed by reaction between gold metal, cyanide and oxygen from air:
[latex]2Au_{s}\:+\:4CN^{-}\:_{aq}\:+\:O_{2}\:_{g}\:+\:2H_{2}O\:_{l}\:=\:2[Au(CN)_{2}]^{-}\:+\:H_{2}O_{2}\:_{aq}\:+\:2OH^{-}\:_{aq} \tag{19}[/latex]
(Hydrogen peroxide, H2O2, is a strong oxidant and will react with various species in solution, being reduced to OH– in the end.) The process was patented in 1887 and is the basis for much of the world’s gold industry today. This leaching reaction is always carried out in a basic solution. However, the cyanide complex is stable even in acid solution. So why not run the process in acid (which is cheaper than base)? First, the reaction is slow in acid. But, more importantly, CN– is a weak base:
[latex]HCN_{aq}\:=\:H^{+}\:_{aq}\:+\:CN^{-}\:_{aq}\:\:\:\:\:\:\:\:\:\:K_{a}\:=\:5.25\:\times\:10^{-10};\:pK_{a}\:=\:9.28 \tag{20}[/latex]
In acid solution, the reverse reaction is very favourable (1/Ka = 1.91 x 109). Furthermore, the vapour pressure for HCN over an aqueous solution is significant:
[latex]HCN_{aq}\:=\:HCN_{g}\:\:\:\:\:\:\:\:\:\:K\:=\:0.1 \tag{21}[/latex]
And, finally, HCN is very toxic. In the end basic conditions are a must. The pH required for effective leaching and occupational safety is around 10.5-11. Lime is able to sustain this pH easily, so it is used rather than the more costly NaOH.
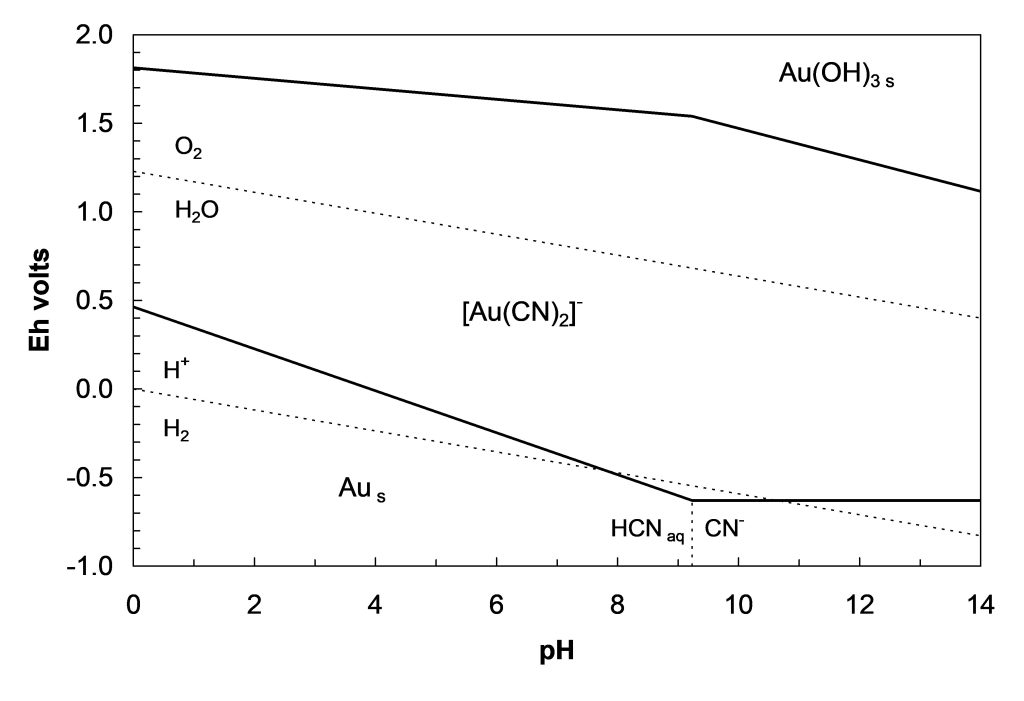
However, cyanide leaching is not without problems. Sodium cyanide is a very expensive reagent ($1000-2000 per tonne). One of the main issues in cyanide leaching for Au is excessive reagent consumption. Many metal ions form very stable cyanide complexes (e.g. [Cu(CN)3]–, [Zn(CN)4]2-, [Fe(CN)6]4-, etc.); CN– is fairly easy to oxidize, forming OCN– in some cases; SCN– is readily formed by reactions of some metal sulfides with cyanide. Many copper-gold mineral deposits with modest concentrations of some copper minerals cannot be economically produced due to excessive cyanide consumption. Finally, cyanide has a bad reputation and obtaining permits to open a new gold cyanidation plant may be very difficult in several jurisdictions.
The Eh-pH diagram for the Au-H2O-Cl system is shown in Figure 6. The complexing species (NaCl and HCl for pH adjustment) are relatively inexpensive. Au can be leached by a suitable oxidant in HCl solution, but, a rather high potential is needed (close to 1 V; lower as aCl- increases) plus a high chloride concentration. This presents a number of problems. O2 will work in principle, but it is slow in practice. Other strong oxidants will tend to be expensive. Consumption of the oxidant may be high for an ore consisting of sulfide minerals (which are fairly easy to oxidize), or other oxidizable constituents (and steel). Further, AuCl4- is a strong oxidant, so even mildly reducing materials in the ore will likely re-precipitate Au metal, defeating the purpose. The strong chloride solutions are quite corrosive toward steel, so materials of construction problems pertain. It is not hard to see why this type of chemistry is not well suited for gold leaching in general.
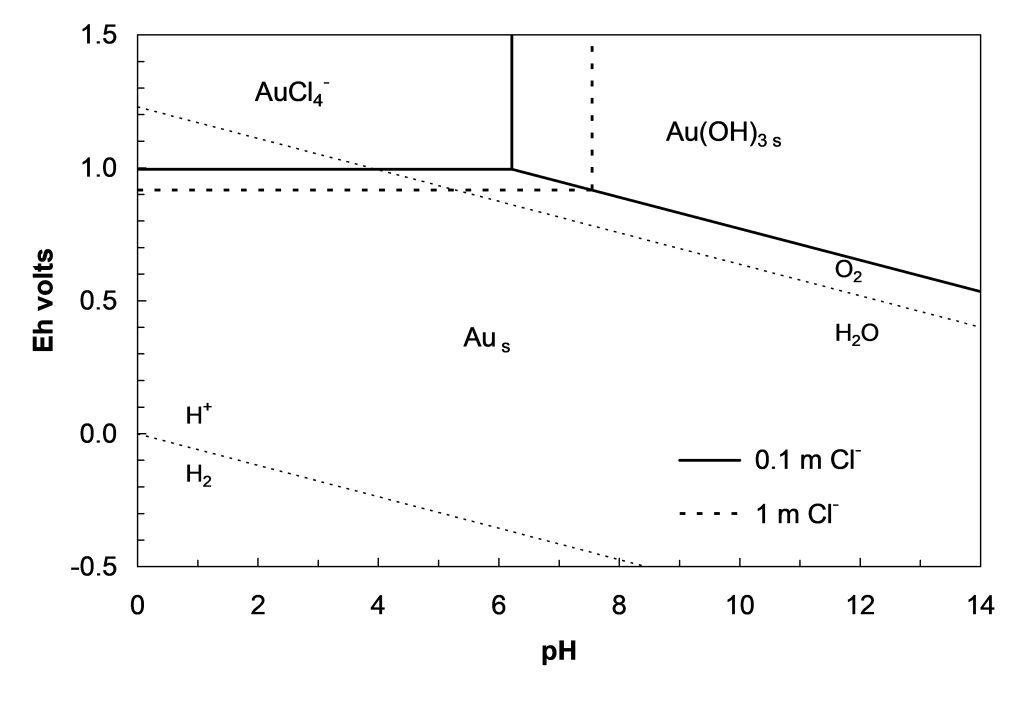
Bauxite Leaching
Bauxite is a mixture of aluminum oxide-hydroxide minerals. The principal components are Al(OH)3, Al(O)OH and Al2O3·H2O. The final product of processing is Al2O3. Bauxite ores contain the equivalent of 40-60% Al2O3. The solubility of the minerals increases in the order: Al2O3 < Al(O)OH < Al(OH)3·H2O; the latter two are the most commonly treated minerals. Both Al(O)OH and Al(OH)3 are soluble in strongly acidic and strongly basic solutions; the compounds are amphoteric (both weak acids and weak bases).
In basic solution:
[latex]Al(OH)_{3}\:_{s}\:+\:OH^{-}\:=\:[Al(OH)_{4}]^{-}\:_{aq} \tag{22}[/latex]
[latex]Al(O)OH\:_{s}\:+\:H_{2}O_{l}\:+\:OH^{-}\:_{aq}\:=\:[Al(OH)_{4}]^{-}\:_{aq}\tag{23}[/latex]
[latex]0.5\text{Al}_2O_{3s} + 1.5\text{H}_{2\text{O}_l} + \text{OH}^-{}_{\text{aq}} = [\text{Al}(\text{OH}_4)]^-{}_{\text{aq}}\tag{24}[/latex]
In acid solution:
[latex]Al(OH)_{3}\:_{s}\:+\:3H^{+}\:_{aq}\:=\:Al^{+3}\:_{aq}\:+3H_{2}O\:_{l}\tag{25}[/latex]
[latex]Al(O)OH\:_{s}\:+\:3H^{+}\:_{aq}\:=\:Al^{+3}\:_{aq}\:+\:2H_{2}O\:_{l}\tag{26}[/latex]
[latex]0.5Al_{2}O_{3}\:_{s}\:+\:3H^{+}\:_{aq}\:=\:Al^{+3}\:_{aq}\:+\:1.5H_{2}O\:_{l}\tag{27}[/latex]
Aqueous Al+3 is present as [Al(H2O)6]+3 at very low pH, and as various OH-/H2O complexes (hydrolyzed) as pH increases. The minimum solubility occurs at pH ~7. Either strong base (NaOH) or strong acid is needed (e.g. H2SO4 or HCl) to attain the necessary high or low pH, respectively. Solubility is significantly enhanced by increasing temperature, and base or acid concentration. This is illustrated in Figure 7. Here the equilibrium concentration of dissolved gibbsite, i.e. Al(OH)3, is plotted as a function of temperature for various concentrations of NaOH. Note that the solubility is expressed as Al2O3 in g/L, since that is the final product of the Bayer process; 1 g Al(OH)3 = 0.6536 g Al2O3.
In fact, the Bayer process (patented in 1887) uses the strong base route at elevated temperatures (autocalves). One might expect that H2SO4 or HCl would be used since NaOH is so much more expensive. There are a number of reasons why acid leaching is not used. A few of the key rationales are provided here.
- Ferric-bearing minerals are much more soluble in acid solution than in basic solution. Then a leach solution purification step for iron removal would be needed. Ferric ion, Fe+3, is chemically similar in some respects to Al+3 and removing ferric iron is prohibitively expensive.
Figure 7. Equilibrium solubility of Al(OH)3 (expressed in terms of the equivalent mass of Al2O3) in aqueous NaOH solutions as a function of temperature. (Adapted from: L.K. Hudson, Critical Reports on Applied Chemistry, 1987, 20 pp. 11-46.) 2.
- In the caustic process NaOH is regenerated. Leaching occurs as per the reactions above. The hot solution is allowed to cool (heat is recovered by heat exchangers for preheating feed to leaching) and Al(OH)3 precipitates from solution. (Prior purification processes are needed as well, especially to remove silica, i.e. SiO2, dissolved from clay minerals. However, the problematic step of iron removal is avoided.) This is just the reverse of reaction (13). This then regenerates the leaching reagent, which is absolutely essential due to its high cost.
In the acid process we could regenerate the acid as well. However, this poses greater difficulties. Due to the very high affinity of Al(III) for oxygen-containing ligands, the salt precipitated from HCl solution is [Al(H2O)6]Cl3. The final product of the process needs to be Al2O3, which is suitable as a feed to molten salt electrolysis to form Al metal at ~1000°C. At these temperatures, water must obviously be absent. In the Bayer process, Al(OH)3 is calcined:
[latex]2Al(OH)_{3}\:_{s}\:=Al_{2}O\:_{3}\:_{s}+3H_{2}0\;_{g}\;\;\;\;\;\Delta H^{\circ}=186\text{ kJ/mol} \tag{28}[/latex]
Calcination of [Al(H2O)6]Cl3 would require a lot more energy, since additional water would have to be driven off, as well as HCl:
[latex]2\:[Al(H_{2}0)_{6}]Cl_{3}\:=\:Al_{2}O_{3s}\:+\:6HCl_{g}\:+\:9H_{2}O_{g}\;\;\;\;\;\Delta H^{\circ}=959\text{ kJ/mol} \tag{29}[/latex]
Again, HCl can be recovered and reused, as with NaOH, but the higher energy costs and issues around iron removal from the leach solution are prohibitively expensive. The situation is worse for sulfate medium. Again a hydrated salt is formed, Al2(SO4)3·nH2O (n = 6 or ~18, depending on conditions). In addition to driving off water, H2SO4 g must be driven off in order to form Al2O3 again. Since sulfuric acid boils at ~340°C, the energy involved in forming H2SO4 g is much higher than forming HCl g; pure HCl is a gas at room temperature and pressure.
- In principle an acid leach solution can be neutralized with base to precipitate Al(OH)3. However, now all the acid is lost and base is consumed as well. This becomes very costly. In addition, at ordinary temperatures a gelatinous Al(OH)3·nH2O precipitate is formed that is almost impossible to filter.
- In the acid leaching and calcination systems strongly acidic solutions and vapours must be handled. These are much more corrosive toward steel than NaOH solutions. In fact, mild steel may be used in many parts of the Bayer process.
In summary, this is a case where the chemistry and conditions downstream from leaching determine the choice of leaching medium. This is a rare example of a situation where a base metal is produced using a basic solution.
Characteristics of an Ideal Leaching Process
When we are trying to leach a mineral of interest the following features are essential:
- The mineral must be soluble in an economically affordable solution.
- The metal must be economically recoverable from solution.
- Co-extracted impurities must be capable of further separation.
- Gangue minerals should not consume excessive amounts of reagents.
- Solid-liquid separation must be feasible.
The following features are desirable:
- The leaching reagents should be recoverable or able to be regenerated for recycling.
- The feed material should be free of clay minerals (clays often make separation of the leach solution from the residual solids difficult).
- The feed material should be porous to allow good contact between the leach solution and the desired minerals, providing a high surface area for reaction, and lessening the extent of size reduction needed for the feed material.
- The leach solution should not be corrosive to plant equipment and materials.
- The leach solution should be as non-toxic as possible.
Summary of Common Leaching Processes
Based on the preceding section, it should come as no surprise that there is not a great variety of leaching systems in practice. Some of the common processes are summarized in the table below. Note the preponderance of sulfuric acid leaching solutions (sulfate media). Some terms are explained here. Oxidized sulfides result oxides, hydroxides, carbonates, sulfates, silicates, etc. Zinc calcine is the product of roast ZnS, i.e. ZnO. A laterite is a weathered, near surface deposit. It has been in contact with surface water (and hence some O2 as well) for an extended time. Nickel laterites contain modest concentrations of nickel and roughly 1/10 as much cobalt. Laterites contain oxides, hydroxides and aluminosilicates. A reduced laterite (nickel-bearing) has been subjected to a reducing roast (e.g. by incomplete combustion of hydrocarbon) prior to leaching. Oxidized gold ore is an original sulfide ore that has undergone oxidation, e.g. naturally over geologic time. A refractory gold ore refers to a sulfide ore that contains finely disseminated gold, or has other factors that mitigate direct cyanidation. Scheelite, i.e. CaWO4, leaching by Na2CO3 is an example of metathesis (exchange). CaCO3 is less soluble than CaWO4, so that soluble Na2WO4 goes into solution. Technically it is leaching with base, but metathesis per se does not fit within the categories outlined in Figure 1.
Media Attributions
- Figure 5. Eh-pH diagram for the Au-H2O-CN
- Figure 6. Eh-pH diagram for the Au-H2O-Cl