Unit 5.2: The Art of Culturing Microbes
Outline
Learning Objectives
After reading the following, you should be able to:
- Describe the basic requirements for culturing bacteria in a laboratory setting.
- Explain why agar is used in culturing.
- Describe the types of media (chemically-defined, complex, mannitol salt, and blood agar) are used to culture bacteria.
- Describe the ways that viruses can be cultivated.
The study of microorganisms is greatly facilitated if we are able to culture them, that is, to keep reproducing populations alive under laboratory conditions. Culturing many microorganisms is challenging because of highly specific nutritional and environmental requirements and the diversity of these requirements among different species.
Nutritional Requirements: The number of available media to grow bacteria is considerable. Some media are considered general all-purpose media and support growth of a large variety of organisms. A prime example of an all-purpose medium is tryptic soy broth (TSB). Specialized media are used in the identification of bacteria and are supplemented with dyes, pH indicators, or antibiotics. When the complete chemical composition of a medium is known, it is called a chemically defined medium. For example, in EZ medium, all individual chemical components are identified and the exact amounts of each are known. In complex media, which contain extracts and digests of yeasts, meat, or plants, the precise chemical composition of the medium is not known. Amounts of individual components are undetermined and variable. Nutrient broth, tryptic soy broth, and brain heart infusion, are all examples of complex media.
Bacterial growth media can be a liquid or a solid. When solid media is desired at gelling agent is added to liquid media to cause it to solidify. For most application, the agent used is a carbohydrate derived from algae called agar. Agar has several properties that make it desirable for microbial media. First, very few microbes are able to metabolize agar as a nutrient source. This is crucial, as you may recall from the gelatinase test, if the gelling agent is digested, the media reverts back to a liquid. Agar liquefies at 100°C, which is conveniently the boiling temperature of water, making it easy to introduce into media, and solidifies at 40°C. This second temperature is also very important, as most microbes that we are interested in studying are human pathogens that grow optimally at body temperature, which is 37°C.
Selective and differential media: Media that inhibit the growth of unwanted microorganisms and support the growth of the organism of interest by supplying nutrients and reducing competition are called selective media. An example of a selective medium is MacConkey agar. It contains bile salts and crystal violet, which interfere with the growth of many gram-positive bacteria and favor the growth of gram-negative bacteria, particularly the Enterobacteriaceae. These species are commonly named enterics, reside in the intestine, and are adapted to the presence of bile salts. The differential media make it easy to distinguish colonies of different bacteria by a change in the color of the colonies or the color of the medium. Color changes are the result of end products created by interaction of bacterial enzymes with differential substrates in the medium or, in the case of hemolytic reactions, the lysis of red blood cells in the medium. In Figure 5.11, the differential fermentation of lactose can be observed on MacConkey agar. The lactose fermenters produce acid, which turns the medium and the colonies of strong fermenters hot pink. The medium is supplemented with the pH indicator neutral red, which turns to hot pink at low pH. Selective and differential media can be combined and play an important role in the identification of bacteria by biochemical methods.
Two other specific types of media that you will be using often in the labs are Mannitol Salt media and Blood Agar media. Like MacConkey agar, mannitol salt media is both selective and differential. It contains a high concentration of salt, so only halophilic or halotolerant organisms can grow on it. It also contains phenol red to detect the fermentation of the sugar mannitol. As discussed previously if the mannitol is fermented, acidic waste products will be produced, causing the media to become yellow (Figure 5.11) Blood agar media is differential only. It does not restrict which bacteria can grow on it. It is differential because it allows us to determine whether a bacteria is capable of causing hemolysis, which is the destruction of the red blood cells that have been added to the media. We can differentiate bacterial species based on their ability to cause, beta hemolysis (completely clearing), alpha hemolysis (partial clearing) or gamma hemolysis (no clearing) (Figure 5.12).
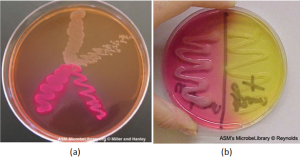
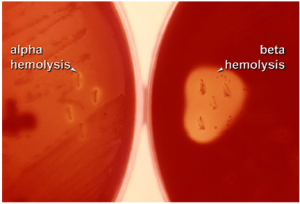
Other requirements for growth: Media alone is not sufficient to promote growth. While the media does take care of nutrient requirement, pH and osmotic pressure, it can’t help with requirements like oxygen or temperature. For temperature, most cultures are grown in incubators set to the microbe’s optimal growth temperature (37°C for most pathogens). When we want to slow down or stop microbial grow, we can place the microbes at colder temperature. This is often done is the laboratory by placing the bacterial samples in a refrigerator, which effectively stops the growth of most microbial pathogens. For the most part, putting bacteria does not harm them, so, as will be discussed more in the next unit, refrigerating, or even freezing bacteria, is an effective way of preserving them for later study.
In regards to oxygen, things can be more difficult. If the bacteria requires, or tolerates oxygen, then culturing is straightforward, as oxygen is prevalent in the atmosphere. When dealing with anaerobic bacteria, extra steps must be taken to allow them to grow properly. The most common approach is to culture in an anaerobic jar (Figure 5.13). Anaerobic jars include chemical packs that remove oxygen and release carbon dioxide (CO2). Another option is to us an anaerobic chamber, which is an enclosed box from which all oxygen is removed. Gloves sealed to openings in the box allow handling of the cultures without exposing the culture to air (Figure 5.13).
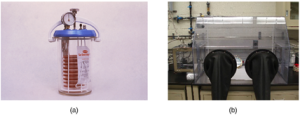
Cultivation of Viruses: Viruses can be grown in vivo (within a whole living organism, plant, or animal) or in vitro (outside a living organism in cells in an artificial environment, such as a test tube, cell culture flask, or agar plate). Bacteriophages can be grown in the presence of a dense layer of bacteria (also called a bacterial lawn) grown in a 0.7 % soft agar in a Petri dish or flat (horizontal) flask (see Figure 5.14). The agar concentration is decreased from the 1.5% usually used in culturing bacteria. The soft 0.7% agar allows the bacteriophages to easily diffuse through the medium. For lytic bacteriophages, lysing of the bacterial hosts can then be readily observed when a clear zone called a plaque is detected (see Figure 5.14). As the phage kills the bacteria, many plaques are observed among the cloudy bacterial lawn.
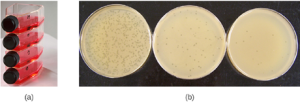
Animal viruses require cells within a host animal or tissue-culture cells derived from an animal. Animal virus cultivation is important for 1) identification and diagnosis of pathogenic viruses in clinical specimens, 2) production of vaccines, and 3) basic research studies. In vivo host sources can be a developing embryo in an embryonated bird’s egg (e.g., chicken, turkey) or a whole animal. For example, most of the influenza vaccine manufactured for annual flu vaccination programs is cultured in hens’ eggs.
The embryo or host animal serves as an incubator for viral replication (see Figure 5.15). Location within the embryo or host animal is important. Many viruses have a tissue tropism, and must therefore be introduced into a specific site for growth. Within an embryo, target sites include the amniotic cavity, the chorioallantoic membrane, or the yolk sac. Viral infection may damage tissue membranes, producing lesions called pox; disrupt embryonic development; or cause the death of the embryo.
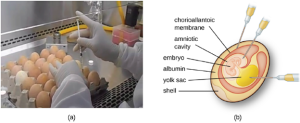
For in vitro studies, various types of cells can be used to support the growth of viruses. A primary cell culture is freshly prepared from animal organs or tissues. Cells are extracted from tissues by mechanical scraping or mincing to release cells or by an enzymatic method using trypsin or collagenase to break up tissue and release single cells into suspension. Because of anchorage-dependence requirements, primary cell cultures require a liquid culture medium in a Petri dish or tissue-culture flask so cells have a solid surface such as glass or plastic for attachment and growth. Viruses can be introduced into these cell cultures for growth. Cell cultures allow for specificity, as you select the types of cells to infect, and control, as you determine the exact culture conditions.