Unit 9.5: Diseases of the Nervous System
Outline
Part 1: Anatomy of the Nervous System
- The Central Nervous System
- The Blood-Brain Barrier
- The Peripheral Nervous System
- The Cells of the Nervous System
Part 2: Bacterial Diseases of the Nervous System
Learning Objectives
After reading the following, you should be able to:
- Describe the basic structure of the nervous system, including protective features and defenses.
- Describe any general features of infections of the nervous system.
- Describe meningitis, including its causative organisms and possible complications.
- Compare and contrast the different causative organisms
- Describe tetanus, including its causative organism and possible complications.
- Describe botulism, including its causative organism and possible complications.
- Describe rabies, including its causative organism and possible complications.
- For all the diseases in this section, describe mode of transmission, portal of entry, modes of detection (where discussed) and treatments (in general, e.g. antiviral medication, vaccine, etc)
Few diseases inspire the kind of fear that rabies does. The name is derived from the Latin word for “madness” or “fury,” most likely because animals infected with rabies may behave with uncharacteristic rage and aggression. And while the thought of being attacked by a rabid animal is terrifying enough, the disease itself is even more frightful. Once symptoms appear, the disease is almost always fatal, even when treated.
Rabies is an example of a neurological disease caused by an acellular pathogen. The rabies virus enters nervous tissue shortly after transmission and makes its way to the central nervous system, where its presence leads to changes in behavior and motor function. Well-known symptoms associated with rabid animals include foaming at the mouth, hydrophobia (fear of water), and unusually aggressive behavior. Rabies claims tens of thousands of human lives worldwide, mainly in Africa and Asia. Most human cases result from dog bites, although many mammal species can become infected and transmit the disease. Human infection rates are low in the United States and many other countries as a result of control measures in animal populations. However, rabies is not the only disease with serious or fatal neurological effects. In this section, we examine the important microbial diseases of the nervous system.
Part 1: Anatomy of the Nervous System
The human nervous system can be divided into two interacting subsystems: the peripheral nervous system (PNS) and the central nervous system (CNS). The CNS consists of the brain and spinal cord. The peripheral nervous system is an extensive network of nerves connecting the CNS to the muscles and sensory structures. The relationship of these systems is illustrated in Figure 9.42.
The Central Nervous System: The brain is the most complex and sensitive organ in the body. It is responsible for all functions of the body, including serving as the coordinating center for all sensations, mobility, emotions, and intellect. Protection for the brain is provided by the bones of the skull, which in turn are covered by the scalp, as shown in Figure 9.43. The scalp is composed of an outer layer of skin, which is loosely attached to the aponeurosis, a flat, broad tendon layer that anchors the superficial layers of the skin. The periosteum, below the aponeurosis, firmly encases the bones of the skull and provides protection, nutrition to the bone, and the capacity for bone repair. Below the boney layer of the skull are three layers of membranes called meninges that surround the brain. The relative positions of these meninges are shown in Figure 9.43. The meningeal layer closest to the bones of the skull is called the dura mater (literally meaning tough mother). Below the dura mater lies the arachnoid mater (literally spider-like mother). The innermost meningeal layer is a delicate membrane called the pia mater (literally tender mother). Unlike the other meningeal layers, the pia mater firmly adheres to the convoluted surface of the brain. Between the arachnoid mater and pia mater is the subarachnoid space. The subarachnoid space within this region is filled with cerebrospinal fluid (CSF). This watery fluid is produced by cells of the choroid plexus—areas in each ventricle of the brain that consist of cuboidal epithelial cells surrounding dense capillary beds. The CSF serves to deliver nutrients and remove waste from neural tissues.
The Blood-Brain Barrier: The tissues of the CNS have extra protection in that they are not exposed to blood or the immune system in the same way as other tissues. The blood vessels that supply the brain with nutrients and other chemical substances lie on top of the pia mater. The capillaries associated with these blood vessels in the brain are less permeable than those in other locations in the body. The capillary endothelial cells form tight junctions that control the transfer of blood components to the brain. In addition, cranial capillaries have far fewer fenestra (pore-like structures that are sealed by a membrane) and pinocytotic vesicles than other capillaries. As a result, materials in the circulatory system have a very limited ability to interact with the CNS directly. This phenomenon is referred to as the blood-brain barrier.
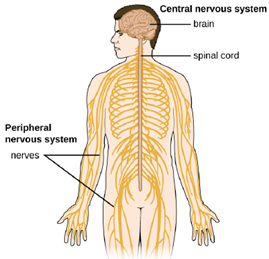
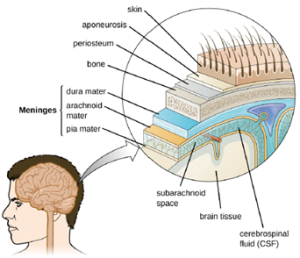
The blood-brain barrier protects the cerebrospinal fluid from contamination, and can be quite effective at excluding potential microbial pathogens. As a consequence of these defenses, there is no normal microbiota in the cerebrospinal fluid. The blood-brain barrier also inhibits the movement of many drugs into the brain, particularly compounds that are not lipid soluble. This has profound ramifications for treatments involving infections of the CNS, because it is difficult for drugs to cross the blood-brain barrier to interact with pathogens that cause infections.
The spinal cord also has protective structures similar to those surrounding the brain. Within the bones of the vertebrae are meninges of dura mater (sometimes called the dural sheath), arachnoid mater, pia mater, and a blood-spinal cord barrier that controls the transfer of blood components from blood vessels associated with the spinal cord. To cause an infection in the CNS, pathogens must successfully breach the blood-brain barrier or blood-spinal cord barrier.
The Peripheral Nervous System: The PNS is formed of the nerves that connect organs, limbs, and other anatomic structures of the body to the brain and spinal cord. Unlike the brain and spinal cord, the PNS is not protected by bone, meninges, or a blood barrier, and, as a consequence, the nerves of the PNS are much more susceptible to injury and infection. Microbial damage to peripheral nerves can lead to tingling or numbness known as neuropathy. These symptoms can also be produced by trauma and noninfectious causes such as drugs or chronic diseases like diabetes.
The Cells of the Nervous System:
Tissues of the PNS and CNS are formed of cells called glial cells (neuroglial cells) and neurons (nerve cells). Glial cells assist in the organization of neurons, provide a scaffold for some aspects of neuronal function, and aid in recovery from neural injury.
Neurons are specialized cells found throughout the nervous system that transmit signals through the nervous system using electrochemical processes. The basic structure of a neuron is shown in Figure 9.44. The cell body (or soma) is the metabolic center of the neuron and contains the nucleus and most of the cell’s organelles. The many finely branched extensions from the soma are called dendrites. The soma also produces an elongated extension, called the axon, which is responsible for the transmission of electrochemical signals through elaborate ion transport processes. Axons of some types of neurons can extend up to one meter in length in the human body. To facilitate electrochemical signal transmission, some neurons have a myelin sheath surrounding the axon. Myelin, formed from the cell membranes of glial cells like the Schwann cells in the PNS and oligodendrocytes in the CNS, surrounds and insulates the axon, significantly increasing the speed of electrochemical signal transmission along the axon. The end of an axon forms numerous branches that end in bulbs called synaptic terminals. Neurons form junctions with other cells, such as another neuron, with which they exchange signals. The junctions, which are actually gaps between neurons, are referred to as synapses. At each synapse, there is a presynaptic neuron and a postsynaptic neuron (or other cell). The synaptic terminals of the axon of the presynaptic terminal form the synapse with the dendrites, soma, or sometimes the axon of the postsynaptic neuron, or a part of another type of cell such as a muscle cell. The synaptic terminals contain vesicles filled with chemicals called neurotransmitters. When the electrochemical signal moving down the axon reaches the synapse, the vesicles fuse with the membrane, and neurotransmitters are released, which diffuse across the synapse and bind to receptors on the membrane of the postsynaptic cell, potentially initiating a response in that cell. That response in the postsynaptic cell might include further propagation of an electrochemical signal to transmit information or contraction of a muscle fiber.
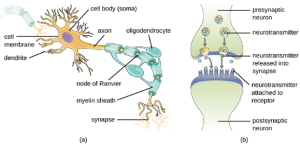
Meningitis and Encephalitis: Although the skull provides the brain with an excellent defense, it can also become problematic during infections. Any swelling of the brain or meninges that results from inflammation can cause intracranial pressure, leading to severe damage of the brain tissues, which have limited space to expand within the inflexible bones of the skull. The term meningitis is used to describe an inflammation of the meninges. Typical symptoms can include severe headache, fever, photophobia (increased sensitivity to light), stiff neck, convulsions, and confusion. An inflammation of brain tissue is called encephalitis, and patients exhibit signs and symptoms similar to those of meningitis in addition to lethargy, seizures, and personality changes. When inflammation affects both the meninges and the brain tissue, the condition is called meningoencephalitis. All three forms of inflammation are serious and can lead to blindness, deafness, coma, and death.
Meningitis and encephalitis can be caused by many different types of microbial pathogens. However, these conditions can also arise from noninfectious causes such as head trauma, some cancers, and certain drugs that trigger inflammation. To determine whether the inflammation is caused by a pathogen, a lumbar puncture is performed to obtain a sample of CSF. If the CSF contains increased levels of white blood cells and abnormal glucose and protein levels, this indicates that the inflammation is a response to an infection.
Part 2: Bacterial Diseases of the Nervous System
Bacterial infections that affect the nervous system are serious and can be life-threatening. Fortunately, there are only a few bacterial species commonly associated with neurological infections.
Bacterial Meningitis: Bacterial meningitis is one of the most serious forms of meningitis. Bacteria that cause meningitis often gain access to the CNS through the bloodstream after trauma or as a result of the action of bacterial toxins. Bacteria may also spread from structures in the upper respiratory tract, such as the oropharynx, nasopharynx, sinuses, and middle ear. Patients with head wounds or cochlear implants (an electronic device placed in the inner ear) are also at risk for developing meningitis.
Many of the bacteria that can cause meningitis are commonly found in healthy people. The most common causes of non-neonatal bacterial meningitis are Neisseria meningitidis, Streptococcus pneumoniae, and Haemophilus influenzae. All three of these bacterial pathogens are spread from person to person by respiratory secretions. Each can colonize and cross through the mucous membranes of the oropharynx and nasopharynx, and enter the blood. Once in the blood, these pathogens can disseminate throughout the body and are capable of both establishing an infection and triggering inflammation in any body site, including the meninges (Figure 9.45). Without appropriate systemic antibacterial therapy, the case-fatality rate can be as high as 70%, and 20% of those survivors may be left with irreversible nerve damage or tissue destruction, resulting in hearing loss, neurologic disability, or loss of a limb.
Mortality rates are much lower (as low as 15%) in populations where appropriate therapeutic drugs and preventive vaccines are available.
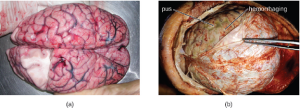
The profound inflammation caused by these microbes can result in early symptoms that include severe headache, fever, confusion, nausea, vomiting, photophobia, and stiff neck. Systemic inflammatory responses associated with some types of bacterial meningitis can lead to hemorrhaging and purpuric lesions on skin, followed by even more severe conditions that include shock, convulsions, coma, and death—in some cases, in the span of just a few hours. Diagnosis of bacterial meningitis is best confirmed by analysis of CSF obtained by a lumbar puncture. Characteristics of specific forms of bacterial meningitis are detailed in the subsections that follow.
- Meningococcal Meningitis: Meningococcal meningitis is a serious infection caused by the gram-negative coccus N. meningitidis. In some cases, death can occur within a few hours of the onset of symptoms. Nonfatal cases can result in irreversible nerve damage, resulting in hearing loss and brain damage, or amputation of extremities because of tissue necrosis.
Meningococcal meningitis can infect people of any age, but its prevalence is highest among infants, adolescents, and young adults. Meningococcal meningitis was once the most common cause of meningitis epidemics in human populations. This is still the case in a swath of sub-Saharan Africa known as the meningitis belt, but meningococcal meningitis epidemics have become rare in most other regions, thanks to meningococcal vaccines. However, outbreaks can still occur in communities, schools, colleges, prisons, and other populations where people are in close direct contact.
N. meningitidis has a high affinity for mucosal membranes in the oropharynx and nasopharynx. Contact with respiratory secretions containing N. meningitidis is an effective mode of transmission. The pathogenicity of N. meningitidis is enhanced by virulence factors that contribute to the rapid progression of the disease. These include lipooligosaccharide (LOS) endotoxin, type IV pili for attachment to host tissues, and polysaccharide capsules that help the cells avoid phagocytosis and complement-mediated killing. Additional virulence factors include IgA protease (which breaks down IgA antibodies), the invasion factors Opa, Opc, and porin (which facilitate transcellular entry through the blood-brain barrier), iron-uptake factors (which strip heme units from hemoglobin in host cells and use them for growth), and stress proteins that protect bacteria from reactive oxygen molecules.
A unique sign of meningococcal meningitis is the formation of a petechial rash on the skin or mucous membranes, characterized by tiny, red, flat, hemorrhagic lesions. This rash, which appears soon after disease onset, is a response to LOS endotoxin and adherence virulence factors that disrupt the endothelial cells of capillaries and small veins in the skin. The blood vessel disruption triggers the formation of tiny blood clots, causing blood to leak into the surrounding tissue. As the infection progresses, the levels of virulence factors increase, and the hemorrhagic lesions can increase in size as blood continues to leak into tissues. Lesions larger than 1.0 cm usually occur in patients developing shock, as virulence factors cause increased hemorrhage and clot formation. Sepsis, as a result of systemic damage from meningococcal virulence factors, can lead to rapid multiple organ failure, shock, disseminated intravascular coagulation, and death.
Prompt presumptive diagnosis of meningococcal meningitis can occur when CSF is directly evaluated by Gram stain, revealing extra- and intracellular gram-negative diplococci with a distinctive coffee-bean microscopic morphology associated with PMNs (Figure 9.46). Meningococcal infections can be treated with antibiotic therapy, and third-generation cephalosporins are most often employed. However, because outcomes can be negative even with treatment, preventive vaccination is the best form of treatment. In 2010, countries in Africa’s meningitis belt began using a new serogroup A meningococcal conjugate vaccine. This program has dramatically reduced the number of cases of meningococcal meningitis by conferring individual and herd immunity.
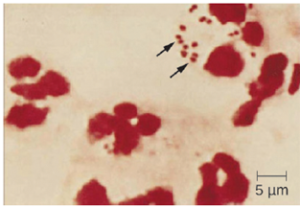
2. Pneumococcal Meningitis: Pneumococcal meningitis is caused by the encapsulated gram-positive bacterium S. pneumoniae (pneumococcus, also called strep pneumo). This organism is commonly found in the microbiota of the pharynx of 30–70% of young children, depending on the sampling method, while S. pneumoniae can be found in fewer than 5% of healthy adults. Although it is often present without disease symptoms, this microbe can cross the blood-brain barrier in susceptible individuals. In some cases, it may also result in septicemia. Since the introduction of the Hib vaccine, S. pneumoniae has become the leading cause of meningitis in humans aged 2 months through adulthood.
S. pneumoniae can be identified in CSF samples using gram-stained specimens. In gram-stained samples, S. pneumoniae appears as gram-positive, lancet-shaped diplococci (Figure 9.47). Major virulence factors produced by S. pneumoniae include PI-1 pilin for adherence to host cells (pneumococcal adherence) and virulence factor B (PavB) for attachment to cells of the respiratory tract; choline-binding proteins
(cbpA) that bind to epithelial cells and interfere with immune factors IgA and C3; and the cytoplasmic bacterial toxin pneumolysin that triggers an inflammatory response.
With the emergence of drug-resistant strains of S. pneumoniae, pneumococcal meningitis is typically treated with broad-spectrum antibiotics and preventative vaccines are available.
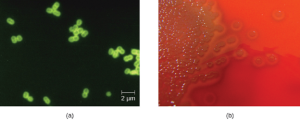
- Haemophilus influenzae Type b: Meningitis due to H. influenzae serotype b (Hib), an encapsulated pleomorphic gram-negative coccobacilli, is now uncommon in most countries, because of the use of the effective Hib vaccine. Without the use of the Hib vaccine, H. influenzae can be the primary cause of meningitis in children 2 months thru 5 years of age. H. influenzae can be found in the throats of healthy individuals, including infants and young children. By five years of age, most children have developed immunity to this microbe. Infants older than 2 months of age, however, do not produce a sufficient protective antibody response and are susceptible to serious disease. The intracranial pressure caused by this infection leads to a 5% mortality rate and 20% incidence of deafness or brain damage in survivors.
H. influenzae produces at least 16 different virulence factors, including LOS, which triggers inflammation, and Haemophilus adhesion and penetration factor (Hap), which aids in attachment and invasion into respiratory epithelial cells. The bacterium also has a polysaccharide capsule that helps it avoid phagocytosis, as well as factors such as IgA1 protease and P2 protein that allow it to evade antibodies secreted from mucous membranes. In addition, factors such as hemoglobin-binding protein (Hgp) and transferrin-binding protein (Tbp) acquire iron from hemoglobin and transferrin, respectively, for bacterial growth.
Meningitis caused by H. influenzae is usually treated with doxycycline, fluoroquinolones, second- and third-generation cephalosporins, and carbapenems. The best means of preventing H. influenza infection is with the use of the Hib polysaccharide conjugate vaccine. It is recommended that all children receive this vaccine at 2, 4, and 6 months of age, with a final booster dose at 12 to 15 months of age.
Tetanus: Tetanus is a noncommunicable disease characterized by uncontrollable muscle spasms (contractions) caused by the action of an exotoxin called tetanospasmin. It generally occurs when C. tetani infects a wound and produces tetanospasmin, which rapidly binds to neural tissue, resulting in an intoxication (poisoning) of neurons. Depending on the site and extent of infection, cases of tetanus can be described as localized, cephalic, or generalized. Generalized tetanus that occurs in a newborn is called neonatal tetanus.
Localized tetanus occurs when tetanospasmin only affects the muscle groups close to the injury site. There is no CNS involvement, and the symptoms are usually mild, with localized muscle spasms caused by a dysfunction in the surrounding neurons. Individuals with partial immunity—especially previously vaccinated individuals who neglect to get the recommended booster shots—are most likely to develop localized tetanus as a result of C. tetani infecting a puncture wound.
Both localized and cephalic tetanus may progress to generalized tetanus—a much more serious condition—if Tetanospasmin is able to spread further into body tissues. In generalized tetanus, tetanospasmin enters neurons of the PNS. From there, tetanospasmin travels from the site of the wound, usually on an extremity of the body, retrograde (back up) to inhibitory neurons in the CNS. There, it prevents the release of gamma aminobutyric acid (GABA), the neurotransmitter responsible for muscle relaxation. The resulting muscle spasms often first occur in the jaw muscles, leading to the characteristic symptom of lockjaw (inability to open the mouth). As the toxin progressively continues to block neurotransmitter release, other muscles become involved, resulting in uncontrollable, sudden muscle spasms that are powerful enough to cause tendons to rupture and bones to fracture. Spasms in the muscles in the neck, back, and legs may cause the body to form a rigid, stiff arch, a posture called opisthotonos (Figure 9.48). Spasms in the larynx, diaphragm, and muscles of the chest restrict the patient’s ability to swallow and breathe, eventually leading to death by asphyxiation (insufficient supply of oxygen).
Neonatal tetanus typically occurs when the stump of the umbilical cord is contaminated with spores of C. tetani after delivery. Although this condition is rare in the United States, neonatal tetanus is a major cause of infant mortality in countries that lack maternal immunization for tetanus and where birth often occurs in unsanitary conditions. At the end of the first week of life, infected infants become irritable, feed poorly, and develop rigidity with spasms. Neonatal tetanus has a very poor prognosis with a mortality rate of 70%–100%.
Treatment for patients with tetanus includes assisted breathing through the use of a ventilator, wound debridement, fluid balance, and antibiotic therapy with metronidazole or penicillin to halt the growth of C. tetani. In addition, patients are treated with tetanospasmin antitoxin, preferably in the form of human immunoglobulin to neutralize nonfixed toxin and benzodiazepines to enhance the effect of GABA for muscle relaxation and anxiety.
A tetanus toxoid (TT) vaccine is available for protection and prevention of tetanus. It is the T component of vaccines such as DTaP, Tdap, and Td. The CDC recommends children receive doses of the DTaP vaccine at 2, 4, 6, and 15–18 months of age and another at 4–6 years of age. One dose of Td is recommended for adolescents and adults as a TT booster every 10 years.
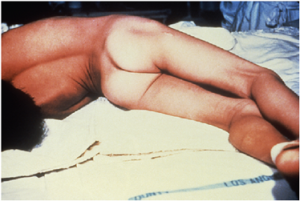
Botulism: Botulism is a rare but frequently fatal illness caused by intoxication by botulism toxin. It can occur either as the result of an infection by C. botulinum, in which case the bacteria produce botulism toxin in vivo, or as the result of a direct introduction of botulism toxin into tissues.
Infection and production of botulism toxin in vivo can result in wound botulism, infant botulism, and adult intestinal toxemia. Wound botulism typically occurs when C. botulinum is introduced directly into a wound after a traumatic injury, deep puncture wound, or injection site. Infant botulism, which occurs in infants younger than 1 year of age, and adult intestinal toxemia, which occurs in immunocompromised adults, results from ingesting C. botulinum endospores in food. The endospores germinate in the body, resulting in the production of botulism toxin in the intestinal tract.
Intoxications occur when botulism toxin is produced outside the body and then introduced directly into the body through food (foodborne botulism), air (inhalation botulism), or a clinical procedure (iatrogenic botulism). Foodborne botulism, the most common of these forms, occurs when botulism toxin is produced in contaminated food and then ingested along with the food.
When botulism toxin enters the bloodstream in the gastrointestinal tract, wound, or lungs, it is transferred to the neuromuscular junctions of motor neurons where it binds irreversibly to presynaptic membranes and prevents the release of acetylcholine from the presynaptic terminal of motor neurons into the neuromuscular junction. The consequence of preventing acetylcholine release is the loss of muscle activity, leading to muscle relaxation and eventually paralysis.
If botulism toxin is absorbed through the gastrointestinal tract, early symptoms of botulism include blurred vision, drooping eyelids, difficulty swallowing, abdominal cramps, nausea, vomiting, constipation, or possibly diarrhea. This is followed by progressive flaccid paralysis, a gradual weakening and loss of control over the muscles. A patient’s experience can be particularly terrifying, because hearing remains normal, consciousness is not lost, and he or she is fully aware of the progression of his or her condition. In infants, notable signs of botulism include weak cry, decreased ability to suckle, and hypotonia (limpness of head or body). Eventually, botulism ends in death from respiratory failure caused by the progressive paralysis of the muscles of the upper airway, diaphragm, and chest.
Botulism is treated with an antitoxin specific for botulism toxin. If administered in time, the antitoxin stops the progression of paralysis but does not reverse it. Once the antitoxin has been administered, the patient will slowly regain neurological function, but this may take several weeks or months, depending on the severity of the case. During recovery, patients generally must remain hospitalized and receive breathing assistance through a ventilator.
Although it is the most toxic biological material known to man, botulinum toxin is often intentionally injected into people to treat other conditions. Type A botulinum toxin is used cosmetically to reduce wrinkles. The injection of minute quantities of this toxin into the face causes the relaxation of facial muscles, thereby giving the skin a smoother appearance. Eyelid twitching and crossed eyes can also be treated with botulinum toxin injections. Other uses of this toxin include the treatment of hyperhidrosis (excessive sweating). In fact, botulinum toxin can be used to moderate the effects of several other apparently nonmicrobial diseases involving inappropriate nerve function. Such diseases include cerebral palsy, multiple sclerosis, and Parkinson’s disease. Each of these diseases is characterized by a loss of control over muscle contractions; treatment with botulinum toxin serves to relax contracted muscles.
Part 3: Viral Diseases of the Nervous System
A number of different viruses and subviral particles can cause diseases that affect the nervous system. Viral diseases tend to be more common than bacterial infections of the nervous system today. Fortunately, viral infections are generally milder than their bacterial counterparts and often spontaneously resolve. Some of the more important acellular pathogens of the nervous system are described in this section.
Viral Meningitis: Although it is much more common than bacterial meningitis, viral meningitis is typically less severe. Many different viruses can lead to meningitis as a sequela of the primary infection, including those that cause herpes, influenza, measles, and mumps. Most cases of viral meningitis spontaneously resolve, but severe cases do occur.
Rabies is a deadly zoonotic disease that has been known since antiquity. The disease is caused by rabies virus (RV), a member of the family Rhabdoviridae, and is primarily transmitted through the bite of an infected mammal. Rhabdoviridae are enveloped RNA viruses that have a distinctive bullet shape (Figure 9.48); they were first studied by Louis Pasteur, who obtained rabies virus from rabid dogs and cultivated the virus in rabbits. He successfully prepared a rabies vaccine using dried nerve tissues from infected animals. This vaccine was used to first treat an infected human in 1885.
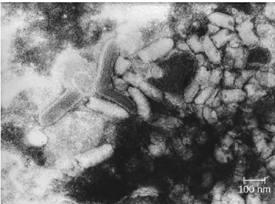
The most common reservoirs in the United States are wild animals such as raccoons (30.2% of all animal cases during 2014), bats (29.1%), skunks (26.3%), and foxes (4.1%); collectively, these animals were responsible for a total of 92.6% of animal rabies cases in the United States in 2014. The remaining 7.4% of cases that year were in domesticated animals such as dogs, cats, horses, mules, sheep, goats, and llamas. While there are typically only one or two human cases per year in the United States, rabies still causes tens of thousands of human deaths per year worldwide, primarily in Asia and Africa.
The low incidence of rabies in the United States is primarily a result of the widespread vaccination of dogs and cats. An oral vaccine is also used to protect wild animals, such as raccoons and foxes, from infection. Oral vaccine programs tend to focus on geographic areas where rabies is endemic. The oral vaccine is usually delivered in a package of bait that is dropped by airplane, although baiting in urban areas is done by hand to maximize safety. Many countries require a quarantine or proof of rabies vaccination for domestic pets being brought into the country. These procedures are especially strict in island nations where rabies is not yet present, such as Australia.
The incubation period for rabies can be lengthy, ranging from several weeks or months to over a year. As the virus replicates, it moves from the site of the bite into motor and sensory axons of peripheral nerves and spreads from nerve to nerve using a process called retrograde transport, eventually making its way to the CNS through the spinal ganglia. Once rabies virus reaches the brain, the infection leads to encephalitis caused by the disruption of normal neurotransmitter function, resulting in the symptoms associated with rabies. The virions act in the synaptic spaces as competitors with a variety of neurotransmitters for acetylcholine, GABA, and glycine receptors. Thus, the action of rabies virus is neurotoxic rather than cytotoxic. After the rabies virus infects the brain, it can continue to spread through other neuronal pathways, traveling out of the CNS to tissues such as the salivary glands, where the virus can be released. As a result, as the disease progresses the virus can be found in many other tissues, including the salivary glands, taste buds, nasal cavity, and tears.
The early symptoms of rabies include discomfort at the site of the bite, fever, and headache. Once the virus reaches the brain and later symptoms appear, the disease is always fatal. Terminal rabies cases can end in one of two ways: either furious or paralytic rabies. Individuals with furious rabies become very agitated and hyperactive. Hydrophobia (a fear of water) is common in patients with furious rabies, which is caused by muscular spasms in the throat when swallowing or thinking about water. Excess salivation and a desire to bite can lead to foaming of the mouth. These behaviors serve to enhance the likelihood of viral transmission, although contact with infected secretions like saliva or tears alone is sufficient for infection. The disease culminates after just a few days with terror and confusion, followed by cardiovascular and respiratory arrest. In contrast, individuals with paralytic rabies generally follow a longer course of disease. The muscles at the site of infection become paralyzed. Over a period of time, the paralysis slowly spreads throughout the body. This paralytic form of disease culminates in coma and death.
Human rabies infections are treated by immunization with multiple doses of an attenuated vaccine to develop active immunity in the patient. Vaccination of an already-infected individual has the potential to work because of the slow progress of the disease, which allows time for the patient’s immune system to develop antibodies against the virus. Patients may also be treated with human rabies immune globulin (antibodies to the rabies virus) to encourage passive immunity. These antibodies will neutralize any free viral particles. Although the rabies infection progresses slowly in peripheral tissues, patients are not normally able to mount a protective immune response on their own.
Part 4: Fungal and Parasitic Diseases of the Nervous System
Fungal infections of the nervous system, called neuromycoses, are rare in healthy individuals. However, neuromycoses can be devastating in immunocompromised or elderly patients. Several eukaryotic parasites are also capable of infecting the nervous system of human hosts. Although relatively uncommon, these infections can also be life-threatening in immunocompromised individuals. In this section, we will first discuss neuromycoses, followed by parasitic infections of the nervous system.
Cryptococcocal Meningitis: Cryptococcus neoformans is a fungal pathogen that can cause meningitis. This yeast is commonly found in soils and is particularly associated with pigeon droppings. It has a thick capsule that serves as an important virulence factor, inhibiting clearance by phagocytosis. Most C. neoformans cases result in subclinical respiratory infections that, in healthy individuals, generally resolve spontaneously with no long-term consequences. In immunocompromised patients or those with other underlying illnesses, the infection can progress to cause meningitis and granuloma formation in brain tissues. Cryptococcus antigens can also serve to inhibit cell-mediated immunity and delayed-type hypersensitivity.
Cryptococcus can be easily cultured in the laboratory and identified based on its extensive capsule (Figure 9.50). C. neoformans is frequently cultured from urine samples of patients with disseminated infections. Prolonged treatment with antifungal drugs is required to treat cryptococcal infections. Many antifungal drugs have difficulty crossing the blood-brain barrier and have strong side effects that necessitate low doses; these factors contribute to the lengthy time of treatment. Patients with AIDS are particularly susceptible to Cryptococcus infections because of their compromised immune state. AIDS patients with cryptococcosis can also be treated with antifungal drugs, but they often have relapses; lifelong doses of fluconazole may be necessary to prevent reinfection.
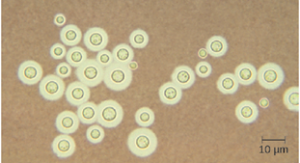
Amoebic Meningitis: Primary amoebic meningoencephalitis (PAM) is caused by Naegleria fowleri. This amoeboflagellate is commonly found free-living in soils and water. It can exist in one of three forms—the infective amoebic trophozoite form, a motile flagellate form, and a resting cyst form. PAM is a rare disease that has been associated with young and otherwise healthy individuals. Individuals are typically infected by the amoeba while swimming in warm bodies of freshwater such as rivers, lakes, and hot springs. The pathogenic trophozoite infects the brain by initially entering through nasal passages to the sinuses; it then moves down olfactory nerve fibers to penetrate the submucosal nervous plexus, invades the cribriform plate, and reaches the subarachnoid space. The subarachnoid space is highly vascularized and is a route of dissemination of trophozoites to other areas of the CNS, including the brain (Figure 9.51). Inflammation and destruction of gray matter leads to severe headaches and fever. Within days, confusion and convulsions occur and quickly progress to seizures, coma, and death. The progression can be very rapid, and the disease is often not diagnosed until autopsy.
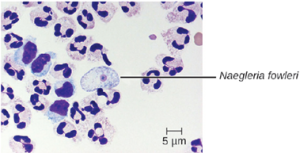
N. fowleri infections can be confirmed by direct observation of CSF; the amoebae can often be seen moving while viewing a fresh CSF wet mount through a microscope. N. fowleri infections are nearly always fatal; only 3 of 138 patients with PAM in the United States have survived. A new experimental drug called miltefosine shows some promise for treating these infections. This drug is a phosphotidylcholine derivative that is thought to inhibit membrane function in N. fowleri, triggering apoptosis and disturbance of lipid-dependent cell signaling pathways. When administered early in infection and coupled with therapeutic hypothermia (lowering the body’s core temperature to reduce the cerebral edema associated with infection), this drug has been successfully used to treat primary amoebic encephalitis.