Unit 1.3 A Brief History of Microbiology
Learning Objectives
After reading this section you should be able to:
- Explain: cell theory, aseptic technique, spontaneous generation, biogenesis, the germ theory of disease, pasteurization and ‘magic bullet”
- Describe the major contributions of Robert Hooke, Anton van Leeuwenhoek, Joseph Lister, Rudolf Virchow, Robert Remak, Ignaz Semmelweis, Robert Koch, Paul Ehrlich, Alexander Fleming and Edward Jenner.
- Describe the experiments and conclusions of Francisco Redi, John Needham, Lazzaro Spallanzani and Louis Pasteur regarding the debate over spontaneous generation.
Several ancient civilizations appear to have had some understanding that disease could be transmitted by things they could not see. The ancient Greeks attributed disease to bad air, mal’aria, which they called “miasmatic odors.” They developed hygiene practices that built on this idea. The Romans also believed in the miasma hypothesis and created a complex sanitation infrastructure to deal with sewage. In Rome, they built aqueducts, which brought fresh water into the city, and a giant sewer, the Cloaca Maxima, which carried waste away and into the river Tiber. Some researchers believe that this infrastructure helped protect the Romans from epidemics of waterborne illnesses.
The Greek physician Hippocrates (460–370 BC) is considered the “father of Western medicine” (Figure 1.9). Unlike many of his ancestors and contemporaries, he dismissed the idea that disease was caused by supernatural forces. Instead, he posited that diseases had natural causes from within patients or their environments. Hippocrates and his heirs are believed to have written the Hippocratic Corpus, a collection of texts that make up some of the oldest surviving medical books. Hippocrates is also often credited as the author of the Hippocratic Oath, taken by new physicians to pledge their dedication to diagnosing and treating patients without causing harm.
While Hippocrates is considered the father of Western medicine, the Greek philosopher and historian Thucydides (460–395 BC) is considered the father of scientific history because he advocated for evidence-based analysis of cause-and-effect reasoning (Figure 1.10). Among his most important contributions are his observations regarding the Athenian plague that killed one-third of the population of Athens between 430 and 410 BC. Having survived the epidemic himself, Thucydides made the important observation that survivors did not get re-infected with the disease, even when taking care of actively sick people. This observation shows an early understanding of the concept of immunity.
Marcus Terentius Varro (116–27 BC) was a prolific Roman writer who was one of the first people to propose the concept that things we cannot see (what we now call microorganisms) can cause disease (Figure 1.10). In Res Rusticae (On Farming), published in 36 BC, he said that “precautions must also be taken in neighborhood swamps. . . because certain minute creatures [animalia minuta] grow there which cannot be seen by the eye, which float in the air and enter the body through the mouth and nose and there cause serious diseases.”
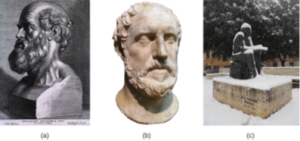
While the ancients may have suspected the existence of invisible “minute creatures,” it wasn’t until the invention of the microscope that their existence was definitively confirmed. Anton van Leeuwenhoek, sometimes hailed as “the Father of Microbiology,” is typically credited as the first person to have created microscopes powerful enough to view microbes (Figure 1.11). In 1674, he described his observations of single-celled organisms, whose existence was previously unknown, in a series of letters to the Royal Society of London. His report was initially met with skepticism, but his claims were soon verified and he became something of a celebrity in the scientific community.
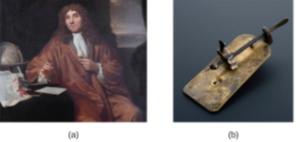
While van Leeuwenhoek is credited with the discovery of microorganisms, others before him had contributed to the nascent field of microbiology. Van Leeuwenhoek’s contemporary, the Englishman Robert Hooke (1635–1703), also made important contributions to microscopy, publishing in his book Micrographia (1665) many observations using compound microscopes. Viewing a thin sample of cork through his microscope, he was the first to observe the structures that we now know as cells (Figure 1.12). Hooke described these structures as resembling “Honey-comb,” and as “small Boxes or Bladders of Air,” noting that each “Cavern, Bubble, or Cell” is distinct from the others (in Latin, “cell” literally means “small room” and is still used in modern English, e.g. jail cell). They likely appeared to Hooke to be filled with air because the cork cells were dead, with only the rigid cell walls providing the structure.
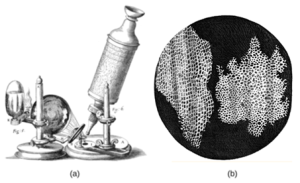
The Debate Over Spontaneous Generation:
With the discovery of this hidden, microscopic world, all around them, scientist turned their focus to where these “animalcules” were coming from. The Greek philosopher Aristotle (384–322 BC) was one of the earliest recorded scholars to articulate the theory of spontaneous generation, the notion that life can arise from nonliving matter. Aristotle proposed that life arose from nonliving material if the material contained pneuma (“vital heat”). As evidence, he noted several instances of the appearance of animals from environments previously devoid of such animals, such as the seemingly sudden appearance of fish in a new puddle of water. This theory persisted into the 17th century, when scientists undertook additional experimentation to support or disprove it. By this time, the proponents of the theory cited how frogs simply seem to appear along the muddy banks of the Nile River in Egypt during the annual flooding. Others observed that mice simply appeared among grain stored in barns with thatched roofs.
Italian physician Francesco Redi (1626–1697), performed an experiment in 1668 that was one of the first to refute the idea that maggots (the larvae of flies) spontaneously generate on meat left out in the open air. He predicted that preventing flies from having direct contact with the meat would also prevent the appearance of maggots. Redi left meat in set of containers, so of which were open to the air and others that were tightly sealed (Figure 1.13). His hypothesis was supported when maggots developed on the meat in the uncovered jars, but no maggots appeared on the meat in the tightly sealed jars. He concluded that maggots could only form when flies were allowed to lay eggs in the meat, and that the maggots were the offspring of flies, not the product of spontaneous generation. Proponents of spontaneous generation were not convinced. They argued that fresh air was required for spontaneous generation. So Redi redid is experiments, but this time instead of sealing the jars completely, he covered them with a fine net of gauze. This set-up allowed for air to enter the jar, but still prevented the flies from entering. No maggots appeared on the meat in the gauze-covered jars even in the presence of fresh air.
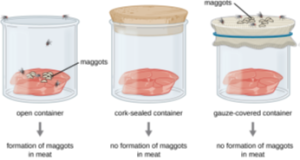
Redi’s results provided strong evidence that organism could not just arise form nonliving matter. Despite this evidence, many scientists believed that while spontaneous generation may not hold true for larger organism, microorganism were simple enough to be able to generate spontaneously.
This argument was strengthened in 1745, when John Needham (1713–1781) published a report of his own experiments, in which he briefly boiled broth infused with plant or animal matter, hoping to kill all preexisting microbes. He then sealed the flasks. After a few days, Needham observed that the broth had become cloudy and a single drop contained numerous microscopic creatures. He argued that the new microbes must have arisen spontaneously.
Lazzaro Spallanzani (1729–1799) did not agree with Needham’s conclusions, and suggested that microorganisms from the air had contaminated Needham’s broth after it had been boiled. To test his hypothesis, he performed hundreds of carefully executed experiments using heated broth. As in Needham’s experiment, he used infused broth, but boiling the broth before sealing the flasks, he sealed some flasks before boiling them. Spallanzani’s results contradicted the findings of Needham: Heated but sealed flasks remained clear, without any signs of spontaneous growth, unless the flasks were subsequently opened to the air. This suggested that microbes were introduced into these flasks from the air. In response to Spallanzani’s findings, Needham argued that life originates from a “vital force” that was destroyed during Spallanzani’s extended boiling. Sealing of the flasks then prevented this force from entering and causing spontaneous generation. Spallanzani’s experiments also drew the same criticism as had Redi’s: by sealing the flasks, he was preventing air from entering, which would prevent spontaneous generation from occurring (Figure 1.14).
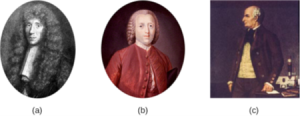
The debate over spontaneous generation continued well into the 19th century, with scientists serving as proponents of both sides. To settle the debate, the Paris Academy of Sciences offered a prize for resolution of the problem. Louis Pasteur, a prominent French chemist who had been studying microbial fermentation and the causes of wine spoilage, accepted the challenge. In 1858, Pasteur filtered air through a gun-cotton filter and, upon microscopic examination of the cotton, found it full of microorganisms, suggesting that the exposure of a broth to air was not introducing a “life force” to the broth but rather airborne microorganisms.
Later, Pasteur made a series of flasks with long, twisted necks (“swan-neck” flasks), in which he boiled broth to sterilize it (Figure 1.15). His design allowed air inside the flasks to be exchanged with air from the outside, but prevented the introduction of any airborne microorganisms, which would get caught in the twists and bends of the flasks’ necks. If a life force besides the airborne microorganisms were responsible for microbial growth within the sterilized flasks, it would have access to the broth, whereas the microorganisms would not. He correctly predicted that sterilized broth in his swan-neck flasks would remain sterile as long as the swan necks remained intact. However, should the necks be broken, microorganisms would be introduced, contaminating the flasks and allowing microbial growth within the broth.
Pasteur’s set of experiments irrefutably disproved the theory of spontaneous generation and earned him the prestigious Alhumbert Prize from the Paris Academy of Sciences in 1862. In a subsequent lecture in 1864, Pasteur articulated “Omne vivum ex vivo” (“Life only comes from life”). In this lecture, Pasteur recounted his famous swan-neck flask experiment, stating that “…life is a germ and a germ is life. Never will the doctrine of spontaneous generation recover from the mortal blow of this simple experiment.” To Pasteur’s credit, it never has.
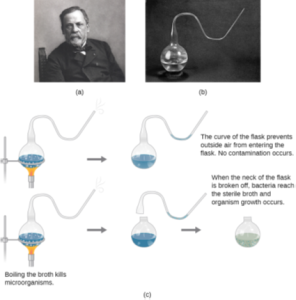
While some scientists were arguing over the theory of spontaneous generation, other scientists were making discoveries leading to a better understanding of what we now call the cell theory. Modern cell theory has two basic tenets:
• All cells only come from other cells.
• Cells are the fundamental units of organisms.
Today, these tenets are fundamental to our understanding of life on earth. However, modern cell theory grew out of the collective work of many scientists.
Recall that the English scientist Robert Hooke first used the term “cells” in 1665 to describe the small chambers within cork that he observed under a microscope of his own design (See Figure 1.11). At the time, Hooke was not aware that the cork cells were long dead and, therefore, lacked the internal structures found within living cells.
Despite Hooke’s early description of cells, their significance as the fundamental unit of life was not yet recognized. Nearly 200 years later, in 1838, Matthias Schleiden (1804–1881), a German botanist who made extensive microscopic observations of plant tissues, described them as being composed of cells. Visualizing plant cells was relatively easy because plant cells are clearly separated by their thick cell walls. Schleiden believed that cells formed through crystallization, rather than cell division.
Theodor Schwann (1810–1882), a noted German physiologist, made similar microscopic observations of animal tissue. In 1839, after a conversation with Schleiden, Schwann realized that similarities existed between plant and animal tissues. This laid the foundation for the first tenet of cell theory, that cells are the fundamental components of plants and animals.
In the 1850s, two Polish scientists living in Germany pushed this idea further. In 1852, Robert Remak (1815–1865), a prominent neurologist and embryologist, published convincing evidence that cells are derived from other cells as a result of cell division. However, this idea was questioned by many in the scientific community. Three years later, Rudolf Virchow (1821–1902), a well-respected pathologist, published an editorial essay entitled “Cellular Pathology,” which popularized the concept of biogenesis, using the Latin phrase omnis cellula a cellula (“all cells arise from cells”), which is essentially the second tenet of modern cell theory. Given the similarity of Virchow’s work to Remak’s, there is some controversy as to which scientist should receive credit for articulating biogenesis (Figure 1.16).
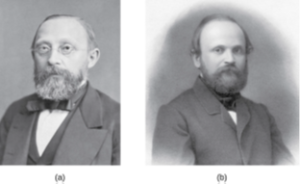
Prior to the discovery of microbes during the 17th century, other theories circulated about the origins of disease. For example, the ancient Greeks proposed the miasma theory, which held that disease originated from particles emanating from decomposing matter, such as that in sewage or cesspits. Such particles infected humans in close proximity to the rotting material. Diseases including the Black Death, which ravaged Europe’s population during the Middle Ages, were thought to have originated in this way.
In 1546, Italian physician Girolamo Fracastoro proposed, in his essay De Contagione et Contagiosis Morbis, that seed-like spores may be transferred between individuals through direct contact, exposure to contaminated clothing, or through the air. We now recognize Fracastoro as an early proponent of the germ theory of disease, which states that diseases may result from microbial infection. However, in the 16th century, Fracastoro’s ideas were not widely accepted and would be largely forgotten until the 19th century.
In 1847, Hungarian obstetrician Ignaz Semmelweis (Figure 1.17) observed that mothers who gave birth in hospital wards staffed by physicians and medical students were more likely to suffer and die from puerperal fever after childbirth (10%–20% mortality rate) than were mothers in wards staffed by midwives (1% mortality rate). Semmelweis observed medical students performing autopsies and then subsequently carrying out vaginal examinations on living patients without washing their hands in between. He suspected that the students carried disease from the autopsies to the patients they examined. His suspicions were supported by the untimely death of a friend, a physician who contracted a fatal wound infection after a postmortem examination of a woman who had died of a puerperal infection. The dead physician’s wound had been caused by a scalpel used during the examination, and his subsequent illness and death closely paralleled that of the dead patient.
Although Semmelweis did not know the true cause of puerperal fever, he proposed that physicians were somehow transferring the causative agent to their patients. He suggested that the number of puerperal fever cases could be reduced if physicians and medical students simply washed their hands with chlorinated lime water before and after examining every patient. When this practice was implemented, the maternal mortality rate in mothers cared for by physicians dropped to the same 1% mortality rate observed among mothers cared for by midwives. This demonstrated that handwashing was a very effective method for preventing disease transmission. Despite this great success, many discounted Semmelweis’s work at the time, and physicians were slow to adopt the simple procedure of handwashing to prevent infections in their patients because it contradicted established norms for that time period.
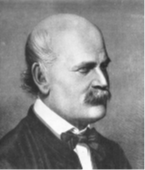
While studying the causes of beer and wine spoilage in 1856, Pasteur discovered properties of fermentation by microorganisms. He had demonstrated with his swan-neck flask experiments (Figure 1.15) that airborne microbes, not spontaneous generation, were the cause of food spoilage, and he suggested that if microbes were responsible for food spoilage and fermentation, they could also be responsible for causing infection. This was the foundation for the germ theory of disease.
Meanwhile, British surgeon Joseph Lister (Figure 1.18) was trying to determine the causes of postsurgical infections. Many physicians did not give credence to the idea that microbes on their hands, on their clothes, or in the air could infect patients’ surgical wounds, despite the fact that 50% of surgical patients, on average, were dying of postsurgical infections. Lister, however, was familiar with the work of Semmelweis and Pasteur; therefore, he insisted on handwashing and extreme cleanliness during surgery. In 1867, to further decrease the incidence of postsurgical wound infections, Lister began using carbolic acid (phenol) spray disinfectant/antiseptic during surgery. His extremely successful efforts to reduce postsurgical infection caused his techniques to become a standard medical practice.
The first proof that bacteria could cause disease came from Robert Koch (Figure 1.19). In 1876, Koch, a German physician, was attempting to identify the cause of anthrax, a disease that was ravaging the sheep and cattle populations in Europe. He discovered a bacteria in the blood of cattle that had died from anthrax. Koch cultured these bacteria in his lab and then injected samples of these bacteria into healthy animals. When these animals became sick and died, he was able to isolate bacteria from their blood and in comparing them to the original sample, found that they were identical. From these early experiments Koch proposed a series of postulates (Koch’s postulates) based on the idea that the cause of a specific disease could be attributed to a specific microbe. Using these postulates, Koch and his colleagues went on to definitively identify the causative pathogens of others diseases, including tuberculosis and cholera. Koch’s “one microbe, one disease” concept was the culmination of the 19th century’s paradigm shift away from miasma theory and toward the germ theory of disease. Koch’s postulates will be discussed more thoroughly in a later unit.
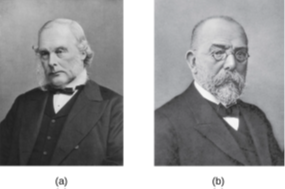
Thousands of years ago, it was first recognized that individuals who survived a smallpox infection were immune to subsequent infections. The practice of inoculating individuals to actively protect them from smallpox appears to have originated in the 10th century in China, when the practice of variolation was described. Variolation refers to the deliberate inoculation of individuals with infectious material from scabs or pustules of smallpox victims. Infectious materials were either injected into the skin or introduced through the nasal route. The infection that developed was usually milder than naturally acquired smallpox, and recovery from the milder infection provided protection against the more serious disease.
Although the majority of individuals treated by variolation developed only mild infections, the practice was not without risks. More serious and sometimes fatal infections did occur, and because smallpox was contagious, infections resulting from variolation could lead to epidemics. Even so, the practice of variolation for smallpox prevention spread to other regions, including India, Africa, and Europe.
Although variolation had been practiced for centuries, the English physician Edward Jenner (1749–1823) is generally credited with developing the modern process of vaccination. Jenner observed that milkmaids who developed cowpox, a disease similar to smallpox but milder, were immune to the more serious smallpox. This led Jenner to hypothesize that exposure to a less virulent pathogen could provide immune protection against a more virulent pathogen, providing a safer alternative to variolation. In 1796, Jenner tested his hypothesis by obtaining infectious samples from a milkmaid’s active cowpox lesion and injecting the materials into a young boy (Figure 1.19). The boy developed a mild infection that included a low-grade fever, discomfort in his axillae (armpit) and loss of appetite. When the boy was later infected with infectious samples from smallpox lesions, he did not contract smallpox. This new approach was termed vaccination, a name deriving from the use of cowpox (Latin vacca meaning “cow”) to protect against smallpox. Today, we know that Jenner’s vaccine worked because the cowpox virus is genetically and antigenically related to the Variola viruses that caused smallpox. The mechanisms of how vaccinations work are discussed in the immune system unit.
The success of Jenner’s smallpox vaccination led other scientists to develop vaccines for other diseases. Perhaps the most notable was Louis Pasteur, who developed vaccines for rabies, cholera, and anthrax. During the 20th and 21st centuries, effective vaccines were developed to prevent a wide range of diseases caused by viruses (e.g., chickenpox and shingles, hepatitis, measles, mumps, polio, and yellow fever) and bacteria (e.g., diphtheria, pneumococcal pneumonia, tetanus, and whooping cough,).
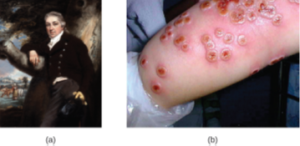
The Search for the ‘Magic Bullet’:
With the relationship between diseases and microorganism firmly establish, scientist began to focus their attention on finding treatments that could be used to eliminate pathogens from their hosts. Treating diseases with chemical substances is known as chemotherapy and can involve the use of natural and/or manufactured compounds. Naturally occurring chemicals that are produced by microorganisms to affect other microorganism are known as antibiotics, whereas those produced in a laboratory are called synthetic drugs.
In the early 1900s, the German physician and scientist Paul Ehrlich (1854–1915) set out to discover or synthesize what he called ‘magic bullets’: chemical compounds capable of killing infectious microbes without harming the patient. In 1909, after screening more than 600 arsenic-containing compounds, Ehrlich’s assistant finally found one such chemical. Compound 606 targeted the bacterium Treponema pallidum, the causative agent of syphilis. Compound 606 was found to successfully cure syphilis in rabbits and soon after was marketed under the name Salvarsan as a remedy for the disease in humans (Figure 14.3). Ehrlich’s innovative approach of systematically screening a wide variety of compounds remains a common strategy for the discovery of new antimicrobial agents even today.
The first antibiotic was discovered by accident. In 1928, Alexander Fleming (1881–1955) returned from holiday and examined some old plates of bacteria in his research laboratory at St. Mary’s Hospital in London. He observed that contaminating mold growth (subsequently identified as a strain of Penicillium chrysogenum) inhibited bacterial growth on one plate. Fleming, therefore, is credited with the discovery of penicillin, the first natural antibiotic, (Figure 1.20). Further experimentation showed that penicillin from the mold was antibacterial against streptococci, meningococci, and Corynebacterium diphtheriae, the causative agent of diphtheria.
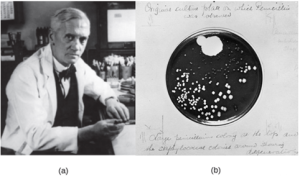
living organisms can be created from non-living matter (e.g., maggots from meat)
All life consists of cells, which can only arise from other cells.
Cells arise only from the division of existing cells.
Some diseases are a result of infection by microbes.
Antimicrobials that are initially produced by living organisms.
Chemicals that slow the growth of, or kill, microbes.