Chapter 13. Nutrition and Physical Activity
Fuel Sources During Activity
The human body uses carbohydrates, fats, and proteins in food and our bodily stores as energy. These essential nutrients are needed regardless of the intensity of the activity you are performing. If you are lying down reading a book or running the Vancouver Marathon, these macronutrients are always needed in the body. However, for these nutrients to be used as fuel for the body, their energy must be transferred into the high-energy molecule Adenosine Triphosphate (ATP).
ATP supplies the energy for muscle contraction to take place. Muscle contraction does not occur without sufficient amounts of ATP. The amount of ATP stored in muscle is very low, only enough to power a few seconds’ worth of contractions. As it is broken down, ATP must be regenerated and replaced quickly to allow for sustained contraction. There are three mechanisms by which ATP can be regenerated in muscle cells: creatine phosphate metabolism, anaerobic glycolysis, and aerobic respiration.[1]
The ATP-PC (Phosphocreatine) System
Once ATP stores have been used (after approx. 1-3 seconds), the creatine phosphate system helps generate the ATP that fuels muscle contraction.
Creatine phosphate is a molecule that can store energy in its phosphate bonds. In a resting muscle, excess ATP transfers its energy to creatine, producing ADP and creatine phosphate. This acts as an energy reserve that can quickly be used to create more ATP. When the muscle starts to contract and needs energy, creatine phosphate transfers its phosphate back to ADP to form ATP and creatine. This reaction is catalyzed by the enzyme creatine kinase and occurs very quickly; thus, creatine phosphate-derived ATP powers the first few seconds of muscle contraction. The creatine phosphate system domnates energy production for high-intensity activities lasting 3-15/20s, after this time, it contributes significantly less[2][3].
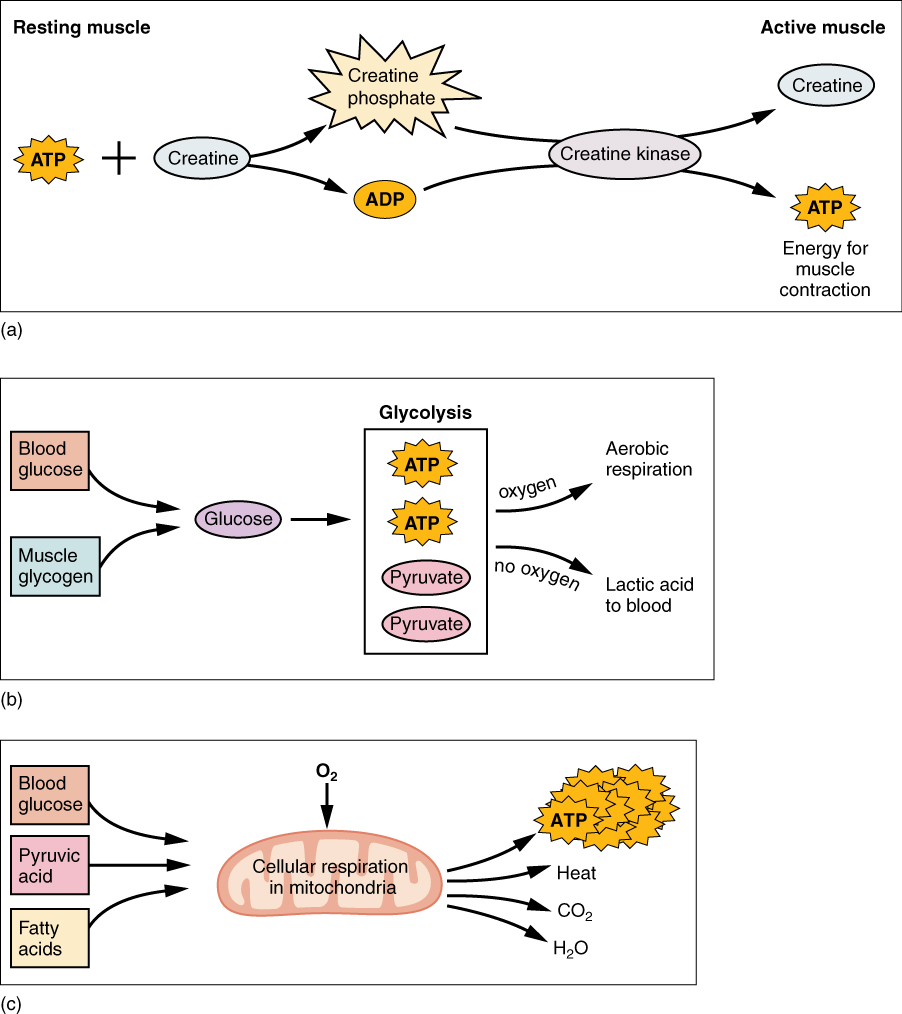
(a) Some ATP is stored in a resting muscle. As contraction starts, it is used up in seconds. More ATP is generated from creatine phosphate for about 15 seconds. (b) Each glucose molecule produces two ATP and two molecules of pyruvic acid, which can be used in aerobic respiration or converted to lactic acid. If oxygen is not available, pyruvic acid is converted to lactic acid, which may contribute to muscle fatigue. This occurs during strenuous exercise when high amounts of energy are needed but oxygen cannot be sufficiently delivered to muscle. (c) Aerobic respiration is the breakdown of glucose in the presence of oxygen (O2) to produce carbon dioxide, water, and ATP. Approximately 95 percent of the ATP required for resting or moderately active muscles is provided by aerobic respiration, which takes place in mitochondria.
Anaerobic Glycolysis and Aerobic Respiration
Glycolysis is the process that breaks down glucose (sugar) to produce ATP, it can be both aerobic (oxygen dependent) and an anaerobic (non-oxygen-dependent). As the ATP produced by creatine phosphate is depleted, muscles turn to anaerobic glycolysis as an ATP source, however, glycolysis cannot generate ATP as quickly as creatine phosphate. Thus, the switch to glycolysis results in a slower rate of ATP availability to the muscle. The sugar used in anaerobic glycolysis is provided from the muscle (stored glycogen is broken down into glucose). The breakdown of one glucose molecule produces two ATP and two molecules of pyruvic acid/pyruvate, which can be used in aerobic respiration or when oxygen levels are low, can be converted to lactic acid (Figure 13.2b).[4][5] When lactic acid/lactate accumulates in cells during exercise, it diffuses out of the muscle and into the bloodstream, it then travels to the liver and is converted back into glucose in a process known as the Cori Cycle. Anaerobic glycolysis is the dominant energy system for all out activities that last approx. 20s-2/3 min.
Anaerobic metabolism occurs in the cytosol of the muscle cells. As seen in Figure 13.3 “Anaerobic versus aerobic metabolism”, a small amount of ATP is produced in the cytosol without the presence of oxygen. If oxygen is not available, pyruvic acid/pyruvate is converted to lactic acid, which may contribute to muscle fatigue. If oxygen is available, pyruvic acid/ pyruvate can be used as fuel for aerobic respiration/metabolism. Aerobic metabolism takes place in the mitochondria of the cell and can use carbohydrates, proteins, or fats as its fuel source. Aerobic metabolism is a much slower process than anaerobic metabolism, but it produces the majority of ATP.
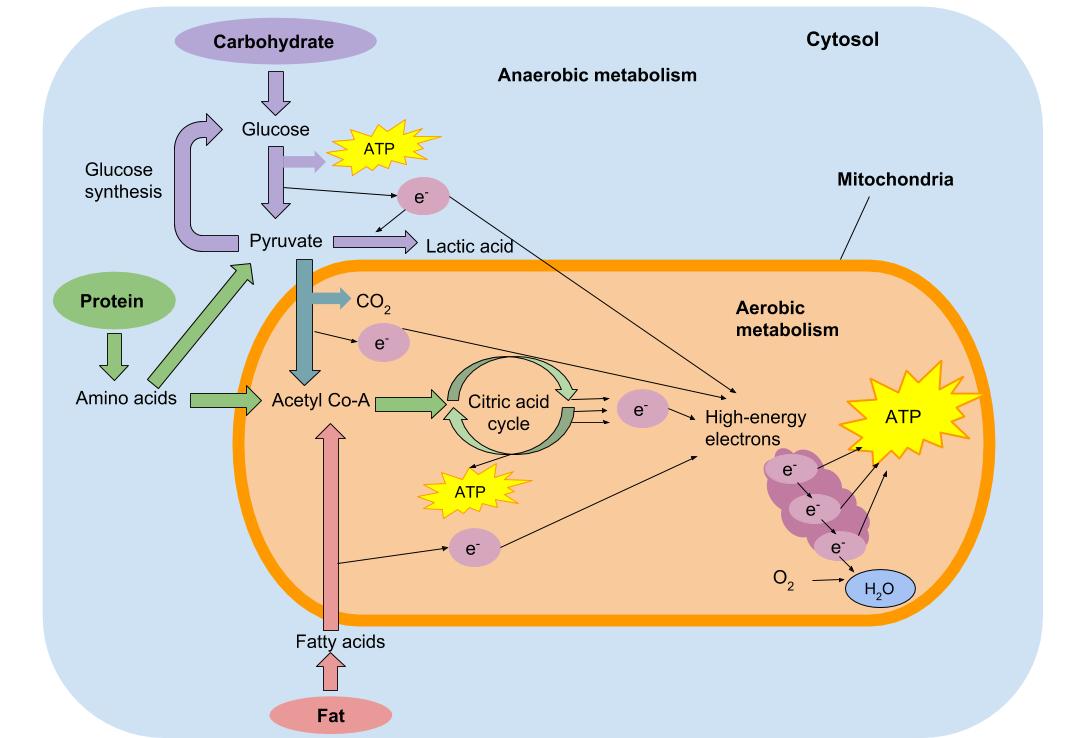
Physical Activity Duration and Fuel Use
The respiratory system plays a vital role in the uptake and delivery of oxygen to muscle cells throughout the body. Oxygen is inhaled into the lungs and transferred to the blood where the cardiovascular system circulates the oxygen-rich blood to the muscles where it is used to generate ATP. When the body is at rest, the heart and lungs can supply the muscles with adequate amounts of oxygen to meet the aerobic metabolism energy needs. However, during physical activity, your muscles’ energy and oxygen needs are increased. In order to provide more oxygen to the muscle cells, your heart rate and breathing rate will increase. The amount of oxygen delivered to the tissues via the cardiovascular and respiratory systems during exercise depends on the exercise duration and intensity, and the physical conditioning of an individual.
During the first few steps of exercise, your muscles are the first to respond to the change in activity level. Your lungs and heart, however, do not react as quickly and during those first few steps, they do not begin to increase the delivery of oxygen. For our bodies to get the energy needed in these beginning steps, the muscles rely on a small amount of ATP stored in resting muscles. The stored ATP can provide energy for only a few seconds before it is depleted. As shown in Figure 13.4[6][/footnote], once the stored ATP is just about used up, the body resorts to another high-energy molecule known as creatine phosphate to convert ADP (adenosine diphosphate) to ATP, and this system dominates ATP production for all out activities lasting approx. 15-20s. After about 15-20 seconds into exercise, the stored creatine phosphate are depleting rapidly up in the muscles. The heart and lungs have still not adapted to the increased need for oxygen so the muscles must begin to produce ATP by anaerobic glycolysis (without oxygen), which is the dominant energy system for activities lasting from approx. 20s/30s- 2/3 min.
As you continue exercising for two to three minutes, your heart rate and breathing rate will increase to supply more oxygen to your muscles. Aerobic metabolism is the most efficient way of producing ATP as it produces 18 times more ATP for each molecule of glucose than anaerobic metabolism. Although the primary source of ATP in aerobic metabolism is carbohydrates, fatty acids and amino acids can also be used as fuel to generate ATP.
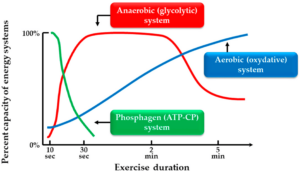
The fuel sources for anaerobic and aerobic metabolism will change depending on the amount of nutrients available and the type of metabolism. Glucose may come from blood glucose (which is from dietary carbohydrates, liver glycogen, or glucose synthesis) or muscle glycogen. Fatty acids are stored as triglycerides in muscles, but about 90% of stored energy is found in adipose tissue. As low to moderate-intensity exercise continues using aerobic metabolism, fatty acids become the predominant fuel source for the exercising muscles. Although protein is not considered a major energy source, small amounts of amino acids are used while resting or performing an activity. The amount of amino acids used for energy metabolism increases if the total dietary energy intake does not meet the nutrient needs or if you are involved in long endurance exercises. When amino acids are broken down removing the nitrogen-containing amino acid, the remaining carbon molecule can be broken down into ATP via aerobic metabolism or used to make glucose. When exercise continues for many hours, amino acid use will increase as an energy source and for glucose synthesis.
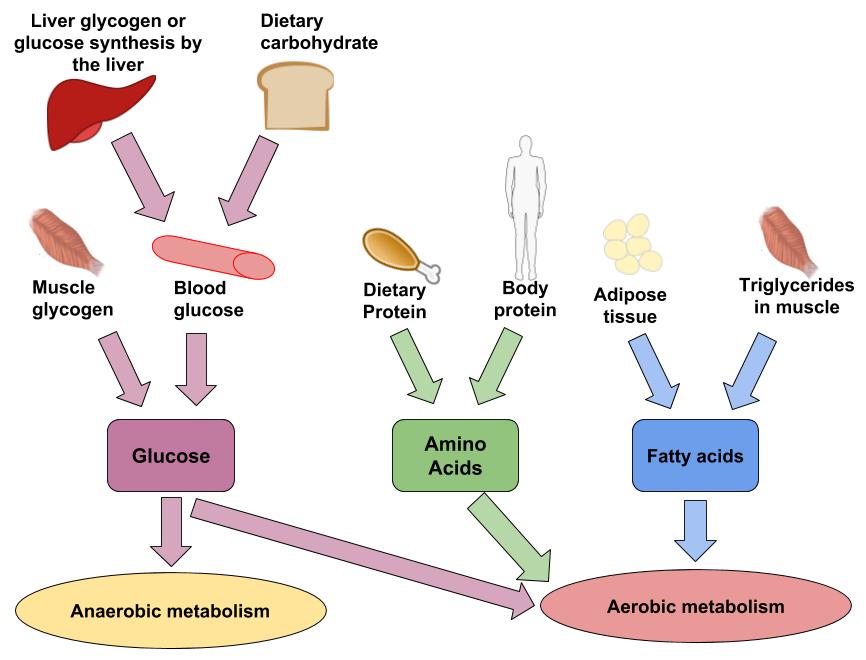
Physical Activity Intensity and Fuel Use
The exercise intensity determines the contribution of the type of fuel source used for ATP production (see Figure 13.6 “The effect of exercise intensity on fuel sources”). Both anaerobic and aerobic metabolism combine during exercise to ensure that the muscles are equipped with enough ATP to carry out the demands placed on them. The amount of contribution from each type of metabolism will depend on the intensity of the activity. When low-intensity activities are performed, aerobic metabolism is used to supply enough ATP to muscles. However, when high-intensity activities are performed, more ATP is needed so muscles must rely on both anaerobic and aerobic metabolism to meet the body’s demands.
The higher the intensity of exercise, the more our bodies rely on carbohydrates to fuel activity. During low-intensity activities, the body will use aerobic metabolism over anaerobic metabolism because it is more efficient and produces larger amounts of ATP. Fatty acids are the primary energy source during low-intensity activity. With fat reserves in the body being almost unlimited, low-intensity activities can continue for a long period of time. Along with fatty acids, a small amount of glucose is used as well. However, unlike fat stores, glycogen stores can be depleted. As glycogen stores are depleted, fatigue will eventually set in.
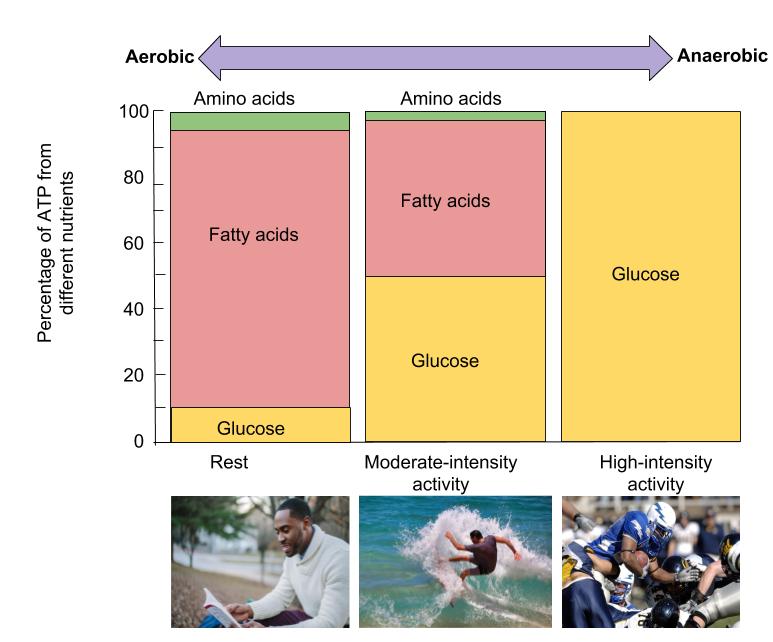
“Hitting the Wall” or “Bonking”
If you are familiar with endurance sports, you may have heard of “hitting the wall” or “bonking.” These colloquial terms refer to the extreme fatigue that sets in after about 1-2 hours of performing an endurance sport, such as marathon running or long-distance cycling or repeated interval sessions. The physiology underlying “hitting the wall” means that muscles have used up all their stored glycogen and are therefore dependent on other nutrients to support their energy needs. Fatty acids are transported from fat-storing cells to the muscle to rectify the nutrient deficit. However, fatty acids take more time to convert to energy than glucose, thus decreasing performance levels. To avoid “hitting the wall” or “bonking,” endurance athletes can load up on carbohydrates for a few days before the event, in a process known as carbohydrate loading. This will maximize the amount of glycogen stored in their liver and muscle tissues. It is important to not assume that carbohydrate loading works for everyone. For example, it’s only effective for events longer than 90 minutes. Another way for athletes to avoid “hitting the wall” is to consume carbohydrate-containing drinks and foods during an endurance event. In fact, throughout the Tour de France—a twenty-two-day, twenty-four-hundred-mile race, the average cyclist consumes more than 60-90 grams of carbohydrates per hour.
Media Attributions
- Muscle metabolism © OpenStax is licensed under a CC BY (Attribution) license
- Exercise-intensity-and-Fuel- is licensed under a CC BY (Attribution) license
- Betts J. Gordon, Young KA, Wise JA, Johnson E, Poe B, Kruse BH, et al. Anatomy and Physiology 2e [Internet]. 2nd ed. Houston, Texas: Open Stax; 2022 [cited 2023 Mar 13]. Available from: https://openstax.org/books/anatomy-and-physiology-2e/pages/1-introduction ↵
- Kraemer, W.J., Fleck, S.J., & Deschenes, M.R. (2021). Exercise Physiology: Integrating Theory and Application. 3rd edition. ISBN: 9781975117429 ↵
- Plowman, SA. and Smith DL. Exercise Physiology For Health, Fitness and Performance, 5th Ed. ISBN: 978-0702029349 ↵
- Plowman, SA. and Smith DL. Exercise Physiology For Health, Fitness and Performance, 5th Ed. ISBN: 978-0702029349 ↵
- Kraemer, W.J., Fleck, S.J., & Deschenes, M.R. (2021). Exercise Physiology: Integrating Theory and Application. 3rd edition. ISBN: 9781975117429 ↵
- [footnote]Yamasaki, T. Benefits of Table Tennis for Brain Health Maintenance and Prevention of Dementia. Encyclopedia 2022, 2(3), 1577-1589; https://doi.org/10.3390/encyclopedia2030107 ↵