49 10.4 Biology of Memory
Learning Objectives
- Describe what neuronal activities are associated with the formation of memories.
- Describe the role of the hippocampus in the formation of memories.
- Describe retrograde and anterograde amnesia.
The biology of memory
Just as information is stored on digital media, the information in long-term memory (LTM) must be stored in the brain. The ability to maintain information in LTM involves a gradual strengthening of the connections among the neurons in the brain. When pathways in these neural networks are frequently and repeatedly fired, the synapses become more efficient in communicating with each other, and these changes create memory. This process, known as long-term potentiation (LTP), refers to the strengthening of the synaptic connections between neurons as a result of frequent stimulation (Lynch, 2002). Drugs that block LTP reduce learning, whereas drugs that enhance LTP increase learning (Lynch et al., 1991). Because the new patterns of activation in the synapses take time to develop, LTP happens gradually. The period of time in which LTP occurs and in which memories are stored is known as the period of consolidation.
Long-term potentiation occurs as a result of changes in the synapses, which suggests that chemicals, particularly neurotransmitters and hormones, must be involved in memory. There is quite a bit of evidence that this is true. Glutamate, a neurotransmitter and a form of the amino acid glutamic acid, is perhaps the most important neurotransmitter in memory (McEntee & Crook, 1993). When animals, including people, are under stress, more glutamate is secreted, and this glutamate can help them remember (McGaugh, 2003). The neurotransmitter serotonin is also secreted when animals learn, and epinephrine may also increase memory, particularly for stressful events (Maki & Resnick, 2000; Sherwin, 1998). Estrogen, a sex hormone, also seems critical for memory for both sexes (e.g., Cheung, Chervonsky, Felmingham, & Bryant, 2013; Korol & Pisani, 2015). Interestingly, women who are experiencing menopause, along with a reduction in estrogen, frequently report memory difficulties (Chester, 2001).
Our knowledge of the role of biology in memory suggests that it might be possible to use drugs to improve our memories, and North Americans spend several hundred million dollars per year on memory supplements with the hope of doing just that. Yet, controlled studies comparing memory enhancers, such as Ritalin, methylphenidate, ginkgo biloba, and amphetamines, with placebo drugs find very little evidence for their effectiveness (Gold, Cahill, & Wenk, 2002; McDaniel, Maier, & Einstein, 2002). Memory supplements are usually no more effective than drinking a sugared soft drink, which also releases glucose and thus improves memory slightly. This is not to say that we cannot someday create drugs that will significantly improve our memory. It is likely that this will occur in the future, but the implications of these advances are as yet unknown (Farah et al., 2004; Turner & Sahakian, 2006).
Although the most obvious potential use of drugs is to attempt to improve memory, drugs might also be used to help us forget. This might be desirable in some cases, such as for those suffering from post-traumatic stress disorder (PTSD) who are unable to forget disturbing memories. Although there are no existing therapies that involve using drugs to help people forget, it is possible that they will be available in the future. These possibilities will raise some important ethical issues: is it ethical to erase memories, and if it is, is it desirable to do so? Perhaps the experience of emotional pain is a part of being a human being, and perhaps the experience of emotional pain may help us cope with the trauma.
Where in the brain are memories made and stored? This is not an easy question to answer. Memory occurs through sophisticated interactions between evolutionarily new and old brain structures (see Figure 10.12). One of the most important brain regions in explicit memory is the hippocampus, which serves as a preprocessor and elaborator of information (Squire, 1992). The hippocampus helps us encode information about spatial relationships, the context in which events were experienced, and the associations among memories (Eichenbaum, 1999). The hippocampus also serves in part as a switching point that holds the memory for a short time and then directs the information to other parts of the brain, such as the cortex, to actually do the rehearsing, elaboration, and long-term storage (Jonides, Lacey, & Nee, 2005). Without the hippocampus, which might be described as the brain’s “librarian,” our explicit memories would be inefficient and disorganized. Eleanor Maguire, Katherine Woollett, and Hugo Spiers (2006) found that the navigational abilities of London taxi drivers were related to the size of the hippocampus, especially on the right side of the brain. London taxi drivers are required to memorize an enormous amount of spatial and navigational information in order to travel around London’s complex network of roads. On the flip side, people with diseases that compromise the hippocampus tend to show reduced ability to spatially navigate (Konishi, Mckenzie, Etcharnendy, Roy, & Bobhot, 2017). Despite these intriguing findings, we have yet to understand the full role of the hippocampus in ordinary people of a range of ages; what is certain is that memory is dependent on a functioning hippocampus.
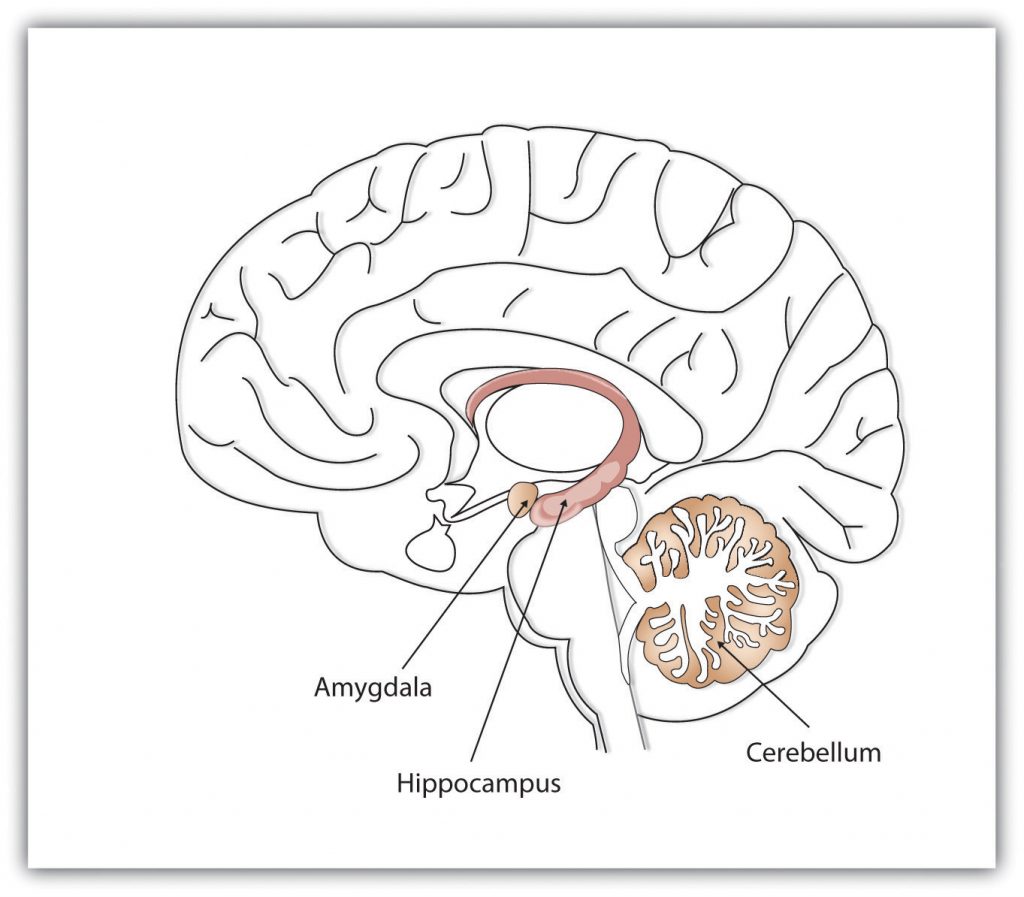
While the hippocampus is handling explicit memory, other brain structures such as the cerebellum and the amygdala are concentrating on implicit and emotional memories, respectively. Research shows that the cerebellum is more active when we are learning associations and in priming tasks, and animals and humans with damage to the cerebellum have more difficulty in classical conditioning studies (Krupa, Thompson, & Thompson, 1993; Woodruff-Pak, Goldenberg, Downey-Lamb, Boyko, & Lemieux, 2000). The storage of many of our most important emotional memories, and particularly those related to fear, is initiated and controlled by the amygdala, which is activated by emotional arousal (Sigurdsson, Doyère, Cain, & LeDoux, 2007). The amygdala functions as a swift-acting processor of threat; recall of emotional memories is associated with activation in the amygdala (McGaugh, Cahill, & Roozendaal, 1996).
Evidence for the role of different brain structures in different types of memories comes in part from case studies of patients who suffer from amnesia, which is a memory disorder that involves the inability to remember information. As with memory interference effects, amnesia can work in either a forward or a backward direction, affecting retrieval or encoding. For people who suffer damage to the brain, for instance, as a result of a stroke or other trauma, the amnesia may work backward. The outcome is retrograde amnesia, which is a memory disorder that produces an inability to retrieve events that occurred before a given time. Demonstrating the fact that the process of memory consolidation takes time (see long-term potentiation above), retrograde amnesia is usually more severe for memories that occurred just prior to the trauma than it is for older memories, and events that occurred just before the event that caused memory loss may never be recovered because they were never completely encoded.
Damage to the hippocampus results in a type of amnesia that works in a forward direction, meaning after the damage to the hippocampus, new memories cannot be encoded. This is known as anterograde amnesia. Anterograde amnesia is the inability to transfer information from short-term into long-term memory, making it impossible to form new memories. One well-known case study was a man named Henry Gustav Molaison — before he died in 2008, he was referred to only as H. M. — who had parts of his hippocampus removed to reduce severe seizures (Corkin, Amaral, González, Johnson, & Hyman, 1997). Following the operation, Molaison developed virtually complete anterograde amnesia. Although he could remember most of what had happened before the operation, and particularly what had occurred early in his life, he could no longer create new memories. Molaison was said to have read the same magazines over and over again without any awareness of having seen them before.
Cases of anterograde amnesia also provide information about the brain structures involved in different types of memory (Bayley & Squire, 2005; Helmuth, 1999; Paller, 2004). Although Molaison’s explicit memory was compromised because his hippocampus was damaged, his implicit memory was not because his cerebellum was intact. He could learn to trace shapes in a mirror, a task that requires procedural memory, but he never had any explicit recollection of having performed this task or of the people who administered the test to him.
Kent Cochrane — before his death in 2014, he was simply referred to as K. C. — was born in Toronto in 1951 and lived with global anterograde amnesia and retrograde episodic amnesia after a motorcycle accident in 1981. His brain was among the most studied in the world. Cochrane suffered from severe brain damage, including bilateral lesions to the hippocampus. Cochrane was able to make use of knowledge and experiences from before his accident; his semantic memory was largely preserved. However, his episodic memory of anything before his accident was completely obliterated. Research conducted on Cochrane has shown that he was able to recall factual information learned prior to his accident, such as the difference between stalactites and stalagmites, but was unable to remember emotional details, such as his brother’s death and a dangerous fall he had (Rosenbaum et al., 2005). As another example, Cochrane could identify people in a family photograph of his brother’s wedding party, but he was unable to recall what event was depicted. He was unable to recollect any events in which he had participated or witnessed. Cochrane’s case is important because it shows that there are different “forms'” of amnesia, which means that different types of memories may rely on different areas of the brain. If semantic and episodic memory can be differentially affected by brain damage to structures like the hippocampus, this suggests that these memories processes are to some extent independent. Extrapolating from cases like H. M. and K. C. will help researchers to understand how memory works in people with normal brains.
Although some brain structures are particularly important in forming memories, this does not mean that all memories are stored in one place. The psychologist Karl Lashley (1929) attempted to determine where memories were stored in the brain by teaching rats how to run mazes, and then lesioning different brain structures to see if they were still able to complete the maze. This idea seemed straightforward, and Lashley expected to find that memory was stored in certain parts of the brain. However, Lashley discovered that no matter where he removed brain tissue, the rats retained at least some memory of the maze, leading him to conclude that memory is not located in a single place in the brain, but rather is distributed throughout it.
Key Takeaways
- When pathways in neural networks are frequently and repeatedly fired, the synapses become more efficient in communicating with each other, and these changes create memory.
- Memories are stored in connected synapses through the process of long-term potentiation. In addition to the cortex, other parts of the brain, including the hippocampus, cerebellum, and the amygdala, are also important in memory.
- Damage to the brain may result in retrograde amnesia or anterograde amnesia. Case studies of patients with amnesia can provide information about the brain structures involved in different types of memory.
- Memory is influenced by chemicals including glutamate, serotonin, epinephrine, and estrogen.
- The hippocampus is critical for the formation and storage of memories.
- Studies comparing memory enhancers with placebo drugs find very little evidence for their effectiveness.
- Memories are not stored in one place in the brain since they involve many brain structures.
Image Attributions
Figure 10.12. Used under a CC BY-NC-SA 4.0 license.
References
Bayley, P. J., & Squire, L. R. (2005). Failure to acquire new semantic knowledge in patients with large medial temporal lobe lesions. Hippocampus, 15(2), 273–280.
Chester, B. (2001). Restoring remembering: Hormones and memory. McGill Reporter, 33(10). Retrieved from http://www.mcgill.ca/reporter/33/10/sherwin
Cheung, J., Chervonsky, L., Felmingham, K. L., & Bryant, R, A. (2013). The role of estrogen in intrusive memories. Neurobiology of Learning and Memory, 106, 87–94.
Corkin, S., Amaral, D. G., González, R. G., Johnson, K. A., & Hyman, B. T. (1997). H. M.’s medial temporal lobe lesion: Findings from magnetic resonance imaging. The Journal of Neuroscience, 17(10), 3964–3979.
Eichenbaum, H. (1999). Conscious awareness, memory, and the hippocampus. Nature Neuroscience, 2(9), 775–776.
Farah, M. J., Illes, J., Cook-Deegan, R., Gardner, H., Kandel, E., King, P., . . . Wolpe, P. R. (2004). Neurocognitive enhancement: What can we do and what should we do? Nature Reviews Neuroscience, 5(5), 421–425.
Gold, P. E., Cahill, L., & Wenk, G. L. (2002). Ginkgo biloba: A cognitive enhancer? Psychological Science in the Public Interest, 3(1), 2–11.
Helmuth, L. (1999). New role found for the hippocampus. Science, 285, 1339–1341.
Jonides, J., Lacey, S. C., & Nee, D. E. (2005). Processes of working memory in mind and brain. Current Directions in Psychological Science, 14(1), 2–5.
Konishi, K., Mckenzie, S., Etcharnendy, N., Roy, S., & Bohbot, V. D. (2017). Hippocampus-dependent spatial learning is associated with higher global cognition among healthy older adults. Neuropsychologia, 106, 310–321.
Korol, D. L., & Pisani, S. L. (2015). Estrogens and cognition: Friends or foe?: An evaluation of the opposing effects of estrogens on learning and memory. Hormones and Behavior, 74, 105–115.
Krupa, D. J., Thompson, J. K., & Thompson, R. F. (1993). Localization of a memory trace in the mammalian brain. Science, 260(5110), 989–991.
Lashley, K. S. (1929). Brain mechanisms and intelligence: A quantitative study of injuries to the brain. Chicago, IL: University of Chicago Press.
Lynch, G. (2002). Memory enhancement: The search for mechanism-based drugs. Nature Neuroscience, 5(Suppl.), 1035–1038.
Lynch, G., Larson, J., Staubli, U., Ambros-Ingerson, J., Granger, R., Lister, R. G., . . . Weingartner, H. J. (1991). Long-term potentiation and memory operations in cortical networks. In C. A. Wickliffe, M. Corballis, & G. White (Eds.), Perspectives on cognitive neuroscience (pp. 110–131). Oxford, England: Oxford University Press.
Maguire, E. A., Woollett, K., & Spiers, H. J. (2006). London taxi drivers and bus drivers: A structural MRI and neuropsychological analysis. Hippocampus, 16(12), 1091–1101.
Maki, P. M., & Resnick, S. M. (2000). Longitudinal effects of estrogen replacement therapy on PET cerebral blood flow and cognition. Neurobiology of Aging, 21, 373–383.
McDaniel, M. A., Maier, S. F., & Einstein, G. O. (2002). “Brain-specific” nutrients: A memory cure? Psychological Science in the Public Interest, 3(1), 12–38.
McEntee, W., & Crook, T. (1993). Glutamate: Its role in learning, memory, and the aging brain. Psychopharmacology, 111(4), 391–401.
McGaugh, J. L. (2003). Memory and emotion: The making of lasting memories. New York, NY: Columbia University Press.
McGaugh, J. L., Cahill, L, & Roozendaal, B. (1996). Involvement of the amygdala in memory storage: Interaction with other brainsystems. Proceedings of the National Academy of Sciences of the USA, 93(24), 13508–13514.
Paller, K. A. (2004). Electrical signals of memory and of the awareness of remembering. Current Directions in Psychological Science, 13(2), 49–55.
Rosenbaum, R. S., Köhler, S., Schacter, D., L., Moscovitch, M., Westmacott, R., Black, S. E., . . . Tulving, E. (2005). The case of K. C.: Contributions of a memory-impaired person to memory theory. Neuropsychologia, 43, 989–1021.
Sherwin, B. B. (1998). Estrogen and cognitive functioning in women. Proceedings of the Society for Experimental Biological Medicine, 217, 17–22.
Sigurdsson, T., Doyère, V., Cain, C. K., & LeDoux, J. E. (2007). Long-term potentiation in the amygdala: A cellular mechanism of fear learning and memory. Neuropharmacology, 52(1), 215–227.
Squire, L. R. (1992). Memory and the hippocampus: A synthesis from findings with rats, monkeys, and humans. Psychological Review, 99(2), 195–231.
Turner, D. C., & Sahakian, B. J. (2006). Analysis of the cognitive enhancing effects of modafinil in schizophrenia. In J. L. Cummings (Ed.), Progress in neurotherapeutics and neuropsychopharmacology (pp. 133–147). Cambridge, England: Cambridge University Press.
Woodruff-Pak, D. S., Goldenberg, G., Downey-Lamb, M. M., Boyko, O. B., & Lemieux, S. K. (2000). Cerebellar volume in humans related to magnitude of classical conditioning. Neuroreport: For Rapid Communication of Neuroscience Research, 11(3), 609–615.