13 3.2 Our Brains Control Our Thoughts, Feelings, and Behaviour
Learning Objectives
- Describe the structures and function of the brain.
- Explain the hemisphere and lobe structure of the cerebral cortex, and describe the function of each area of the cortex.
- Define the concepts of brain plasticity, neurogenesis, and brain lateralization.
The brains of all animals are very similar in overall form. In each animal, the brain is layered, and the basic structures of the brain are similar. The brain has three main regions: the hindbrain, the midbrain, and the forebrain. All three work together through complex connections and feedback loops. The hindbrain regulates basic survival functions such as breathing, moving, resting, and feeding. The midbrain sits atop the hindbrain and relays information between the hindbrain and other structures. The forebrain is the remaining part of the brain and is critical to providing more advanced functions that have evolved in humans, such as better memory, more sophisticated social interactions, and the ability to experience emotions. Humans have a very large and highly developed outer layer known as the cerebral cortex, which makes us particularly adept at these processes.
The old brain: Wired for survival
The hindbrain includes the brainstem, cerebellum, pons, reticular formation, and medulla. The brain stem is the oldest and innermost region of the brain. It is designed to control the most basic functions of life, including breathing, attention, and motor responses (see Figure 3.5). The brain stem begins where the spinal cord enters the skull and forms the medulla, which is the area of the brain stem that controls heart rate and breathing. In many cases, the medulla alone is sufficient to maintain life; animals that have the remainder of their brains above the medulla severed are still able to eat, breathe, and even move. The spherical shape above the medulla is the pons. This structure in the brain stem helps control movements of the body, such as chewing, and carries sensory information to other areas of the brain.
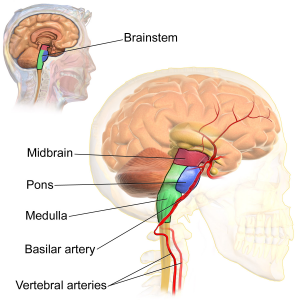
Running through the medulla and the pons is a long, narrow network of neurons known as the reticular formation. The job of the reticular formation is to filter out some of the stimuli that are coming into the brain from the spinal cord and to relay the remainder of the signals to other areas of the brain. The reticular formation also plays important roles in walking, eating, sexual activity, and sleeping. When electrical stimulation is applied to the reticular formation of an animal, it immediately becomes fully awake, and when the reticular formation is severed from the higher brain regions, the animal falls into a deep coma. The cerebellum, which means “little brain,” consists of two wrinkled ovals behind the brain stem and functions to coordinate voluntary movement. People who have damage to the cerebellum have difficulty walking, keeping their balance, and holding their hands steady. Consuming alcohol influences the cerebellum, which is why people who are drunk have more difficulty walking in a straight line. Also, the cerebellum contributes to emotional responses, helps us discriminate between different sounds and textures, and is important in learning (Bower & Parsons, 2003).
The midbrain sits above the hindbrain and relays sensory and motor information from the hindbrain to the forebrain, and vice versa. A layer of neurons within the midbrain called the substantia nigra is an important producer of the neurotransmitter dopamine, and also coordinates movement; this area is damaged in Parkinson’s disease.
Above the midbrain sits the forebrain, known as the cerebrum. Thought, planning, and language all happen in the cerebrum, as does the integration of sensory information. We make sense of the world in the cerebrum. The cerebrum contains many structures and is covered with a wrinkled layer called the cortex.
The thalamus is the egg-shaped structure above the brain stem that applies still more filtering to the sensory information that is coming up from the spinal cord and through the reticular formation, and it relays some of these remaining signals to the higher brain levels (Sherman & Guillery, 2006). The thalamus also receives some of the higher brain’s replies, forwarding them to the medulla and the cerebellum. The thalamus is also important in sleep because it shuts off incoming signals from the senses, allowing us to rest.
Whereas the primary function of the brain stem is to regulate the most basic aspects of life, including motor functions, the limbic system is largely responsible for memory and emotions, including our responses to reward and punishment. The limbic system is a brain area, located between the brain stem and the two cerebral hemispheres, that governs emotion and memory (see Figure 3.6). It includes the amygdala, the hypothalamus, and the hippocampus.
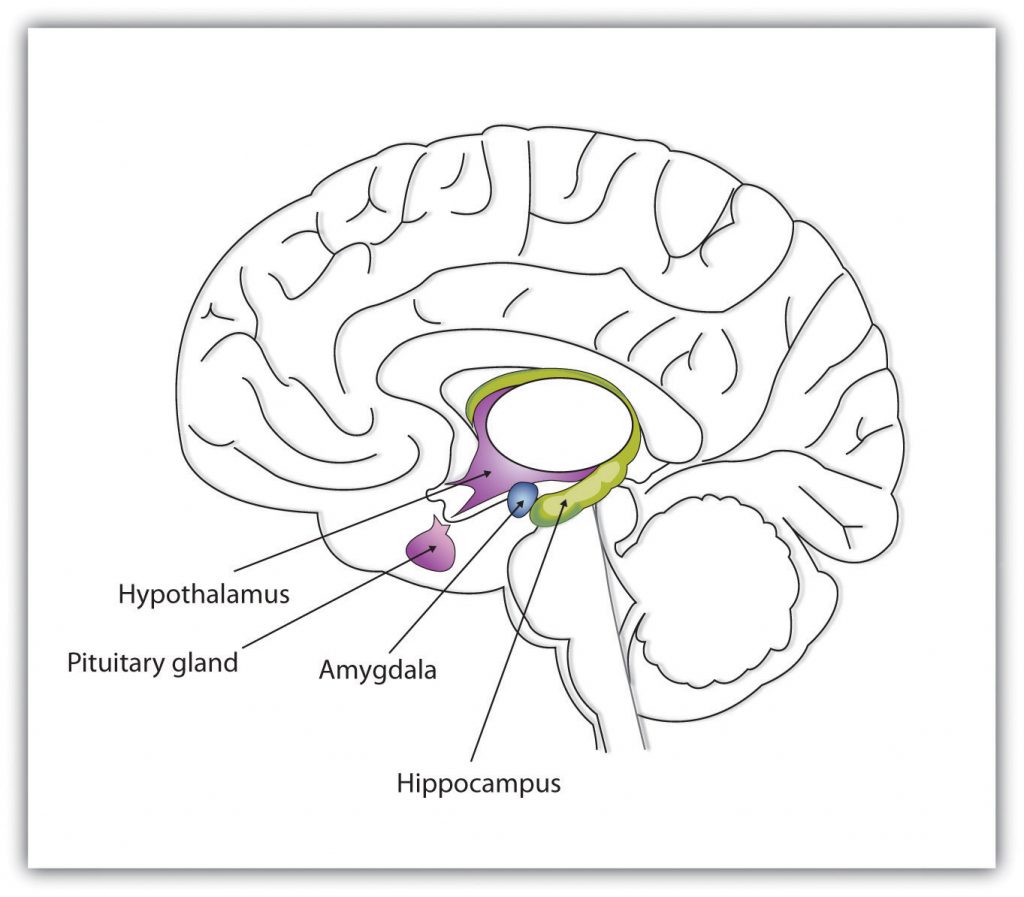
The amygdala, which comes from the Latin word for almond, consists of two “almond-shaped” clusters, and it is primarily responsible for regulating our perceptions of, and reactions to, aggression and fear. The amygdala has connections to other bodily systems related to fear, including the sympathetic nervous system, which is important in fear responses, facial responses to perceive and express emotions, the processing of smells, and the release of neurotransmitters related to stress and aggression (Best, 2009). In one early study, Heinrich Klüver and Paul Bucy (1939) damaged the amygdala of an aggressive rhesus monkey. They found that the once angry animal immediately became passive and no longer responded to fearful situations with aggressive behaviour. Electrical stimulation of the amygdala in other animals also influences aggression. In addition to helping us experience fear, the amygdala also helps us learn from situations that create fear. When we experience events that are dangerous, the amygdala stimulates the brain to remember the details of the situation so that we learn to avoid it in the future (Sigurdsson, Doyère, Cain, & LeDoux, 2007).
Located just under the thalamus, the location for which it is named, the hypothalamus is a brain structure that contains a number of small areas that perform a variety of functions, including the regulation of hunger and sexual behaviour, and it links the nervous system to the endocrine system via the pituitary gland. Through its many interactions with other parts of the brain, the hypothalamus helps regulate body temperature, hunger, thirst, and sex; it responds to the satisfaction of these needs by creating feelings of pleasure. James Olds and Peter Milner (1954) discovered these reward centres accidentally after they had momentarily stimulated the hypothalamus of a rat. The researchers noticed that after being stimulated, the rat continued to move to the exact spot in its cage where the stimulation had occurred, as if it were trying to re-create the circumstances surrounding its original experience. Upon further research into these reward centres, Olds (1958) discovered that animals would do almost anything to re-create enjoyable stimulation, including crossing a painful electrified grid to receive it. In one experiment, a rat was given the opportunity to electrically stimulate its own hypothalamus by pressing a pedal. The rat enjoyed the experience so much that it pressed the pedal more than 7,000 times per hour until it collapsed from sheer exhaustion.
The hippocampus consists of two “horns” that curve back from the amygdala. The hippocampus is important in storing information in long-term memory. If the hippocampus is damaged, a person cannot build new memories, living instead in a strange world where everything they experience just fades away, even while older memories from the time before the damage are untouched.
The cerebral cortex
The key to the advanced intelligence of humans is not found in the size of our brains. What sets humans apart from other animals is our larger cerebral cortex, which is the outer, bark-like layer of our brain that allows us to so successfully use language, acquire complex skills, create tools, and live in social groups (Gibson, 2002). In humans, the cerebral cortex is wrinkled and folded, rather than smooth as it is in most other animals. This creates a much greater surface area and size, and it allows increased capacities for learning, remembering, and thinking. The folding of the cerebral cortex is referred to as corticalization.
Although the cortex is only about one-tenth of an inch (2.5 mm) thick, it makes up more than 80% of the brain’s weight. The cortex contains about 20 billion nerve cells and 300 trillion synaptic connections (de Courten-Myers, 1999). Supporting all these neurons are billions more glial cells; these are cells that surround and link to the neurons, protecting them, providing them with nutrients and absorbing unused neurotransmitters. The glia come in different forms and have different functions. For instance, the myelin sheath surrounding the axon of many neurons is a type of glial cell. The glia are essential partners of neurons, without which the neurons could not survive nor function (Miller, 2005).
The cerebral cortex is divided into two hemispheres, and each hemisphere is divided into four lobes, each separated by folds known as fissures. If we look at the cortex starting at the front of the brain and moving over the top (see Figure 3.7), we see first the frontal lobe, located behind the forehead, which is responsible primarily for thinking, planning, memory, and judgment. Following the frontal lobe is the parietal lobe, located from the middle to the back of the skull, which is responsible primarily for processing information about touch. Next comes the occipital lobe, located at the very back of the skull, which processes visual information. Finally, located in front of the occipital lobe and between the ears, is the temporal lobe, which is responsible primarily for hearing and language.
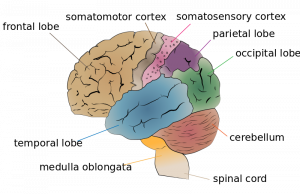
German physicists Gustav Fritsch and Eduard Hitzig (1870/2009) found that the movement that followed the brain stimulation only occurred when they stimulated a specific arch-shaped region that runs across the top of the brain from ear to ear, just at the front of the parietal lobe (see Figure 3.8). Fritsch and Hitzig had discovered the motor cortex, which is the part of the cortex that controls and executes movements of the body by sending signals to the cerebellum and the spinal cord. More recent research has mapped the motor cortex even more fully by providing mild electronic stimulation to different areas of the motor cortex in fully conscious patients while observing their bodily responses; because the brain has no sensory receptors, these patients feel no pain. This research has revealed that the motor cortex is specialized for providing control over the body, in the sense that the parts of the body that require more precise and finer movements, such as the face and the hands, also are allotted the greatest amount of cortical space.
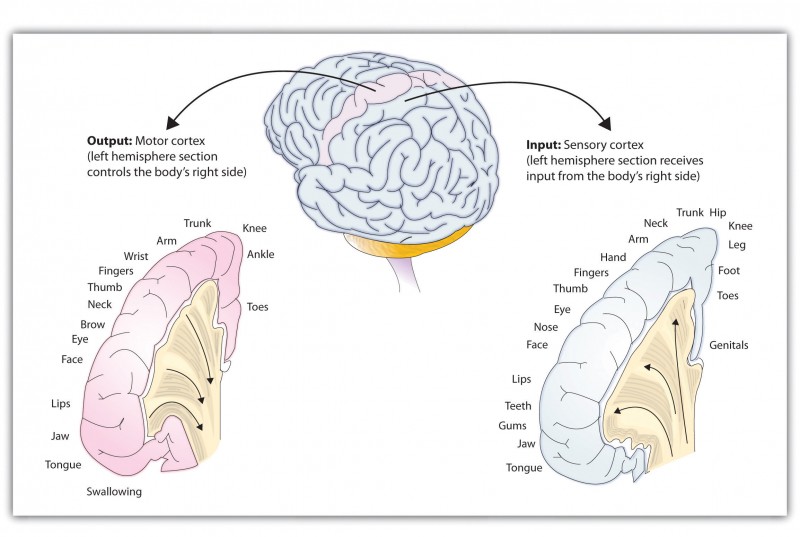
Just as the motor cortex sends out messages to the specific parts of the body, the somatosensory cortex, which is located just behind and parallel to the motor cortex at the back of the frontal lobe, receives information from the skin’s sensory receptors and the movements of different body parts. Again, the more sensitive the body region, the more area is dedicated to it in the sensory cortex. Our sensitive lips, for example, occupy a large area in the sensory cortex, as do our fingers and genitals.
Other areas of the cortex process other types of sensory information. The visual cortex is the area located in the occipital lobe at the very back of the brain that processes visual information. If you were stimulated in the visual cortex, you would see flashes of light or colour, and perhaps you remember having had the experience of “seeing stars” when you were hit in, or fell on, the back of your head. The temporal lobe, located on the lower side of each hemisphere, contains the auditory cortex, which is responsible for hearing and language. The temporal lobe also processes some visual information, providing us with the ability to name the objects around us (Martin, 2007).
The motor and sensory areas of the cortex account for a relatively small part of the total cortex. The remainder of the cortex is made up of association areas in which sensory and motor information is combined and associated with our stored knowledge. These association areas are the places in the brain that are responsible for most of the things that make human beings seem human. The association areas are involved in higher mental functions, such as learning, thinking, planning, judging, moral reflecting, figuring, and spatial reasoning.
Lateralization
When Fritsch and Hitzig (1870/2009) applied mild electric stimulation to different parts of a dog’s cortex, they discovered that they could make different parts of the dog’s body move. Furthermore, they discovered an important and unexpected principle of brain activity. They found that stimulating the right side of the brain produced movement in the left side of the dog’s body, and vice versa. This finding follows from a general principle about how the brain is structured, called contralateral control, meaning the brain is wired such that in most cases the left hemisphere receives sensations from and controls the right side of the body, and the right hemisphere communicates in the same way with the left side. Vision processing works a little differently. The left half of the visual field from each eye is processed in the right hemisphere, while information from the right halves of the visual fields of both eyes goes to the left hemisphere.
Interestingly, while both hemispheres of the brain work together when we are thinking or processing information, the right and left sides have different specializations. Processing of language and analytical-mathematical reasoning occurs mostly in the left hemisphere, while processing of visuospatial imagery and music occurs mostly in the right hemisphere. However, characterizing people as being “left-brained” or “right-brained” shows a misunderstanding of the complex interactions between the hemispheres that takes place during all tasks. It is not possible to reduce thinking or understanding to simply the work of one hemisphere. See the Research Focus below for a discussion of the research that produced our knowledge about specializations of the right and left hemispheres.
The brain is flexible: Neuroplasticity
The control of some specific bodily functions, such as movement, vision, and hearing, is performed in specified areas of the cortex. If these areas are damaged, the individual will likely lose the ability to perform the corresponding function. For instance, if an infant suffers damage to facial recognition areas in the temporal lobe, it is likely that they will never be able to recognize faces (Farah, Rabinowitz, Quinn, & Liu, 2000). On the other hand, the brain is not divided up in an entirely rigid way. The brain’s neurons have a remarkable capacity to reorganize and extend themselves to carry out particular functions in response to the needs of the organism and to repair damage. As a result, the brain constantly creates new neural communication routes and rewires existing ones. Neuroplasticity refers to the brain’s ability to change its structure and function in response to experience or damage. Neuroplasticity enables us to learn and remember new things and adjust to new experiences.
Our brains are the most “plastic” when we are young children, as it is during this time that we learn the most about our environment. On the other hand, neuroplasticity continues to be observed even in adults (Kolb & Fantie, 1989). The principles of neuroplasticity help us understand how our brains develop to reflect our experiences. For instance, accomplished musicians have a larger auditory cortex compared with the general population (Bengtsson et al., 2005) and also require less neural activity to move their fingers over the keys than do novices (Münte, Altenmüller, & Jäncke, 2002). These observations reflect the changes in the brain that follow our experiences.
Plasticity is also observed when there is damage to the brain or to parts of the body that are represented in the motor and sensory cortexes. When a tumour in the left hemisphere of the brain impairs language, the right hemisphere will begin to compensate to help the person recover the ability to speak (Thiel et al., 2006). If a person loses a finger, the area of the sensory cortex that previously received information from the missing finger will begin to receive input from adjacent fingers, causing the remaining digits to become more sensitive to touch (Fox, 1984).
Although neurons cannot repair or regenerate themselves as skin or blood vessels can, new evidence suggests that the brain can engage in neurogenesis, which is the forming of new neurons (van Praag, Zhao, Gage, & Gazzaniga, 2004). These new neurons originate deep in the brain and may then migrate to other areas of the brain where they form new connections with other neurons (Gould, 2007). This leaves open the possibility that someday scientists might be able to rebuild damaged brains by creating drugs that help grow neurons.
Research Focus
Identifying the unique functions of the left and right hemispheres using split-brain patients
We have seen that the left hemisphere of the brain primarily senses and controls the motor movements on the right side of the body, and vice versa. This fact provides an interesting way to study brain lateralization, which is the idea that the left and the right hemispheres of the brain are specialized to perform different functions. Michael Gazzaniga, Joseph Bogen, and Roger Sperry (1965) studied a patient, known as W. J., who had undergone an operation to relieve severe seizures. In this surgery, the region that normally connects the two halves of the brain and supports communication between the hemispheres, known as the corpus callosum, is severed. As a result, the patient essentially becomes a person with two separate brains. Because the left and right hemispheres are separated, each hemisphere develops a mind of its own, each with its own sensations, concepts, and motivations (Gazzaniga, 2005).
In their research, Gazzaniga, Bogen, and Sperry tested the ability of W. J. to recognize and respond to objects and written passages that were presented to only the left or to the right brain hemispheres. The researchers had W. J. look straight ahead and then flashed, for a fraction of a second, a picture of a geometrical shape to the left of where he was looking. By doing so, they ensured that — because the two hemispheres had been separated — the image of the shape was experienced only in the right brain hemisphere because sensory input from the left side of the body is sent to the right side of the brain. They found that W. J. was able to identify what had been shown when asked to pick the object from a series of shapes with the left hand but that this was not possible when the object was shown in the right visual field. In contrast, W. J. could easily read written material presented in the right visual field, which was experienced in the left hemisphere, but not when it was presented in the left visual field.
This research, and many other studies following it, has demonstrated that the two brain hemispheres specialize in different abilities. In most people, the ability to speak, write, and understand language is located in the left hemisphere. This is why W. J. could read passages that were presented on the right side, which were transmitted to the left hemisphere, but could not read passages that were only experienced in the right brain hemisphere. The left hemisphere is also better at math and at judging time and rhythm. It is also superior in coordinating the order of complex movements, such as lip movements needed for speech. The right hemisphere, in contrast, has only very limited verbal abilities, yet it excels in perceptual skills. The right hemisphere is able to recognize objects, including faces, patterns, and melodies, and it can put a puzzle together or draw a picture. This is why W. J. could pick out the image when presented on the left, but not the right, visual field.
Although this research demonstrated that the brain is in fact lateralized, such that the two hemispheres specialize in different activities, this does not mean that when people behave in a certain way or perform a certain activity they are only using one hemisphere of their brains at a time. That would be drastically oversimplifying the concept of brain hemisphere differences. We normally use both hemispheres at the same time, and the difference between the abilities of the two hemispheres is not absolute (Soroker et al., 2005).
The following Public Broadcasting Service (PBS) episode of Scientific American Frontiers vividly demonstrates Gazzaniga’s research:
- Video: Pieces of Mind (Chedd-Angier Production Company, 1997)
Psychology in Everyday Life
Why are some people left-handed?
Across cultures and ethnic groups, about 90% of people are mainly right-handed, whereas only 10% are primarily left-handed (Peters, Reimers, & Manning, 2006). This fact is puzzling, partly because the number of left-handers is so low and partly because other animals, including our closest primate relatives, do not show any type of “handedness.” The existence of right-handers and left-handers provides an interesting example of the relationship among evolution, biology, and social factors as well as how the same phenomenon can be understood at different levels of analysis (Harris, 1990; McManus, 2002).
At least some handedness is determined by genetics. Ultrasound scans show that nine out of ten fetuses suck the thumb of their right hand, suggesting that the preference is determined before birth (Hepper, Wells, & Lynch, 2005), and the mechanism of transmission has been linked to a gene on the X chromosome (Jones & Martin, 2000). It has also been observed that left-handed people are likely to have fewer children, and this may be, in part, because the mothers of left-handers are more prone to miscarriages and other prenatal problems (McKeever, Cerone, Suter, & Wu, 2000).
However, culture also plays a role. In the past, left-handed children were forced to write with their right hands in many countries, and this practice continues, particularly in collectivistic cultures, such as India and Japan, where left-handedness is viewed negatively as compared with individualistic societies, such as Canada and the United States. For example, India has about half as many left-handers as the United States (Ida & Mandal, 2003).
There are both advantages and disadvantages to being left-handed in a world where most people are right-handed. One problem for lefties is that the world is designed for right-handers. Automated teller machines (ATMs), classroom desks, scissors, microscopes, drill presses, and table saws are just some examples of everyday machinery designed with the most important controls on the right side. This may explain, in part, why left-handers suffer somewhat more accidents than do right-handers (Dutta & Mandal, 2006).
Despite the potential difficulty living and working in a world designed for right-handers, there seem to be some advantages to being left-handed. Throughout history, a number of prominent artists have been left-handed, including Leonardo da Vinci, Michelangelo, Pablo Picasso, and Max Escher. Because the right hemisphere is superior in imaging and visual abilities, there may be some advantage to using the left hand for drawing or painting (Springer & Deutsch, 1998). Left-handed people are also better at envisioning three-dimensional objects, which may explain why there is such a high number of left-handed architects, artists, and chess players in proportion to their numbers (Coren, 1992). However, there are also more left-handers among those with reading disabilities, allergies, and migraine headaches (Geschwind & Behan, 2007), perhaps due to the fact that a small minority of left-handers owe their handedness to a birth trauma, such as being born prematurely (Betancur, Vélez, Cabanieu, & le Moal, 1990).
In sports in which handedness may matter, such as tennis, boxing, fencing, or judo, left-handers may have an advantage. They play many games against right-handers and learn how to best handle their styles. Right-handers, however, play very few games against left-handers, which may make them more vulnerable. This explains why a disproportionately high number of left-handers are found in sports where direct one-on-one action predominates. In other sports, such as golf, there are fewer left-handed players because the handedness of one player has no effect on the competition.
The fact that left-handers excel in some sports suggests the possibility that they may have also had an evolutionary advantage because their ancestors may have been more successful in important skills such as hand-to-hand combat (Bodmer & McKie, 1994). At this point, however, this idea remains only a hypothesis, and determinants of human handedness are yet to be fully understood.
Key Takeaways
- The brain is divided into hindbrain, midbrain, and forebrain structures that must work together.
- The cerebral cortex, made up of billions of neurons and glial cells, is divided into the right and left hemispheres, and these are further divided into four lobes.
- The frontal lobe is primarily responsible for thinking, planning, memory, and judgment. The parietal lobe is primarily responsible for bodily sensations and touch. The temporal lobe is primarily responsible for hearing and language. The occipital lobe is primarily responsible for vision. Other areas of the cortex act as association areas, responsible for integrating information.
- The brain changes as a function of experience and potential damage in a process known as plasticity. The brain can generate new neurons through neurogenesis.
- The motor cortex controls voluntary movements. Body parts requiring the most control and dexterity take up the most space in the motor cortex.
- The sensory cortex receives and processes bodily sensations. Body parts that are the most sensitive occupy the greatest amount of space in the sensory cortex.
- The left cerebral hemisphere is primarily responsible for language and speech in most people, whereas the right hemisphere specializes in spatial and perceptual skills, visualization, and the recognition of patterns, faces, and melodies.
- The severing of the corpus callosum, which connects the two hemispheres, creates a “split-brain patient,” with the effect of creating two separate minds operating in one person.
- Studies with split-brain patients as research participants have been used to study brain lateralization.
- Neuroplasticity allows the brain to adapt and change as a function of experience or damage.
Exercises and Critical Thinking
- Do you think that animals experience emotion? What aspects of brain structure might lead you to believe that they do or do not?
- Consider your own experiences and speculate on which parts of your brain might be particularly well-developed as a result of these experiences.
- Which brain hemisphere are you likely to be using when you search for a fork in the kitchen? Which brain hemisphere are you most likely to be using when you struggle to remember the name of an old friend?
- Do you think that encouraging a left-handed child to use their right hand is a good idea? Why or why not?
Image Attributions
Figure 3.5. The Brainstem by BruceBlaus is used under a CC BY 3.0 license.
Figure 3.6. Used under a CC BY-NC-SA 4.0 license.
Figure 3.7. Cerebrum Lobes by Jkwchui is used under a CC BY-SA 3.0 license.
Figure 3.8. Used under a CC BY-NC-SA 4.0 license.
References
Bengtsson, S. L., Nagy, Z., Skare, S., Forsman, L., Forssberg, H., & Ullén, F. (2005). Extensive piano practicing has regionally specific effects on white matter development. Nature Neuroscience, 8(9), 1148–1150.
Best, B. (2009). The anatomical basis of mind. Retrieved from http://www.benbest.com/science/anatmind/anatmind.html
Betancur, C., Vélez, A., Cabanieu, G., & le Moal, M. (1990). Association between left-handedness and allergy: A reappraisal. Neuropsychologia, 28(2), 223–227.
Bodmer, W., & McKie, R. (1994). The book of man: The quest to discover our genetic heritage. London, England: Little, Brown and Company.
Bower, J. M., & Parsons, J. M. (2003). Rethinking the lesser brain. Scientific American, 289, 50–57.
Chedd-Angier Production Company. (1997, January 22). Pieces of mind [Television series episode]. In Scientific American Frontiers. Retrieved from http://www.chedd-angier.com/video/SAF/SAF_703.webm
Coren, S. (1992). The left-hander syndrome: The causes and consequences of left-handedness. New York, NY: Free Press.
de Courten-Myers, G. M. (1999). The human cerebral cortex: Gender differences in structure and function. Journal of Neuropathology and Experimental Neurology, 58, 217–226.
Dutta, T., & Mandal, M. K. (2006). Hand preference and accidents in India. Laterality: Asymmetries of Body, Brain, and Cognition, 11, 368–372.
Farah, M. J., Rabinowitz, C., Quinn, G. E., & Liu, G. T. (2000). Early commitment of neural substrates for face recognition. Cognitive Neuropsychology, 17(1–3), 117–123.
Fox, J. L. (1984). The brain’s dynamic way of keeping in touch. Science, 225(4664), 820–821.
Fritsch, G., & Hitzig, E. (1870/2009). Electric excitability of the cerebrum (Über die Elektrische erregbarkeit des Grosshirns). Epilepsy & Behavior, 15(2), 123–130. (Original work published 1870)
Gazzaniga, M. S. (2005). Forty-five years of split-brain research and still going strong. Nature Reviews Neuroscience, 6(8), 653–659.
Gazzaniga, M. S., Bogen, J. E., & Sperry, R. W. (1965). Observations on visual perception after disconnexion of the cerebral hemispheres in man. Brain, 88(2), 221–236.
Geschwind, N., & Behan, P. (2007). Left-handedness: Association with immune disease, migraine, and developmental learning disorder. Cambridge, MA: MIT Press.
Gibson, K. R. (2002). Evolution of human intelligence: The roles of brain size and mental construction. Brain Behavior and Evolution 59, 10–20.
Gould, E. (2007). How widespread is adult neurogenesis in mammals? Nature Reviews Neuroscience 8, 481–488.
Harris, L. J. (1990). Cultural influences on handedness: Historical and contemporary theory and evidence. In S. Coren (Ed.), Advances in psychology, Vol. 67. Left-handedness: Behavioral implications and anomalies (pp. 195–258). Oxford, England: North-Holland.
Hepper, P. G., Wells, D. L., & Lynch, C. (2005). Prenatal thumb sucking is related to postnatal handedness. Neuropsychologia, 43, 313–315.
Ida, Y., & Mandal, M. K. (2003). Cultural differences in side bias: Evidence from Japan and India. Laterality: Asymmetries of Body, Brain, and Cognition, 8(2), 121–133.
Jones, G. V., & Martin, M. (2000). A note on Corballis (1997) and the genetics and evolution of handedness: Developing a unified distributional model from the sex-chromosomes gene hypothesis. Psychological Review, 107(1), 213–218.
Klüver, H., & Bucy, P. C. (1939). Preliminary analysis of functions of the temporal lobes in monkeys. Archives of Neurology & Psychiatry (Chicago), 42, 979–1000.
Kolb, B., & Fantie, B. (1989). Development of the child’s brain and behavior. In C. R. Reynolds & E. Fletcher-Janzen (Eds.), Handbook of clinical child neuropsychology (pp. 17–39). New York, NY: Plenum Press.
Martin, A. (2007). The representation of object concepts in the brain. Annual Review of Psychology, 58, 25–45.
McKeever, W. F., Cerone, L. J., Suter, P. J., & Wu, S. M. (2000). Family size, miscarriage-proneness, and handedness: Tests of hypotheses of the developmental instability theory of handedness. Laterality: Asymmetries of Body, Brain, and Cognition, 5(2), 111–120.
McManus, I. C. (2002). Right hand, left hand: The origins of asymmetry in brains, bodies, atoms, and cultures. Cambridge, MA: Harvard University Press.
Miller, G. (2005). Neuroscience: The dark side of glia. Science, 308(5723), 778–781.
Münte, T. F., Altenmüller, E., & Jäncke, L. (2002). The musician’s brain as a model of neuroplasticity. Nature Reviews Neuroscience, 3(6), 473–478.
Olds, J. (1958). Self-stimulation of the brain: Its use to study local effects of hunger, sex, and drugs. Science, 127, 315–324.
Olds, J., & Milner, P. (1954). Positive reinforcement produced by electrical stimulation of septal area and other regions of rat brain. Journal of Comparative and Physiological Psychology, 47, 419–427.
Peters, M., Reimers, S., & Manning, J. T. (2006). Hand preference for writing and associations with selected demographic and behavioral variables in 255,100 subjects: The BBC Internet study. Brain and Cognition, 62(2), 177–189.
Sherman, S. M., & Guillery, R. W. (2006). Exploring the thalamus and its role in cortical function (2nd ed.). Cambridge, MA: MIT Press.
Sigurdsson, T., Doyère, V., Cain, C. K., & LeDoux, J. E. (2007). Long-term potentiation in the amygdala: A cellular mechanism of fear learning and memory. Neuropharmacology, 52(1), 215–227.
Soroker, N., Kasher, A., Giora, R., Batori, G., Corn, C., Gil, M., & Zaidel, E. (2005). Processing of basic speech acts following localized brain damage: A new light on the neuroanatomy of language. Brain and Cognition, 57(2), 214–217.
Springer, S. P., & Deutsch, G. (1998). Left brain, right brain: Perspectives from cognitive neuroscience (5th ed.). New York, NY: W. H. Freeman/Times Books/Henry Holt & Co.
Thiel, A., Habedank, B., Herholz, K., Kessler, J., Winhuisen, L., Haupt, W. F., & Heiss, W. D. (2006). From the left to the right: How the brain compensates progressive loss of language function. Brain and Language, 98(1), 57–65.
van Praag, H., Zhao, X., Gage, F. H., & Gazzaniga, M. S. (2004). Neurogenesis in the adult mammalian brain. In The cognitive neurosciences (3rd ed., pp. 127–137). Cambridge, MA: MIT Press.