Proteins
Proteins are chains of amino acid monomers linked by covalent bonds called peptide bonds folded into a specific shape. The linear chain of amino acids may be referred to as a peptide. Chains with few amino acids (i.e. less than 50 amino acids) are called oligopeptides. Proteins that consist of multiple peptide chains are known as polypeptides. Some proteins consist of a single peptide chain. Some proteins consist of many peptides.
There are 20 naturally occurring amino acids. Every amino acid consists of a central carbon atom covalently bonded to four groups: a hydrogen, an amino group (-NH2), a carboxyl group (-COOH), and a reactivity or R group. The R groups are unique to each amino acid. R groups may be nonpolar and hydrophobic, polar and hydrophilic, or charged and hydrophilic. When the amino acids are joined by dehydration synthesis into a growing peptide, the amino groups always face one direction, and the carboxyl groups always face the opposite direction. As a result, every protein has an amino end or N-terminus and a carboxyl end or C-terminus. The N-terminus is considered the “start” of the protein and the C-terminus is considered the “end”.
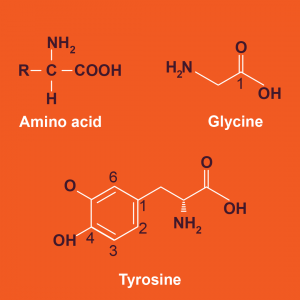
Protein structure can be organized into four levels of complexity.
- Primary protein structure is simply the order or sequence of amino acids within the peptide chain(s). The covalent peptide bond stabilizes primary protein structure.
- Secondary protein structure is the formation of hydrogen bonds between amino acids within a peptide chain, forming either a coil called an α-helix or a flat structure called a β-sheet. Peptides may contain both types of secondary structural features or only one type.
- Tertiary protein structure is the arrangement of the peptide chains in space. For most proteins, hydrophobic interactions drive proteins to “hide” hydrophobic protein surfaces from the surrounding aqueous solution. However, hydrogen bonds and covalent bonds can also stabilize tertiary protein structure. For example, the amino acid cysteine contains a sulfhydryl group (-SH) that can form strong covalent bonds called disulfide bonds with another cysteine. Disulfide bonds are important to the tertiary structure of some proteins. In all proteins, tertiary structure is how the α-helices or a β-sheets within single peptide chains are arranged relative to one another in three-dimensional (3-D) space.
- Finally, quaternary protein structure is the arrangement of different peptides relative to one another in 3-D space. Weak noncovalent interactions such as hydrogen bonds or strong covalent bonds such as disulfide bonds may stabilize quaternary protein structure, depending on the amino acid sequence of that protein. Importantly, not all proteins have quaternary protein structure because some peptides consist of only one peptide chain. In proteins that are only a single peptide chain, the highest level of protein structure these proteins achieve is tertiary protein structure.
Let us examine the different levels of protein structure in a human protein. Hemoglobin is a protein synthesized by red blood cells that binds and transports oxygen in blood. Hemoglobin is a polypeptide consisting of four peptides: two identical α-globin peptides and two identical β-globin peptides. The α- and β-globin peptides differ in primary structure or amino acid sequence. Both globins are mostly α-helical proteins and, therefore, have similar secondary structures. Each globin folds in a unique manner dictated by the chemical nature of the amino acids within each peptide. However, both globin subunits must fold to accommodate an iron-containing heme group that contains the oxygen-binding site. The folding of each globin into a shape that is covalently bound to heme is the tertiary structure of each peptide. Because hemoglobin is a polypeptide, this protein has quaternary structure. The quaternary structure of hemoglobin is the arrangement of the four globin molecules in space.
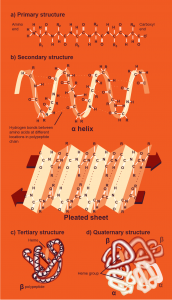
The structure of proteins determines their function. For example, the function of hemoglobin is to transport oxygen in blood. This function is only possible because the tertiary structure of hemoglobin permits oxygen-binding to occur at the covalently bound heme molecule. Additionally, because each hemoglobin protein can bind a maximum of four oxygen molecules, this permits a large volume of oxygen to be transported in hemoglobin at a time. The shape of proteins determines what molecules the protein can interact with, including other proteins. The final shape or fold of a protein that permits cellular function is known as the protein’s native fold.
Changes to environmental conditions can alter protein folding and, therefore, protein function. For example, changes to the pH of the surrounding solution can disrupt the hydrogen bonds that stabilize secondary, tertiary, and/or quaternary protein structure. When the native fold of proteins is lost or disrupted, proteins are denatured. Denatured proteins are biologically inactive because they can no longer interact with other cellular molecules due to their altered structure. Note that the peptide bonds that link amino acids within denatured proteins remains intact.
Proteins are diverse molecules in cells that perform many cellular functions. Recall that enzymes are proteins that can perform chemical reactions. All the reactions that help extract energy from food we digest in the small intestine are performed by cellular enzymes. Protective structures such as hair and nails consist of an abundant protein called keratin. Keratin is a fibrous protein, meaning it forms an extended thread-like structure that is not soluble in water. In contrast, the protein hemoglobin is a globular protein, meaning it forms a compact structure that is generally soluble in water. Proteins are also found protruding from cell membranes as receptors for signals such as hormones or neurotransmitters. Proteins embedded in cell membranes that move ions across the cell membrane permit nerve and muscle cells to send and receive signals, respectively. Clearly, proteins perform critical cellular functions and this is only possible because of their unique structures.