Unit 7: The Digestive System
Unit outline
Part 1: Overview of the Digestive System
Part 2: Digestive System Processes and Regulation
Part 3: The Mouth, Pharynx, and Esophagus
Part 5: The Small and Large Intestines
Part 6: Accessory Organs in Digestion: The Liver, Pancreas, and Gallbladder
Part 8: Chemical Digestion and Absorption: A Closer Look
- Chemical Digestion
- Carbohydrate Digestion
- Protein Digestion
- Lipid Digestion
- Nucleic Acid Digestion
- Absorption
Practice Questions
Learning Objectives
At the end of this unit, you should be able to:
I. Describe the major functions of the digestive system.
II. Describe the relationship between the following processes in the gastrointestinal system: ingestion, digestion, absorption, defecation.
III. Distinguish between extracellular digestion and intracellular digestion.
IV. Describe the anatomy of the buccal cavity and explain its functions in digestion.
V. Describe the process of deglutition (swallowing), explaining why food, when swallowed, does not enter the respiratory tract or the nasal cavity.
VI. Describe the anatomy and functions of the esophagus.
VII. Describe the anatomy and functions of the stomach.
VIII. Describe the liver with reference to: anatomy, function, connection to the duodenum and gallbladder, blood supply.
IX. Describe the anatomy and functions of the pancreas.
X. Describe the anatomy and functions of the small intestine.
XI. Describe the anatomy and functions of the large intestine.
XII. Describe the process of defecation
XIII. Specify five essential nutritional factors.
XIV. Describe the chemical digestion of the following, specifying the source and the function of the principal enzymes involved: carbohydrates, proteins, lipids, nucleic acids.
XV. Specify the end-products of the digestion of the following and explain how they are absorbed: carbohydrates, proteins, lipids, nucleic acids.
XVI. Describe the control of the secretion of digestive juices in humans in terms of: nervous control, hormonal control.
Learning Objectives and Guiding Questions
At the end of this unit, you should be able to complete all the following tasks, including answering the guiding questions associated with each task.
I. Describe the major functions of the digestive system.
- Describe the six major processes occurring during digestive system activity, and list all the organs of the gastrointestinal tract that perform each one.
II. Describe the relationship between the following processes in the gastrointestinal system: ingestion, digestion, absorption, defecation.
- Clearly define each of the following terms as they relate to the gastrointestinal system:
- Ingestion
- Digestion
- Absorption
- Defecation
III. Distinguish between extracellular digestion and intracellular digestion.
- Describe and clearly distinguish between extracellular digestion and intracellular digestion and state the specific location(s) in the human body where extracellular digestion occurs.
IV. Describe the anatomy of the buccal cavity and explain its functions in digestion.
- Describe the anatomy of the buccal cavity, specifying the relative location and major tissue type(s) of each of the following structures:
- Lips
- Cheeks
- Hard palate
- Soft palate
- Uvula
- Teeth
- Tongue
- Salivary glands
- Describe how each of the following structures contributes to the food-related functions served by the buccal cavity:
- Lips
- Cheeks
- Hard palate
- Soft palate
- Uvula
- Teeth
- Tongue
- Salivary glands
V. Describe the process of deglutition (swallowing), explaining why food, when swallowed, does not enter the respiratory tract or the nasal cavity.
- Describe the process of deglutition in terms of its three major phases, describing the function and the neural control of each step.
VI. Describe the anatomy and functions of the esophagus.
- Describe the anatomy of the esophagus by using correct anatomical terms to describe:
- Its location in the human body.
- Its overall structure.
- The layers of tissue of which it is composed.
- Describe how each tissue layer of the esophagus contributes to the primary function of the esophagus.
VII. Describe the anatomy and functions of the stomach.
- Describe the anatomy of the stomach by using correct anatomical terms to describe:
- Its location in the human body.
- Its overall structure.
- The layers of tissue of which it is composed.
- Describe how each tissue layer of the stomach performs (or contributes to):
- Propulsion.
- Mechanical digestion.
- Chemical digestion.
- Name the four secretory cell types that make up each gastric gland. For each cell type, state the product(s) it secretes and the function of its product(s).
- Name one hormone secreted by the stomach, and state:
-
- Its specific site (tissue and/or cell type) of production.
- The stimulus for its production.
- In which organ its target cells are located.
- The effect(s) of its release.
VIII. Describe the liver with reference to: anatomy, function, connection to the duodenum and gallbladder, blood supply.
- Describe the anatomy of the liver by using correct anatomical terms to describe:
- Its location in the human body.
- Its connections to organs of the gastrointestinal tract and other accessory organs of the digestive system.
- Describe the function served by the liver as part of the digestive system.
- Describe the vasculature delivering blood to and from the liver, and explain how this vasculature relates to the functions served by the liver in the body.
IX. Describe the anatomy and functions of the pancreas.
- Describe the anatomy of the pancreas by using correct anatomical terms to describe:
- Its location in the human body.
- Its connections to organs of the gastrointestinal tract.
- Describe and distinguish between the endocrine and exocrine functions of the pancreas.
X. Describe the anatomy and functions of the small intestine.
- Describe the anatomy of the small intestine by using correct anatomical terms to describe:
- Its location in the human body.
- Its three main anatomical subdivisions.
- Its connections to other organs of the gastrointestinal tract, and to accessory organs of the digestive system.
- Explain in detail how the small intestine performs (or contributes to):
- Propulsion.
- Mechanical digestion.
- Chemical digestion.
- Absorption.
- Name two hormones secreted by the small intestine. For each hormone, state:
-
- The stimulus for its production.
- In which organ its target cells are located.
- The effect(s) of its release.
XI. Describe the anatomy and functions of the large intestine.
- Describe the anatomy of the large intestine by using correct anatomical terms to describe:
- Its location in the human body.
- Its main anatomical subdivisions.
- The layers of tissue of which it is composed.
- Its connections to other organs of the gastrointestinal tract.
- Explain in detail how the large intestine performs (or contributes to):
-
- Propulsion.
- Mechanical digestion.
- Chemical digestion.
- Absorption.
- Defecation.
XII. Describe the process of defecation
- Describe the process of defecation, explaining the function and the neural control of each step.
XIII. Specify five essential nutritional factors.
- Clearly explain the difference between an essential nutrient and a nonessential nutrient.
- Define vitamin and describe the general functions, categories and examples of vitamins.
-
- Define ‘vitamin’ and describe the general functions of vitamins.
- Explain how the main difference between lipid-soluble and water-soluble vitamins leads to differences in dietary requirements for each type.
- List all the water-soluble and fat-soluble vitamins, along with the primary function of each vitamin.
3. Define the term mineral and list six major minerals (macrominerals) with one function of each.
-
- Specify the six major minerals in humans and describe one major function of each.
- With the aid of specific examples, clearly distinguish between:
i. Minerals and vitamins
ii. Trace minerals and major minerals
XIV. Describe the chemical digestion of the following, specifying the source and the function of the principal enzymes involved: carbohydrates, proteins, lipids, nucleic acids.
XV. Specify the end-products of the digestion of the following and explain how they are absorbed: carbohydrates, proteins, lipids, nucleic acids.
- Describe the function of all the enzymes involved in carbohydrate digestion in the gastrointestinal tract. For each enzyme, state its name, source organ, site of action, substrate, and product.
- Name the organ in the gastrointestinal tract within which the majority of chemical digestion of carbohydrates occurs.
- Specify the end products of the carbohydrate digestion that occurs in the gastrointestinal tract.
- Explain where and how each end product of carbohydrate digestion ultimately is absorbed from the lumen of the gastrointestinal tract into the blood.
- Name the organ in the gastrointestinal tract within which the majority of chemical digestion of proteins occurs.
- Specify the end products of the protein digestion that occurs in the gastrointestinal tract.
- Explain where and how each end product of protein digestion ultimately is absorbed from the lumen of the gastrointestinal tract into the blood.
- Describe the function of all the enzymes involved in lipid digestion in the gastrointestinal tract. For each enzyme, state its name, source organ, site of action, substrate, and product.
- Name the organ in the gastrointestinal tract within which the majority of chemical digestion of lipids occurs.
- Specify the end products of the lipid digestion that occurs in the gastrointestinal tract. Explain where and how each end product ultimately is absorbed from the lumen of the gastrointestinal tract into the blood.
- Describe the type(s) of molecules that the end products of lipid digestion can be reassembled into, and what other functions they might serve.
- Describe the function of all the enzymes involved in nucleic acid digestion in the gastrointestinal tract. For each enzyme, state its name, source organ, site of action, substrate, and product.
- Name the organ in the gastrointestinal tract within which the majority of chemical digestion of nucleic acids occurs.
- Specify the end products of the nucleic acid digestion that occurs in the gastrointestinal tract.
- Explain where and how each end product of nucleic acid digestion ultimately is absorbed from the lumen of the gastrointestinal tract into the blood.
- Describe the type(s) of molecules that the end products of nucleic acid digestion can be reassembled into, and what other functions they might serve.
XVI. Describe the control of the secretion of digestive juices in humans in terms of: nervous control, hormonal control.
- Describe the pathways by which the nervous system regulates:
- Gastric secretory activity during the cephalic phase of gastric secretion.
- Gastric secretory activity during the gastric phase of gastric secretion.
- Gastric secretory activity during the intestinal phase of gastric secretion.
- Describe the hormonal regulation of:
-
- Gastric secretory activity during the gastric phase of gastric secretion.
- Gastric secretory activity during the intestinal phase of gastric secretion.
- Bile production and release.
- Pancreatic juice production and release.
The digestive system is continually at work, yet people seldom appreciate the complex tasks it performs in a choreographed biologic symphony. Consider what happens when you eat an apple. Of course, you enjoy the apple’s taste as you chew it, but in the hours that follow, unless something goes amiss and you get a stomachache, you don’t notice that your digestive system is working. You may be taking a walk or studying or sleeping, having forgotten all about the apple, but your stomach and intestines are busy digesting it and absorbing its vitamins and other nutrients. By the time any waste material is excreted, the body has appropriated all it can use from the apple. In short, whether you pay attention or not, the organs of the digestive system perform their specific functions, allowing you to use the food you eat to keep you going. This chapter examines the structure and functions of these organs, and explores the mechanics and chemistry of the digestive processes.
Part 1: Overview of the Digestive System
The function of the digestive system is to break down the foods you eat, release their nutrients, and absorb those nutrients into the body. Although the small intestine is the workhorse of the system, where the majority of digestion occurs, and where most of the released nutrients are absorbed into the blood or lymph, each of the digestive system organs makes a vital contribution to this process (Figure 1).
As is the case with all body systems, the digestive system does not work in isolation; it functions cooperatively with the other systems of the body. Consider for example, the interrelationship between the digestive and cardiovascular systems. Arteries supply the digestive organs with oxygen and processed nutrients, and veins drain the digestive tract.
These intestinal veins, constituting the hepatic portal system, are unique; they do not return blood directly to the heart. Rather, this blood is diverted to the liver where its nutrients are off-loaded for processing before blood completes its circuit back to the heart. At the same time, the digestive system provides nutrients to the heart muscle and vascular tissue to support their functioning. The interrelationship of the digestive and endocrine systems is also critical. Hormones secreted by several endocrine glands, as well as endocrine cells of the pancreas, the stomach, and the small intestine, contribute to the control of digestion and nutrient metabolism. In turn, the digestive system provides the nutrients to fuel endocrine function. Table 1 gives a quick glimpse at how these other systems contribute to the functioning of the digestive system.
Digestive System Organs
The easiest way to understand the digestive system is to divide its organs into two main categories. The first group is the organs that make up the alimentary canal. Accessory digestive organs comprise the second group and are critical for orchestrating the breakdown of food and the assimilation of its nutrients into the body. Accessory digestive organs, despite their name, are critical to the function of the digestive system.
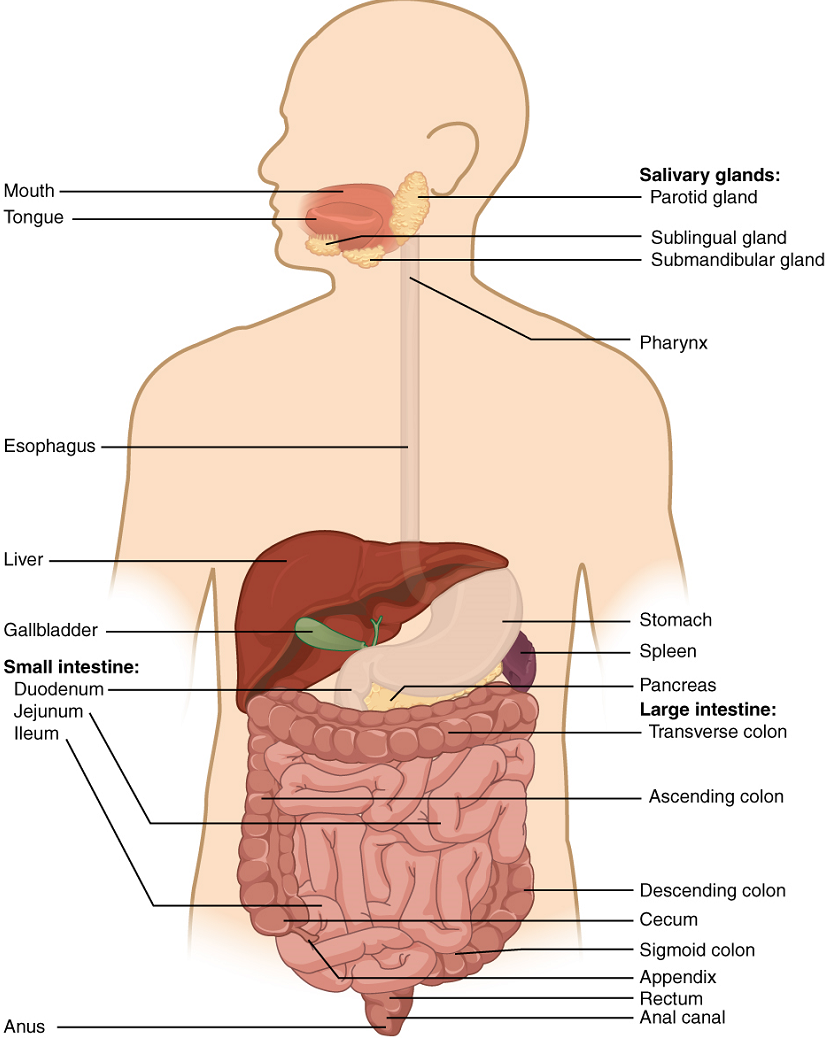
- Alimentary Canal Organs: Also called the gastrointestinal (GI) tract or gut, the alimentary canal (aliment- = “to nourish”) is a one-way tube about 7.62 metres (25 feet) in length during life and closer to 10.67 metres (35 feet) in length when measured after death, once smooth muscle tone is lost. The main function of the organs of the alimentary canal is to nourish the body. This tube begins at the mouth and terminates at the anus. Between those two points, the canal is modified as the pharynx, esophagus, stomach, and small and large intestines to fit the functional needs of the body. Both the mouth and anus are open to the external environment; thus, food and wastes within the alimentary canal are technically considered to be outside the body. Only through the process of absorption do the nutrients in food enter into and nourish the body’s “inner space.”
- Accessory Structures: Each accessory digestive organ aids in the breakdown of food (Figure 2). Within the mouth, the teeth and tongue begin mechanical digestion, whereas the salivary glands begin chemical digestion. Once food products enter the small intestine, the gallbladder, liver, and pancreas release secretions—such as bile and enzymes—essential for digestion to continue. Together, these are called accessory organs because they sprout from the lining cells of the developing gut (mucosa) and augment its function; indeed, you could not live without their vital contributions, and many significant diseases result from their malfunction. Even after development is complete, they maintain a connection to the gut by way of ducts.
Body system | Benefits received by the digestive system |
---|---|
Cardiovascular | Blood supplies digestive organs with oxygen and processed nutrients; capillaries receive absorbed nutrients |
Endocrine | Hormones help regulate secretion in digestive glands and accessory organs |
Integumentary | Skin helps protect digestive organs and synthesizes vitamin D to facilitate calcium absorption |
Lymphatic | Mucosa-associated lymphoid tissue defend against entry of pathogens; lacteals absorb lipids; lymphatic vessels transport lipids to bloodstream |
Muscular | Skeletal muscles support and protect abdominal organs |
Nervous | Sensory and motor neurons help regulate secretions and muscle contractions in the digestive tract |
Respiratory | Respiratory organs provide oxygen and remove carbon dioxide |
Skeletal | Bones help protect and support digestive organs |
Urinary | Kidneys convert vitamin D into its active form, allowing calcium absorption in the small intestine |
Histology of the Alimentary Canal
Throughout its length, the alimentary tract is composed of the same four tissue layers; the details of their structural arrangements vary to fit their specific functions. Starting from the lumen and moving outwards, these layers are the mucosa, submucosa, muscularis, and serosa, which is continuous with the mesentery (Figure 2).
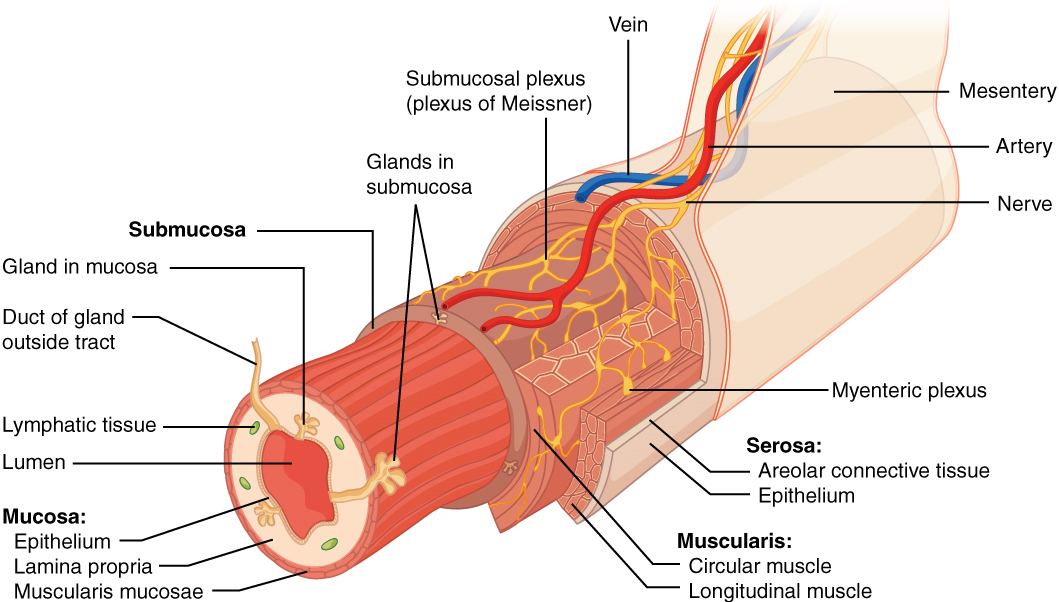
The mucosa is referred to as a mucous membrane, because mucous production is a characteristic feature of gut epithelium. The membrane consists of epithelium, which is in direct contact with ingested food, and the lamina propria, a layer of connective tissue analogous to the dermis. In addition, the mucosa has a thin, smooth muscle layer, called the muscularis mucosa.
- Epithelium—In the mouth, pharynx, esophagus, and anal canal, the epithelium is primarily a non-keratinized, stratified squamous epithelium. In the stomach and intestines, it is a simple columnar epithelium. Notice that the epithelium is in direct contact with the lumen, the space inside the alimentary canal. Interspersed among its epithelial cells are goblet cells, which secrete mucus and fluid into the lumen, and enteroendocrine cells, which secrete hormones into the interstitial spaces between cells. Epithelial cells have a very brief lifespan, averaging from only a couple of days (in the mouth) to about a week (in the gut). This process of rapid renewal helps preserve the health of the alimentary canal, despite the wear and tear resulting from continued contact with foodstuffs.
- Lamina propria—In addition to loose connective tissue, the lamina propria contains numerous blood and lymphatic vessels that transport nutrients absorbed through the alimentary canal to other parts of the body.
- Muscularis mucosa—This thin layer of smooth muscle is in a constant state of tension, pulling the mucosa of the stomach and small intestine into undulating folds. These folds dramatically increase the surface area available for digestion and absorption.
As its name implies, the submucosa lies immediately beneath the mucosa. A broad layer of dense connective tissue, it connects the overlying mucosa to the underlying muscularis. It includes blood and lymphatic vessels (which transport absorbed nutrients), and a scattering of submucosal glands that release digestive secretions. Additionally, it serves as a conduit for a dense branching network of nerves, the submucosal plexus, which functions as described below.
The third layer of the alimentary canal is the muscularis (also called the muscularis externa). The muscularis in the small intestine is made up of a double layer of smooth muscle: an inner circular layer and an outer longitudinal layer. The contractions of these layers promote mechanical digestion, expose more of the food to digestive chemicals, and move the food along the canal. In the most proximal and distal regions of the alimentary canal, including the mouth, pharynx, proximal part of the esophagus, and external anal sphincter, the muscularis is made up of skeletal muscle, which gives you voluntary control over swallowing and defecation. The basic two-layer structure found in the small intestine is modified in the organs proximal and distal to it. The stomach is equipped for its churning function by the addition of a third layer, the oblique muscle. While the colon has two layers like the small intestine, its longitudinal layer is segregated into three narrow parallel bands, the tenia coli, which make it look like a series of pouches rather than a simple tube.
The serosa is the portion of the alimentary canal superficial to the muscularis. Present only in the region of the alimentary canal within the abdominal cavity, it consists of a layer of visceral peritoneum overlying a layer of loose connective tissue. Instead of serosa, the mouth, pharynx, and esophagus have a dense sheath of collagen fibres called the adventitia. These tissues serve to hold the alimentary canal in place near the ventral surface of the vertebral column.
Nerve Supply: As soon as food enters the mouth, it is detected by receptors that send impulses along the sensory neurons of cranial nerves. Without these nerves, not only would your food be without taste, but you would also be unable to feel either the food or the structures of your mouth, and you would be unable to avoid biting yourself as you chew, an action enabled by the motor branches of cranial nerves.
Intrinsic innervation of much of the alimentary canal is provided by the enteric nervous system, which runs from the esophagus to the anus, and contains approximately 100 million motor, sensory, and interneurons (unique to this system compared to all other parts of the peripheral nervous system). (see Figure 2).
Blood Supply: The blood vessels serving the digestive system have two functions. They transport the protein and carbohydrate nutrients absorbed by mucosal cells after food is digested in the lumen. Lipids are absorbed via lacteals, tiny structures of the lymphatic system. The blood vessels’ second function is to supply the organs of the alimentary canal with the nutrients and oxygen needed to drive their cellular processes.
The proximal parts of the alimentary canal are supplied with blood by arteries branching off the aortic arch and thoracic aorta. Below this point, the alimentary canal is supplied with blood by arteries branching from the abdominal aorta. The celiac trunk services the liver, stomach, and duodenum, whereas the superior and inferior mesenteric arteries supply blood to the remaining small and large intestines.
The veins that collect nutrient-rich blood from the small intestine (where most absorption occurs) empty into the hepatic portal system. This venous network takes the blood into the liver where the nutrients are either processed or stored for later use. Only then does the blood drained from the alimentary canal viscera circulate back to the heart. To appreciate just how demanding the digestive process is on the cardiovascular system, consider that while you are “resting and digesting,” about one-fourth of the blood pumped with each heartbeat enters arteries serving the intestines.
The Peritoneum: The digestive organs within the abdominal cavity are held in place by the peritoneum, a broad serous membranous sac made up of squamous epithelial tissue surrounded by connective tissue. It is composed of two different regions: the parietal peritoneum, which lines the abdominal wall, and the visceral peritoneum, which envelopes the abdominal organs (Figure 3). The peritoneal cavity is the space bounded by the visceral and parietal peritoneal surfaces. A few milliliters of watery fluid act as a lubricant to minimize friction between the serosal surfaces of the peritoneum.
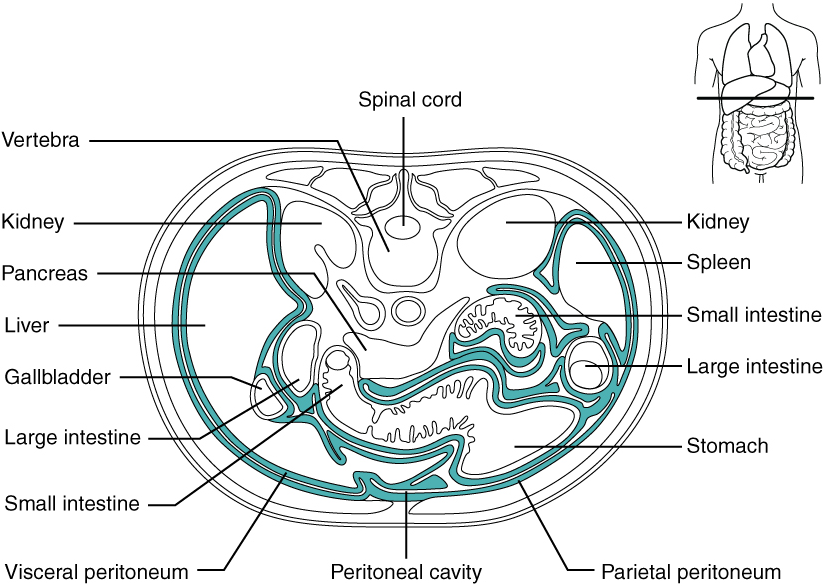
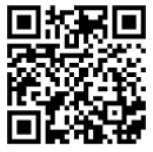
Part 2: Digestive System Processes and Regulation
The digestive system uses mechanical and chemical activities to break food down into absorbable substances during its journey through the digestive system. Table 2 provides an overview of the basic functions of the digestive organs.
Organ | Mechanical functions | Chemical Functions | Absorptive Functions | Other functions |
---|---|---|---|---|
Mouth | Ingests food
Chews and mixes food Moves food into pharynx |
Salivary glands secrete salivary amylase: begins chemical breakdown of carbohydrates
Salivary glands secrete lingual lipase: begins some breakdown of lipids via lingual lipase |
Moistens and dissolves food, allowing taste
Cleans and lubricates teeth and oral cavity Some antimicrobial activity |
|
Pharynx | Propels food from oral cavity to esophagus | Lubricates food and passageways | ||
Esophagus | Propels food to stomach | Lubricates food and passageways | ||
Stomach | Mixes and churns food with gastric juices to form chyme
Releases food into duodenum as chyme Absorbs some fat-soluble substance (e.g., alcohol, aspirin) Secretes antimicrobial substances |
Secrete hydrochloric acid (HCl), needed for enzyme action (e.g. activation of pepsinogen) and immune function (killing many ingested pathogens)
Gastric glands secrete pepsinogen (activated pepsin by stomach acid): begins digestion of proteins Enhances activity of lingual lipase Secretes intrinsic factor required for vitamin B12 absorption in small intestine |
|
|
Small intestine | Mixes chyme with digestive juices
Propels food at a rate slow enough for digestion and absorption Performs physical digestion via segmentation |
Provides optimal medium for enzymatic activity: pancreatic amylase, pancreatic lipase, brush border enzymes | Absorbs breakdown products of carbohydrates, proteins, lipids, nucleic acids
Absorbs vitamins, minerals, water |
|
Accessory organs |
|
Liver: produces bile salts which emulsify lipids, aiding their digestion and absorption
Gallbladder: stores, concentrates, and releases bile Bicarbonate-rich pancreatic juice helps neutralize acidic chyme and provide optimal environment for enzymatic activity |
||
Large intestine | Further breaks down food residues
Propels feces toward rectum Eliminates feces |
Absorbs most residual water, some minerals, vitamins produced by enteric bacteria | Concentrates and temporarily stored food residue prior to defecation
Mucus eases passage of feces through colon |
Digestive Processes
The processes of digestion include six activities: ingestion, propulsion, mechanical or physical digestion, chemical digestion, absorption, and defecation.
The first of these processes, ingestion, refers to the entry of food into the alimentary canal through the mouth. There, the food is chewed and mixed with saliva, which contains enzymes that begin breaking down the carbohydrates in the food plus some lipid digestion via lingual lipase. Chewing increases the surface area of the food and allows an appropriately sized bolus to be produced.
Food leaves the mouth when the tongue and pharyngeal muscles propel it into the esophagus. This act of swallowing, the last voluntary act until defecation, is an example of propulsion, which refers to the movement of food through the digestive tract. It includes both the voluntary process of swallowing and the involuntary process of peristalsis. Peristalsis consists of sequential, alternating waves of contraction and relaxation of the longitudinal and circular smooth muscle layers in the wall of the alimentary canal, which act to propel food along (Figure 4). These waves also play a role in mixing food with digestive juices.
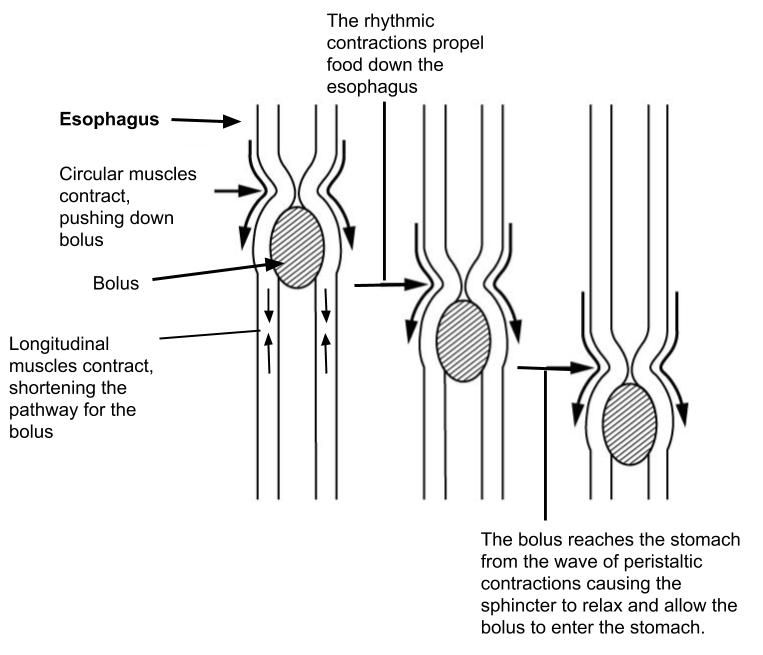
Digestion includes both mechanical and chemical processes. Mechanical digestion is a purely physical process that does not change the chemical nature of the food. Instead, it makes the food smaller to increase both surface area and mobility. It includes mastication, or chewing, as well as tongue movements that help break food into smaller bits and mix food with saliva. Although there may be a tendency to think that mechanical digestion is limited to the first steps of the digestive process, it occurs after the food leaves the mouth, as well. The mechanical churning of food in the stomach serves to further break it apart and expose more of its surface area to digestive juices, creating an acidic “soup” called chyme. Segmentation, which occurs mainly in the small intestine, consists of localized contractions of circular muscle of the muscularis layer of the alimentary canal. These contractions isolate small sections of the intestine, moving their contents back and forth while continuously subdividing, breaking up, and mixing the contents. By moving food back and forth in the intestinal lumen, segmentation mixes food with digestive juices and facilitates absorption.
In chemical digestion, starting in the mouth, digestive secretions break down complex food molecules into their chemical building blocks (for example, proteins into separate amino acids). These secretions vary in composition, but typically contain water, various enzymes, acids, and salts. The process is completed in the small intestine. Since this chemical digestion occurs in the lumen of the gastrointestinal tract as a result of secretions into the lumen, it is a form of extracellular digestion. (Contrast this with the intracellular digestion that occurs after phagocytosis, for example.)
Food that has been broken down is of no value to the body unless it enters the bloodstream and its nutrients are put to work. This occurs through the process of absorption, which takes place primarily within the small intestine. There, most nutrients are absorbed from the lumen of the alimentary canal into the bloodstream through the epithelial cells that make up the mucosa. Lipids are absorbed into lacteals and are transported via the lymphatic vessels to the bloodstream (the subclavian veins near the heart). The details of these processes will be discussed later.
In defecation, the final step in digestion, undigested materials are removed from the body as feces.
In some cases, a single organ is in charge of a digestive process. For example, ingestion occurs only in the mouth and defecation only in the anus. However, most digestive processes involve the interaction of several organs and occur gradually as food moves through the alimentary canal (Figure 5).
Some chemical digestion occurs in the mouth. Some absorption can occur in the mouth and stomach, for example, alcohol and aspirin.
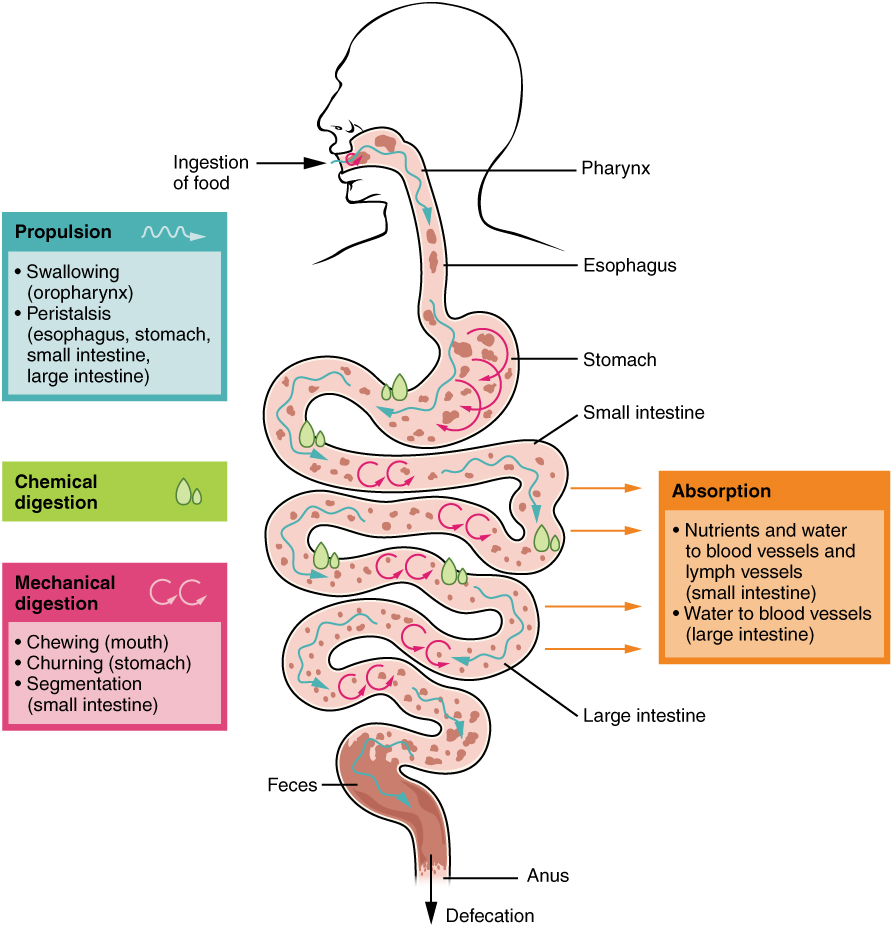
Regulatory Mechanisms
Neural and endocrine regulatory mechanisms work to maintain the optimal conditions in the lumen needed for digestion and absorption. These regulatory mechanisms, which stimulate digestive activity through mechanical and chemical activity, are controlled both extrinsically and intrinsically.
Neural Controls: The walls of the alimentary canal contain a variety of sensors that help regulate digestive functions. These include mechanoreceptors, chemoreceptors, and osmoreceptors, which are capable of detecting mechanical, chemical, and osmotic stimuli, respectively. For example, these receptors can sense when the presence of food has caused the stomach to expand, whether food particles have been sufficiently broken down, how much liquid is present, and the type of nutrients in the food (lipids, carbohydrates, and/or proteins). Stimulation of these receptors provokes an appropriate reflex that furthers the process of digestion. This may entail sending a message that activates the glands that secrete digestive juices into the lumen, or it may mean the stimulation of muscles within the alimentary canal, thereby activating peristalsis and segmentation that move food along the intestinal tract.
Hormonal Controls: A variety of hormones are involved in the digestive process. The main digestive hormone of the stomach is gastrin, which is secreted in response to the presence of food. Gastrin stimulates the secretion of gastric acid by the parietal cells of the stomach mucosa. Other GI hormones are produced and act upon the gut and its accessory organs. Hormones produced by the duodenum include secretin, which stimulates a watery secretion of bicarbonate by the pancreas; cholecystokinin (CCK), which stimulates the secretion of pancreatic enzymes and bile from the liver and release of bile from the gallbladder; and gastric inhibitory peptide, which inhibits gastric secretion and slows gastric emptying and motility.
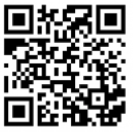
Part 3: The Mouth, Pharynx, and Esophagus
In this section, you will examine the anatomy and functions of the three main organs of the upper alimentary canal—the mouth, pharynx, and esophagus—as well as three associated accessory organs—the tongue, salivary glands, and teeth.
The Mouth
The cheeks, tongue, and palate frame the mouth, which is also called the oral cavity (or buccal cavity). The structures of the mouth are illustrated in Figure 6, and the digestive functions of the mouth are summarized in Table 3.
At the entrance to the mouth are the lips, or labia (singular = labium). Their outer covering is skin, which transitions to a mucous membrane in the mouth proper. Lips are very vascular with a thin layer of keratin; hence, the reason they are “red.” They have a huge representation on the cerebral cortex, which probably explains the human fascination with kissing! The lips cover the orbicularis oris muscle, which regulates what comes in and goes out of the mouth. The labial frenulum is a midline fold of mucous membrane that attaches the inner surface of each lip to the gum. The cheeks make up the oral cavity’s sidewalls. While their outer covering is skin, their inner covering is mucous membrane. This membrane is made up of non-keratinized, stratified squamous epithelium. Beneath the skin and mucous membranes are connective tissue and buccinator muscles. The next time you eat some food, notice how the buccinator muscles in your cheeks and the orbicularis oris muscle in your lips contract, helping you keep the food from falling out of your mouth. Additionally, notice how these muscles work when you are speaking.
The pocket-like part of the mouth that is framed on the inside by the gums and teeth, and on the outside by the cheeks and lips is called the oral vestibule. Moving farther into the mouth, the opening between the oral cavity and throat (oropharynx) is called the fauces (like the kitchen “faucet”). The main open area of the mouth, or oral cavity proper, runs from the gums and teeth to the fauces.
When you are chewing, you do not find it difficult to breathe simultaneously. The next time you have food in your mouth, notice how the arched shape of the roof of your mouth allows you to handle both digestion and respiration at the same time. This arch is called the palate. The anterior region of the palate serves as a wall (or septum) between the oral and nasal cavities as well as a rigid shelf against which the tongue can push food. It is created by the maxillary and palatine bones of the skull and, given its bony structure, is known as the hard palate.
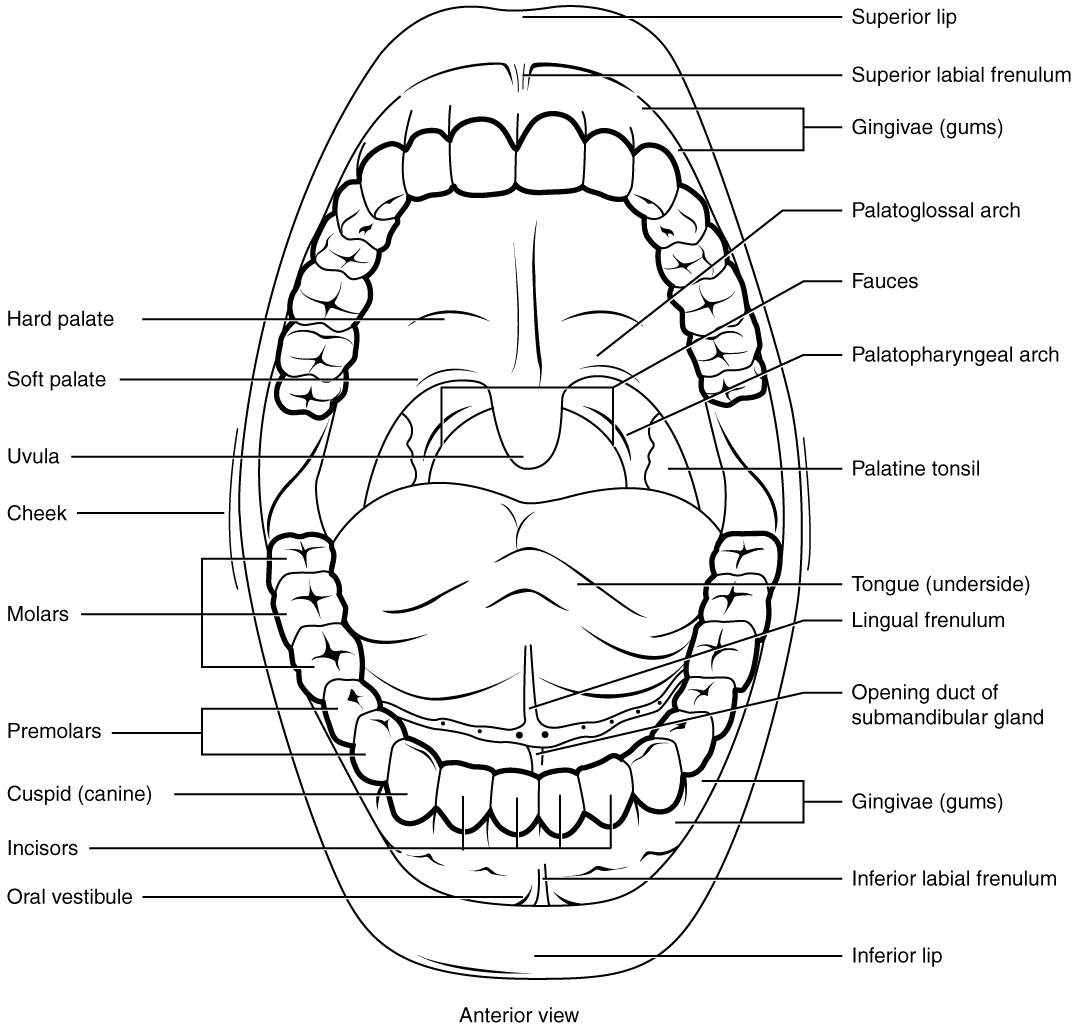
If you run your tongue along the roof of your mouth, you’ll notice that the hard palate ends in the posterior oral cavity, and the tissue becomes fleshier. This part of the palate, known as the soft palate, is composed mainly of skeletal muscle. You can therefore manipulate, subconsciously, the soft palate—for instance, to yawn, swallow, or sing (see Figure 6).
A fleshy bead of tissue called the uvula drops down from the centre of the posterior edge of the soft palate. Although some have suggested that the uvula is a vestigial organ, it serves an important purpose. When you swallow, the soft palate and uvula move upward, helping to keep foods and liquid from entering the nasal cavity. Unfortunately, it can also contribute to the sound produced by snoring. Two muscular folds extend downward from the soft palate, on either side of the uvula
The Tongue: Perhaps you have heard it said that the tongue is the strongest muscle in the body. Those who stake this claim cite its strength proportionate to its size. Although it is difficult to quantify the relative strength of different muscles, it remains indisputable that the tongue is a workhorse, facilitating ingestion, mechanical digestion, chemical digestion (lingual lipase), sensation (of taste, texture, and temperature of food), swallowing, and vocalization.
The tongue is attached to the mandible, the styloid processes of the temporal bones, and the hyoid bone. The hyoid is unique in that it only distantly/indirectly articulates with other bones. The tongue is positioned over the floor of the oral cavity. A medial septum extends the entire length of the tongue, dividing it into symmetrical halves.
Beneath its mucous membrane covering, each half of the tongue is composed of the same number and type of intrinsic and extrinsic skeletal muscles. The intrinsic muscles (those within the tongue) allow you to change the size and shape of your tongue, as well as to stick it out, if you wish. Having such a flexible tongue facilitates both swallowing and speech. The extrinsic muscles of the tongue originate outside the tongue and insert into connective tissues within the tongue. Working in concert, these muscles perform three important digestive functions in the mouth: (1) position food for optimal chewing, (2) gather food into a bolus (rounded mass), and (3) position food so it can be swallowed.
The top and sides of the tongue are studded with papillae, extensions of lamina propria of the mucosa, which are covered in stratified squamous epithelium (Figure 7). Papillae (often incorrectly referred too as taste buds) are bumps on the superior surface of the tongue that contain taste buds (gustatory receptors) and touch receptors. Lingual glands in the lamina propria of the tongue secrete mucus and a watery serous fluid that contains the enzyme lingual lipase, which plays a minor role in breaking down triglycerides but does not begin working until it is activated in the stomach.
The Salivary Glands: Many small salivary glands are housed within the mucous membranes of the mouth and tongue. These minor exocrine glands are constantly secreting saliva, either directly into the oral cavity or indirectly through ducts, even while you sleep. In fact, an average of 1 to 1.5 liters of saliva is secreted each day. Usually just enough saliva is present to moisten the mouth and teeth. Secretion increases when you eat, because saliva is essential to moisten food and initiate the chemical breakdown of carbohydrates. Small amounts of saliva are also secreted by the labial glands in the lips. In addition, the buccal glands in the cheeks, palatal glands in the palate, and lingual glands in the tongue help ensure that all areas of the mouth are supplied with adequate saliva.
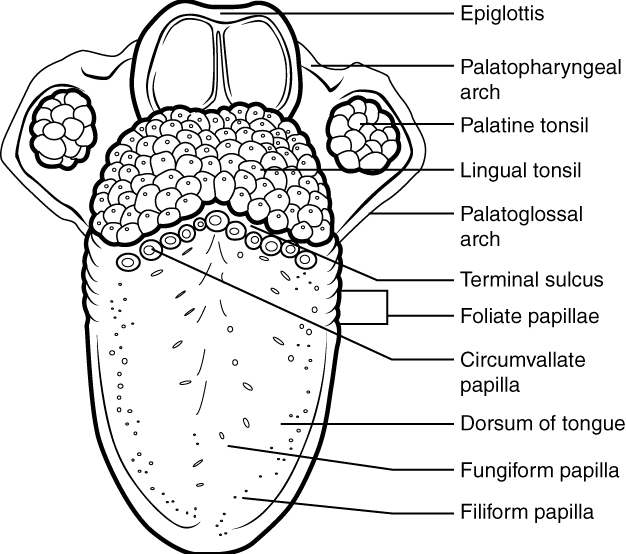
The Major Salivary Glands: Outside the oral mucosa are three pairs of major salivary glands, which secrete the majority of saliva into ducts that open into the mouth:
- The submandibular glands, which are in the floor of the mouth, secrete saliva into the mouth through the submandibular ducts.
- The sublingual glands, which lie below the tongue, use the lesser sublingual ducts to secrete saliva into the oral cavity.
- The parotid glands lie between the skin and the masseter muscle, near the ears. They secrete saliva into the mouth through the parotid duct, which is located near the second upper molar tooth (Figure 8).
Saliva: Saliva is essentially (>95%) water. The remainder is a complex mixture of ions, glycoproteins, enzymes, growth factors, and waste products. Perhaps the most important ingredient in salvia from the perspective of digestion is the enzyme salivary amylase, which initiates the breakdown of starch. Food does not spend enough time in the mouth to allow all the carbohydrates to break down, but salivary amylase continues acting until it is inactivated by stomach acids. Bicarbonate and phosphate ions function as chemical buffers, maintaining saliva at a pH between 6.35 and 6.85.
Salivary mucus helps lubricate food, facilitating movement in the mouth, bolus formation, and swallowing. Saliva contains immunoglobulin A, which prevents microbes from penetrating the epithelium, and lysozyme, which makes saliva antimicrobial.
Each of the major salivary glands secretes a unique formulation of saliva according to its cellular makeup. For example, the parotid glands secrete a watery solution that contains salivary amylase. The submandibular glands have cells similar to those of the parotid glands, as well as mucus-secreting cells. Therefore, saliva secreted by the submandibular glands also contains amylase but in a liquid thickened with mucus. The sublingual glands contain mostly mucous cells, and they secrete the thickest saliva with the least amount of salivary amylase.
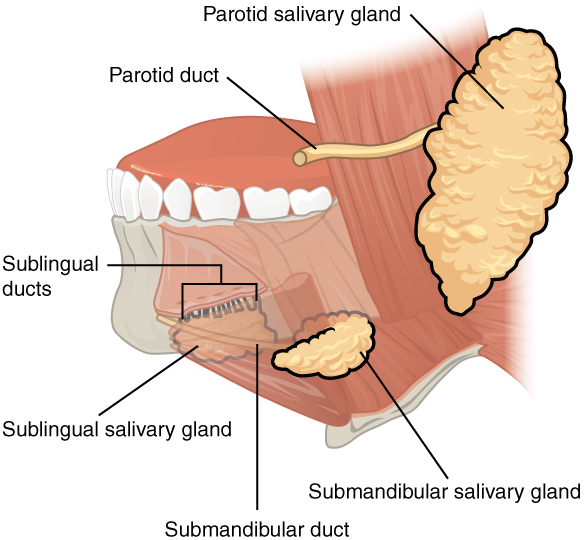
The Teeth: The teeth, or dentes (singular = dens), are organs similar to bones that you use to tear, grind, and otherwise mechanically break down food.
Types of Teeth: During the course of your lifetime, you have two sets of teeth (one set of teeth is a dentition). Your 20 deciduous teeth, or baby teeth, first begin to appear at about 6 months of age. Between approximately age 6 and 12, these teeth are replaced by 32 permanent teeth. Moving from the centre of the mouth toward the side, these are as follows:
- The eight incisors, four top and four bottom, are the sharp front teeth you use for biting into food.
- The four cuspids (or canines) flank the incisors and have a pointed edge (cusp) to tear up food. These fang-like teeth are superb for piercing tough or fleshy foods.
- Posterior to the cuspids are the eight premolars (or bicuspids), which have an overall flatter shape with two rounded cusps useful for mashing foods.
The Pharynx
The pharynx (throat) is involved in both digestion and respiration. It receives food and air from the mouth, and air from the nasal cavities. When food enters the pharynx, involuntary muscle contractions close off the air passageways.
A short tube of skeletal muscle lined with a mucous membrane, the pharynx runs from the posterior oral and nasal cavities to the opening of the esophagus and larynx. It has three subdivisions. The most superior, the nasopharynx, is involved only in breathing and speech. The other two subdivisions, the oropharynx and the laryngopharynx, are used for both breathing and digestion. The oropharynx begins inferior to the nasopharynx and is continuous below with the laryngopharynx (Figure 9). The inferior border of the laryngopharynx connects to the esophagus, whereas the anterior portion connects to the larynx, allowing air to flow into the bronchial tree. During swallowing, the elevator skeletal muscles of the pharynx contract, raising and expanding the pharynx to receive the bolus of food. Once received, these muscles relax and the constrictor muscles of the pharynx contract, forcing the bolus into the esophagus and initiating peristalsis.
Usually during swallowing, the soft palate and uvula rise reflexively to close off the entrance to the nasopharynx. At the same time, the larynx is pulled superiorly and the cartilaginous epiglottis, its most superior structure, folds inferiorly, covering the glottis (the opening to the larynx); this process effectively blocks access to the trachea and bronchi. When the food “goes down the wrong way,” it goes into the trachea. When food enters the trachea, the reaction is to cough, which usually forces the food up and out of the trachea, and back into the pharynx.
The Esophagus
The esophagus is a muscular tube that connects the pharynx to the stomach. It is approximately 25.4 cm (10 in) in length, located posterior to the trachea, and remains in a collapsed form when not engaged in swallowing. The esophagus runs a mainly straight route through the mediastinum of the thorax (Figure 10). To enter the abdomen, the esophagus penetrates the diaphragm through an opening called the esophageal hiatus.
Structure | Action | Outcome |
---|---|---|
Lips and cheeks | Confine food between teeth | Food is chewed evenly during mastication |
Salivary glands | Secrete saliva | Moisten and lubricate lining of the mouth and pharynx
Moisten, soften, dissolve food Clean mouth and teeth Salivary amylase breaks down starch |
Tongue’s extrinsic muscles | Move tongue sideways, and in and out | Manipulate food for chewing
Shape food into a bolus Manipulate food for swallowing |
Tongue’s intrinsic muscles | Change tongue shape | Manipulate food for swallowing |
Taste buds | Sense food in mouth, sense taste | Nerve impulses from taste buds are conducted to salivary nuclei in the brain stem and then to salivary glands, stimulating saliva secretion |
Lingual glands | Secrete lingual lipase | Functions optimally in the stomach, breaks down triglycerides into fatty acids and diglycerides |
Teeth | Shred and crush food | Break down solid food into smaller particles for deglutition |
Passage of Food through the Esophagus: The upper esophageal sphincter, which is continuous with the inferior pharyngeal constrictor, controls the movement of food from the pharynx into the esophagus. The upper two-thirds of the esophagus consists of both smooth and skeletal muscle fibres, with the latter fading out in the bottom third of the esophagus. Rhythmic waves of peristalsis, which begin in the upper esophagus, propel the bolus of food toward the stomach. Meanwhile, secretions from the esophageal mucosa lubricate the esophagus and food. Food passes from the esophagus into the stomach at the lower esophageal sphincter (also called the gastroesophageal or cardiac sphincter). Recall that sphincters are muscles that surround tubes and serve as valves, closing the tube when the sphincters contract and opening it when they relax. The lower esophageal sphincter relaxes to let food pass into the stomach, and then contracts to prevent stomach acids from backing up into the esophagus. Surrounding this sphincter is the muscular diaphragm, which helps close off the sphincter when no food is being swallowed.
Histology of the Esophagus: The mucosa of the esophagus is made up of an epithelial lining that contains non-keratinized, stratified squamous epithelium, with a layer of basal and parabasal cells. This epithelium protects against erosion from food particles. The mucosa’s lamina propria contains mucus-secreting glands. The muscularis layer changes according to location: In the upper third of the esophagus, the muscularis is skeletal muscle. In the middle third, it is both skeletal and smooth muscle. In the lower third, it is smooth muscle. As mentioned previously, the most superficial layer of the esophagus is called the adventitia, not the serosa. In contrast to the stomach and intestines, the loose connective tissue of the adventitia is not covered by a fold of visceral peritoneum. The digestive functions of the esophagus are identified in Table 4.
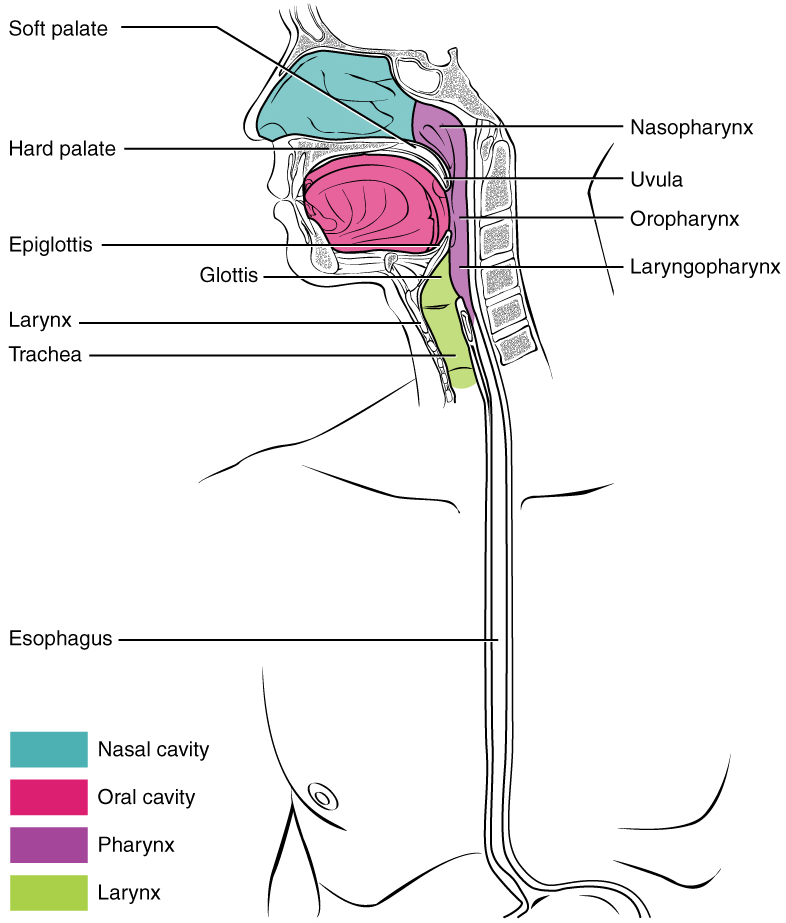
Action | Outcome |
---|---|
Upper esophageal sphincter relaxation | Allows bolus to move from laryngopharynx to esophagus |
Peristalsis | Propels bolus through esophagus |
Lower esophageal sphincter relaxation | Allows bolus to move from esophagus into stomach; prevents chyme from entering esophagus |
Mucus secretion | Lubricates esophagus, allowing easy passage of bolus |
Deglutition: Deglutition is another word for swallowing—the movement of food from the mouth to the stomach. The entire process takes about 4 to 8 seconds for solid or semisolid food, and about 1 second for very soft food and liquids. Although this sounds quick and effortless, deglutition is, in fact, a complex process that involves both the skeletal muscle of the tongue and the muscles of the pharynx and esophagus. It is aided by the presence of mucus and saliva. There are three stages in deglutition: the voluntary phase, the pharyngeal phase, and the esophageal phase (Figure 11). The autonomic nervous system controls the latter two phases.
The Voluntary Phase: The voluntary phase of deglutition (also known as the oral or buccal phase) is so called because you can control when you swallow food. In this phase, chewing has been completed and swallowing is set in motion. The tongue moves upward and backward against the palate, pushing the bolus to the back of the oral cavity and into the oropharynx. Other muscles keep the mouth closed and prevent food from falling out. At this point, the two involuntary phases of swallowing begin.
The Pharyngeal Phase: In the pharyngeal phase, stimulation of receptors in the oropharynx sends impulses to the deglutition centre (a collection of neurons that controls swallowing) in the medulla oblongata. Impulses are then sent back to the uvula and soft palate, causing them to move upward and close off the nasopharynx. The laryngeal muscles also constrict to prevent aspiration of food into the trachea. At this point, deglutition apnea takes place, which means that breathing ceases for a very brief time. Contractions of the pharyngeal constrictor muscles move the bolus through the oropharynx and laryngopharynx. Relaxation of the upper esophageal sphincter then allows food to enter the esophagus.
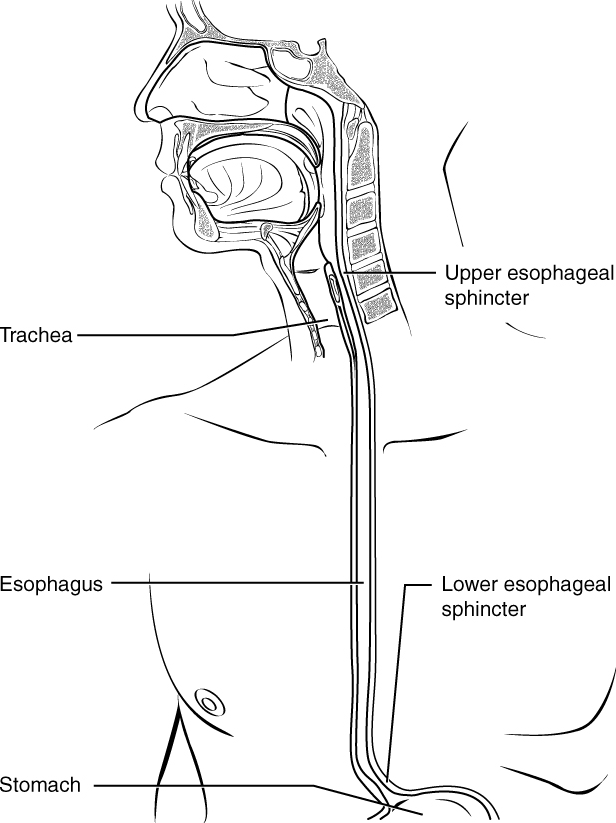
The Esophageal Phase: The entry of food into the esophagus marks the beginning of the esophageal phase of deglutition and the initiation of peristalsis. As in the previous phase, the complex neuromuscular actions are controlled by the medulla oblongata. Peristalsis propels the bolus through the esophagus and toward the stomach. The circular muscle layer of the muscularis contracts, pinching the esophageal wall and forcing the bolus forward. At the same time, the longitudinal muscle layer of the muscularis also contracts, shortening this area and pushing out its walls to receive the bolus. In this way, a series of contractions keeps moving food toward the stomach. When the bolus nears the stomach, distention of the esophagus initiates a short reflex relaxation of the lower esophageal sphincter that allows the bolus to pass into the stomach. During the esophageal phase, esophageal glands secrete mucus that lubricates the bolus and minimizes friction.
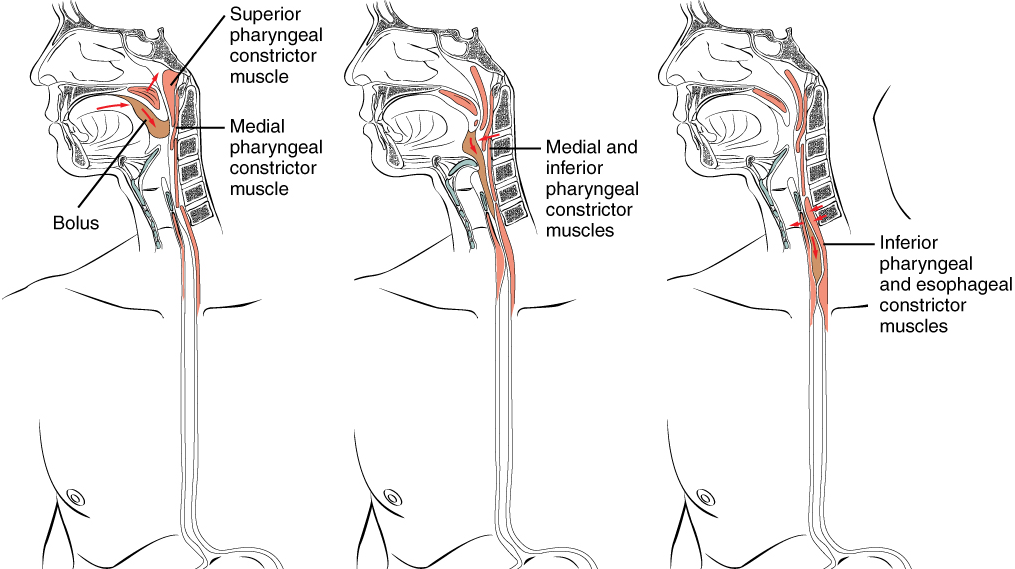
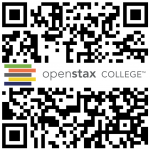
Part 4: The Stomach
Although a minimal amount of carbohydrate digestion occurs in the mouth, chemical digestion really gets underway in the stomach. An expansion of the alimentary canal that lies immediately inferior to the esophagus, the stomach links the esophagus to the first part of the small intestine (the duodenum) and is relatively fixed in place at its esophageal and duodenal ends. In between, however, it can be a highly active structure, contracting and continually changing position and size. These contractions provide mechanical assistance to digestion. The empty stomach is only about the size of your fist, but can stretch to hold as much as 4 litres of food and fluid, or more than 75 times its empty volume, and then return to its resting size when empty. Although you might think that the size of a person’s stomach is related to how much food that individual consumes, body weight does not correlate with stomach size. Rather, when you eat greater quantities of food—such as at holiday dinner—you stretch the stomach more than when you eat less.
An important function of the stomach is to serve as a temporary holding chamber. You can ingest a meal far more quickly than it can be digested and absorbed by the small intestine. Thus, the stomach holds food and parses only small amounts into the small intestine at a time. Foods are not processed in the order they are eaten; rather, they are mixed together with digestive juices in the stomach until they are converted into chyme, which is released into the small intestine.
As you will see in the sections that follow, the stomach plays several important roles in chemical digestion, including the continued digestion of carbohydrates and the initial digestion of proteins and triglycerides. Little if any nutrient absorption occurs in the stomach, with the exception of the negligible amount of nutrients in alcohol.
Structure
There are four main regions in the stomach: the cardia, fundus, body, and pylorus (Figure 12). The cardia (or cardiac region) is the point where the esophagus connects to the stomach and through which food passes into the stomach. Located inferior to the diaphragm, above and to the left of the cardia, is the dome-shaped fundus. Below the fundus is the body, the main part of the stomach. The funnel-shaped pylorus connects the stomach to the duodenum. The wider end of the funnel, the pyloric antrum, connects to the body of the stomach. The narrower end is called the pyloric canal, which connects to the duodenum. The smooth muscle pyloric sphincter is located at this latter point of connection and controls stomach emptying. In the absence of food, the stomach deflates inward, and its mucosa and submucosa fall into a large fold called a ruga.
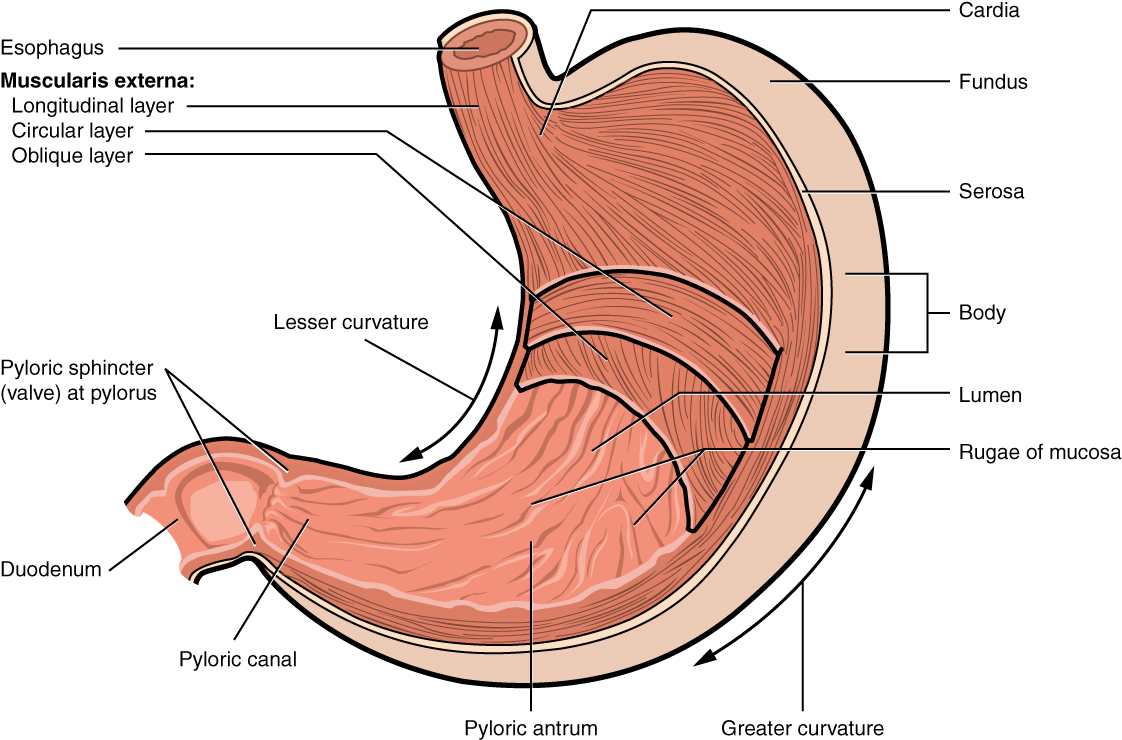
Histology: The wall of the stomach is made of the same four layers as most of the rest of the alimentary canal, but with adaptations to the mucosa and muscularis for the unique functions of this organ. In addition to the typical circular and longitudinal smooth muscle layers, the muscularis has an inner oblique smooth muscle layer (Figure 13). As a result, in addition to moving food through the canal, the stomach can vigorously churn food, mechanically breaking it down into smaller particles.
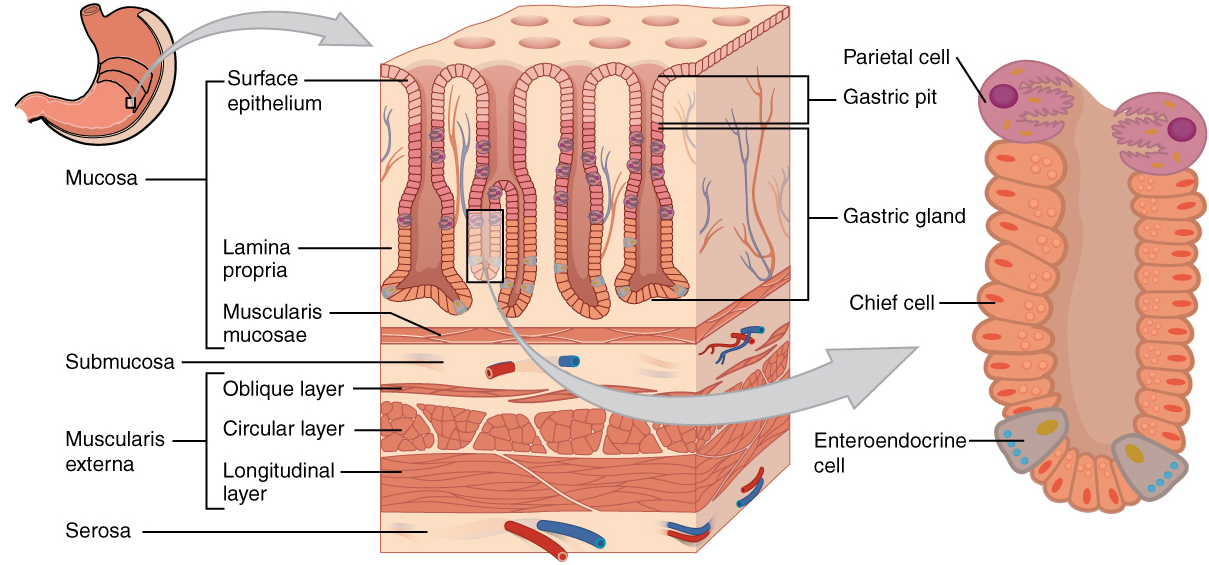
The stomach mucosa’s epithelial lining consists only of surface mucus cells, which secrete a protective coat of alkaline mucus. A vast number of gastric pits dot the surface of the epithelium, giving it the appearance of a well-used pincushion, and mark the entry to each gastric gland, which secretes a complex digestive fluid referred to as gastric juice.
Although the walls of the gastric pits are made up primarily of mucus cells, the gastric glands are made up of different types of cells. The glands of the cardia and pylorus are composed primarily of mucus-secreting cells. Cells that make up the pyloric antrum secrete mucus and a number of hormones, including the majority of the stimulatory hormone, gastrin. The much larger glands of the fundus and body of the stomach, the site of most chemical digestion, produce most of the gastric secretions. These glands are made up of a variety of secretory cells. These include parietal cells, chief cells, mucous neck cells, and enteroendocrine cells.
- Parietal cells—Located primarily in the middle region of the gastric glands are parietal cells, which are among the most highly differentiated of the body’s epithelial cells. These relatively large cells produce both hydrochloric acid (HCl) and intrinsic factor. HCl is responsible for the high acidity (pH 1.5 to 3.5) of the stomach contents and is needed to activate the protein-digesting enzyme, pepsin. The acidity also kills much of the bacteria you ingest with food and helps to denature proteins, making them more available for enzymatic digestion. Intrinsic factor is a glycoprotein necessary for the absorption of vitamin B12 in the small intestine.
- Chief cells—Located primarily in the basal regions of gastric glands are chief cells, which secrete pepsinogen, the inactive proenzyme form of pepsin. HCl is necessary for the conversion of pepsinogen to pepsin.
- Mucous neck cells—Gastric glands in the upper part of the stomach contain mucous neck cells that secrete thin, acidic mucus that is much different from the mucus secreted by the goblet cells of the surface epithelium. The role of this mucus is not currently known.
- Enteroendocrine cells—Finally, enteroendocrine cells found in the gastric glands secrete various hormones into the interstitial fluid of the lamina propria. These include gastrin, which is released mainly by enteroendocrine G cells.
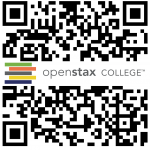
Gastric Secretion: The secretion of gastric juice is controlled by both nerves and hormones. Stimuli in the brain, stomach, and small intestine activate or inhibit gastric juice production. This is why the three phases of gastric secretion are called the cephalic, gastric, and intestinal phases (Figure 14). However, once gastric secretion begins, all three phases can occur simultaneously.
The cephalic phase (reflex phase) of gastric secretion, which is relatively brief, takes place before food enters the stomach. The smell, taste, sight, or thought of food triggers this phase. For example, when you bring a piece of sushi to your lips, impulses from receptors in your taste buds or the nose are relayed to your brain, which returns signals that increase gastric secretion to prepare your stomach for digestion. This enhanced secretion is a conditioned reflex, meaning it occurs only if you like or want a particular food. Depression and loss of appetite can suppress the cephalic reflex.
The gastric phase of secretion lasts 3 to 4 hours, and is set in motion by local neural and hormonal mechanisms triggered by the entry of food into the stomach. For example, when your sushi reaches the stomach, it creates distention that activates the stretch receptors. This stimulates parasympathetic neurons to release acetylcholine, which then provokes increased secretion of gastric juice. Partially digested proteins, caffeine, and rising pH stimulate the release of gastrin from enteroendocrine G cells, which in turn induces parietal cells to increase their production of HCl, which is needed to create an acidic environment for the conversion of pepsinogen to pepsin, and protein digestion. Additionally, the release of gastrin activates vigorous smooth muscle contractions. However, it should be noted that the stomach does have a natural means of avoiding excessive acid secretion and potential heartburn. Whenever pH levels drop too low, cells in the stomach react by suspending HCl secretion and increasing mucous secretions.
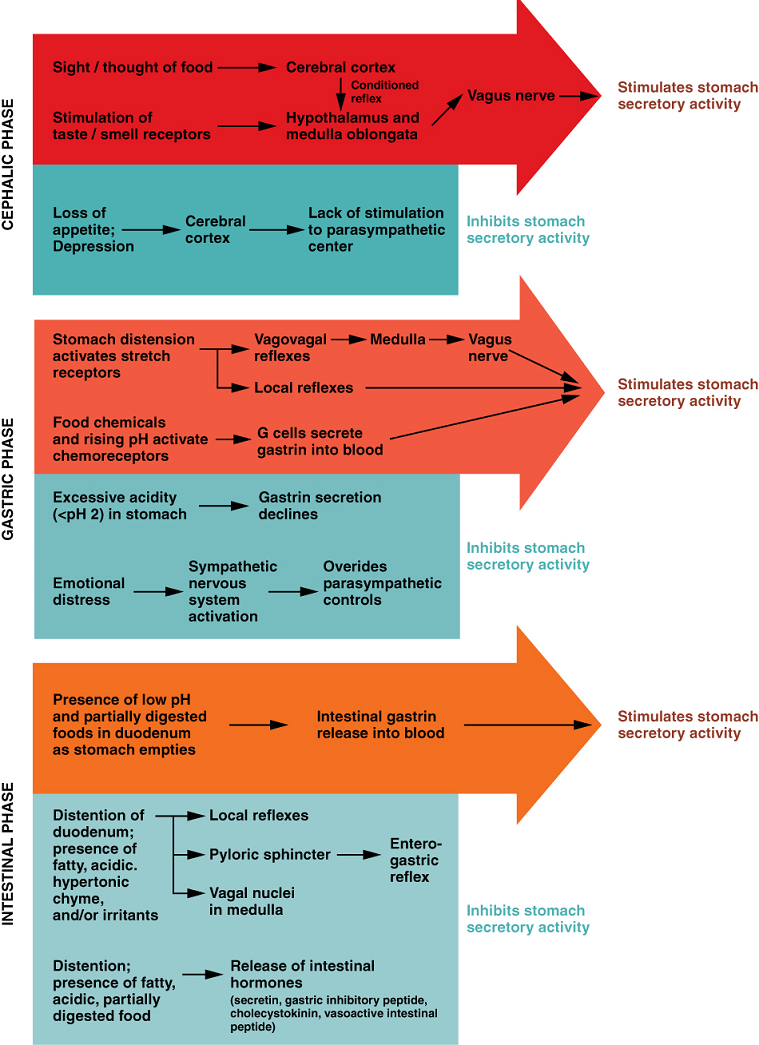
The intestinal phase of gastric secretion has both excitatory and inhibitory elements. The duodenum has a major role in regulating the stomach and its emptying. When partially digested food fills the duodenum, intestinal mucosal cells release a hormone called intestinal (enteric) gastrin, which further excites gastric juice secretion. This stimulatory activity is brief, however, because when the intestine distends with chyme, the enterogastric reflex inhibits secretion. One of the effects of this reflex is to close the pyloric sphincter, which blocks additional chyme from entering the duodenum.
The Mucosal Barrier: The mucosa of the stomach is exposed to the highly corrosive acidity of gastric juice. Gastric enzymes that can digest protein can also digest the stomach itself. The stomach is protected from self-digestion by the mucosal barrier. This barrier has several components. First, the stomach wall is covered by a thick coating of bicarbonate-rich mucus. This mucus forms a physical barrier, and its bicarbonate ions neutralize acid. Second, the epithelial cells of the stomach’s mucosa meet at tight junctions, which block gastric juice from penetrating the underlying tissue layers. Finally, stem cells located where gastric glands join the gastric pits quickly replace damaged epithelial mucosal cells, when the epithelial cells are shed. In fact, the surface epithelium of the stomach is completely replaced every 3 to 6 days.
Digestive Functions of the Stomach
The stomach participates in virtually all the digestive activities with the exception of ingestion and defecation. Although almost all absorption takes place in the small intestine, the stomach does absorb some nonpolar substances, such as alcohol and aspirin.
Mechanical Digestion: Within a few moments after food after enters your stomach, mixing waves begin to occur at intervals of approximately 20 seconds. A mixing wave is a unique type of peristalsis that mixes and softens the food with gastric juices to create chyme. The initial mixing waves are relatively gentle, but these are followed by more intense waves, starting at the body of the stomach and increasing in force as they reach the pylorus. It is fair to say that long before your sushi exits through the pyloric sphincter, it bears little resemblance to the sushi you ate.
The pylorus, which holds around 30 mL (1 fluid ounce) of chyme, acts as a filter, permitting only liquids and small food particles to pass through the mostly, but not fully, closed pyloric sphincter. In a process called gastric emptying, rhythmic mixing waves force about 3 mL of chyme at a time through the pyloric sphincter and into the duodenum. Release of a greater amount of chyme at one time would overwhelm the capacity of the small intestine to handle it. The rest of the chyme is pushed back into the body of the stomach, where it continues mixing. This process is repeated when the next mixing waves force more chyme into the duodenum.
Gastric emptying is regulated by both the stomach and the duodenum. The presence of chyme in the duodenum activates receptors that inhibit gastric secretion. This prevents additional chyme from being released by the stomach before the duodenum is ready to process it.
Chemical Digestion: The fundus plays an important role, because it stores both undigested food and gases that are released during the process of chemical digestion. Food may sit in the fundus of the stomach for a while before being mixed with the chyme. While the food is in the fundus, the digestive activities of salivary amylase continue until the food begins mixing with the acidic chyme. Ultimately, mixing waves incorporate this food with the chyme, the acidity of which inactivates salivary amylase. The acidity of the chyme also allows lingual lipase to break down triglycerides into free fatty acids and diglycerides more efficiently than it could in the less acidic environment of the mouth.
The breakdown of protein begins in the stomach through the actions of HCl and the enzyme pepsin. During infancy, gastric glands also produce rennin, an enzyme that helps digest milk protein.
Its numerous digestive functions notwithstanding, there is only one stomach function necessary to life: the production of intrinsic factor. The intestinal absorption of vitamin B12, which is necessary for both the production of mature red blood cells and normal neurological functioning, cannot occur without intrinsic factor. People who undergo total gastrectomy (stomach removal)—for life-threatening stomach cancer, for example—can survive with minimal digestive dysfunction if they receive vitamin B12 injections.
The contents of the stomach are completely emptied into the duodenum within 2 to 4 hours after you eat a meal. Different types of food take different amounts of time to process. Foods heavy in carbohydrates empty fastest, followed by high-protein foods. Meals with a high triglyceride content remain in the stomach the longest. Since enzymes in the small intestine digest fats slowly, food can stay in the stomach for 6 hours or longer when the duodenum is processing fatty chyme. However, note that this is still a fraction of the 24 to 72 hours that full digestion typically takes from start to finish.
Part 5: The Small and Large Intestines
The word intestine is derived from a Latin root meaning “internal,” and indeed, the two organs together nearly fill the interior of the abdominal cavity. In addition, called the small and large bowel, or colloquially the “guts,” they constitute the greatest mass and length of the alimentary canal and, with the exception of ingestion, perform all digestive system functions.
The Small Intestine
Chyme released from the stomach enters the small intestine, which is the primary digestive organ in the body. Not only is this where most digestion occurs, it is also where practically all absorption occurs. The longest part of the alimentary canal, the small intestine is about 3.05 metres (10 feet) long in a living person (but about twice as long in a cadaver due to the loss of muscle tone). Since this makes it about five times longer than the large intestine, you might wonder why it is called “small.” In fact, its name derives from its relatively smaller diameter of only about 2.54 cm (1 in), compared with 7.62 cm (3 in) for the large intestine. As we’ll see shortly, in addition to its length, the folds and projections of the lining of the small intestine work to give it an enormous surface area, which is approximately 200 m2, more than 100 times the surface area of your skin. This large surface area is necessary for complex processes of digestion and absorption that occur within it.
Structure: The coiled tube of the small intestine is subdivided into three regions. From proximal (at the stomach) to distal, these are the duodenum, jejunum, and ileum (Figure 15).
The shortest region is the 25.4-cm (10-in) duodenum, which begins at the pyloric sphincter. Just past the pyloric sphincter, it bends posteriorly behind the peritoneum, becoming retroperitoneal, and then makes a C-shaped curve around the head of the pancreas before ascending anteriorly again to return to the peritoneal cavity and join the jejunum. The duodenum can therefore be subdivided into four segments: the superior, descending, horizontal, and ascending duodenum.
Of particular interest is the hepatopancreatic ampulla (ampulla of Vater). Located in the duodenal wall, the ampulla marks the transition from the anterior portion of the alimentary canal to the mid-region, and is where the bile duct (through which bile passes from the liver) and the main pancreatic duct (through which pancreatic juice passes from the pancreas) join. This ampulla opens into the duodenum at a tiny volcano-shaped structure called the major duodenal papilla. The hepatopancreatic sphincter (sphincter of Oddi) regulates the flow of both bile and pancreatic juice from the ampulla into the duodenum.
The jejunum is about 0.9 metres (3 feet) long (in life) and runs from the duodenum to the ileum. Jejunum means “empty” in Latin and supposedly was so named by the ancient Greeks who noticed it was always empty at death.
No clear demarcation exists between the jejunum and the final segment of the small intestine, the ileum.
The ileum is the longest part of the small intestine, measuring about 1.8 metres (6 feet) in length. It is thicker, more vascular, and has more developed mucosal folds than the jejunum. The ileum joins the cecum, the first portion of the large intestine, at the ileocecal sphincter (or valve). The jejunum and ileum are tethered to the posterior abdominal wall by the mesentery. The large intestine frames these three parts of the small intestine.
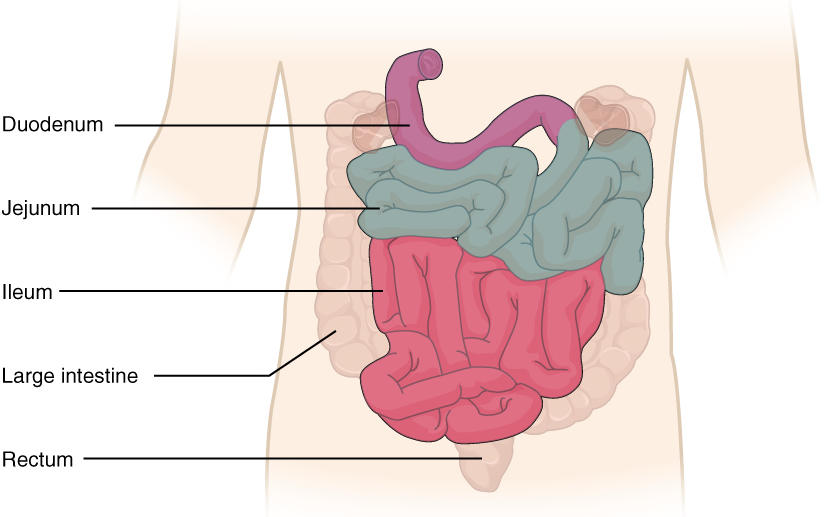
Parasympathetic nerve fibres from the vagus nerve and sympathetic nerve fibres from the thoracic splanchnic nerve provide extrinsic innervation to the small intestine. The superior mesenteric artery is its main arterial supply. Veins run parallel to the arteries and drain into the superior mesenteric vein. Nutrient-rich blood from the small intestine is then carried to the liver via the hepatic portal vein.
Histology: The wall of the small intestine is composed of the same four layers typically present in the alimentary system. However, three features of the mucosa and submucosa are unique. These features, which increase the absorptive surface area of the small intestine more than 600-fold, include circular folds, villi, and microvilli (Figure 16). These adaptations are most abundant in the proximal two-thirds of the small intestine, where the majority of absorption occurs.
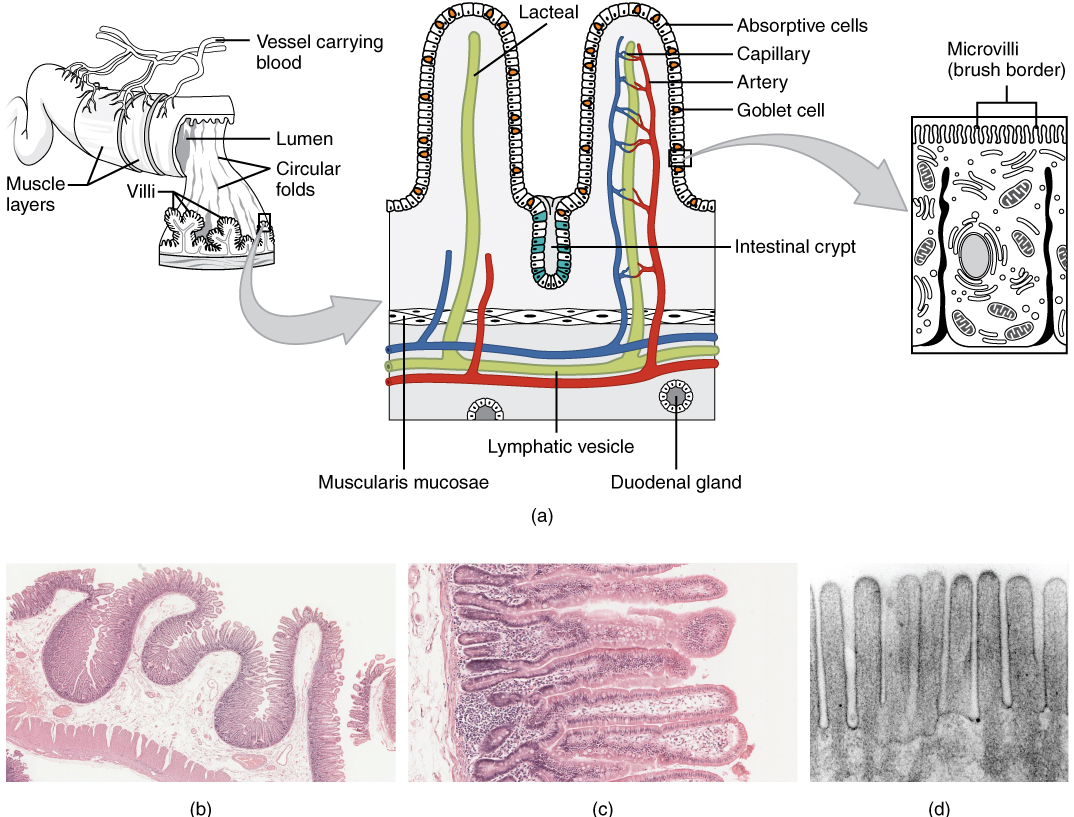
Circular folds: Also called a plica circulare, a circular fold is a deep ridge in the mucosa and submucosa. Beginning near the proximal part of the duodenum and ending near the middle of the ileum, these folds facilitate absorption. Their shape causes the chyme to spiral, rather than move in a straight line, through the small intestine. Spiraling slows the movement of chyme and provides the time needed for nutrients to be fully absorbed.
Villi: Within the circular folds are small (0.5–1 mm long) hair-like vascularized projections called villi (singular = villus) that give the mucosa a furry texture. There are about 20 to 40 villi per square millimetre, increasing the surface area of the epithelium tremendously. The mucosal epithelium, primarily composed of absorptive cells, covers the villi. In addition to muscle and connective tissue to support its structure, each villus contains a capillary bed composed of one arteriole and one venule, as well as a lymphatic capillary called a lacteal. The breakdown products of carbohydrates and proteins (sugars and amino acids) can enter the bloodstream directly, but lipid breakdown products are absorbed by the lacteals and transported to the bloodstream via the lymphatic system.
Microvilli: As their name suggests, microvilli (singular = microvillus) are much smaller (1 µm) than villi. They are cylindrical apical surface extensions of the plasma membrane of the mucosa’s epithelial cells, and are supported by microfilaments within those cells. Although their small size makes it difficult to see each microvillus, their combined microscopic appearance suggests a mass of bristles, which is termed the brush border. Fixed to the surface of the microvilli membranes are enzymes that finish digesting carbohydrates and proteins. There are an estimated 200 million microvilli per square millimetre of small intestine, greatly expanding the surface area of the plasma membrane and thus greatly enhancing absorption.
Intestinal Glands: In addition to the three specialized absorptive features just discussed, the mucosa between the villi is dotted with deep crevices that each lead into a tubular intestinal gland (crypt of Lieberkühn), which is formed by cells that line the crevices (see Figure 16). These produce intestinal juice, a slightly alkaline (pH 7.4 to 7.8) mixture of water and mucus. Each day, about 0.95 to 1.9 liters (1 to 2 quarts) are secreted in response to the distention of the small intestine or the irritating effects of chyme on the intestinal mucosa.
The submucosa of the duodenum is the only site of the complex mucus-secreting duodenal glands (Brunner’s glands), which produce a bicarbonate-rich alkaline mucus that buffers the acidic chyme as it enters from the stomach.
Mechanical Digestion in the Small Intestine: The movement of intestinal smooth muscles includes both segmentation and a form of peristalsis called migrating motility complexes. The kind of peristaltic mixing waves seen in the stomach are not observed here.
If you could see into the small intestine when it was going through segmentation, it would look as if the contents were being shoved incrementally back and forth, as the rings of smooth muscle repeatedly contract and then relax. Segmentation in the small intestine does not force chyme through the tract. Instead, it combines the chyme with digestive juices and pushes food particles against the mucosa to be absorbed. The duodenum is where the most rapid segmentation occurs, at a rate of about 12 times per minute. In the ileum, segmentations are only about eight times per minute (Figure 17).
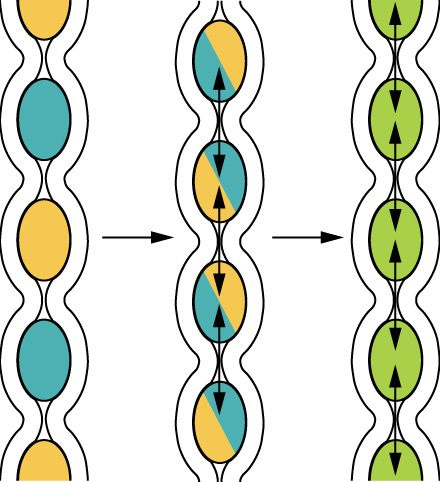
When most of the chyme has been absorbed, the small intestinal wall becomes less distended. At this point, the localized segmentation process is replaced by transport movements. The duodenal mucosa secretes the hormone motilin, which initiates peristalsis. These complexes, which begin in the duodenum, force chyme through a short section of the small intestine and then stop. The next contraction begins a little bit farther down than the first, forces chyme a bit farther through the small intestine, then stops. These complexes move slowly down the small intestine, forcing chyme on the way, taking around 90 to 120 minutes to finally reach the end of the ileum. At this point, the process is repeated, starting in the duodenum.
The ileocecal valve, a sphincter, is usually in a constricted state, but when motility in the ileum increases, this sphincter relaxes, allowing food residue to enter the first portion of the large intestine, the cecum. Relaxation of the ileocecal sphincter is controlled by both nerves and hormones. First, digestive activity in the stomach provokes the gastroileal reflex, which increases the force of ileal segmentation. Second, the stomach releases the hormone gastrin, which enhances ileal motility, thus relaxing the ileocecal sphincter. After chyme passes through, backward pressure helps close the sphincter, preventing backflow into the ileum. Because of this reflex, your lunch is completely emptied from your stomach and small intestine by the time you eat your dinner. It takes about 3 to 5 hours for all chyme to leave the small intestine.
Chemical Digestion in the Small Intestine: The digestion of proteins and carbohydrates, which partially occurs in the stomach, is completed in the small intestine with the aid of intestinal and pancreatic juices. Lipids arrive in the intestine largely undigested, so much of the focus here is on lipid digestion, which is facilitated by bile and the enzyme pancreatic lipase.
Moreover, intestinal juice combines with pancreatic juice to provide a liquid medium that facilitates absorption. The intestine is also where most water is absorbed, via osmosis. The small intestine’s absorptive cells also synthesize digestive enzymes and then place them in the plasma membranes of the microvilli. This distinguishes the small intestine from the stomach; that is, enzymatic digestion occurs not only in the lumen, but also on the luminal surfaces of the mucosal cells.
For optimal chemical digestion, chyme must be delivered from the stomach slowly and in small amounts. This is because chyme from the stomach is typically hypertonic, and if large quantities were forced all at once into the small intestine, the resulting osmotic water loss from the blood into the intestinal lumen would result in potentially life-threatening low blood volume. In addition, continued digestion requires an upward adjustment of the low pH of stomach chyme, along with rigorous mixing of the chyme with bile and pancreatic juices. Both processes take time, so the pumping action of the pylorus must be carefully controlled to prevent the duodenum from being overwhelmed with chyme.
The Large Intestine
The large intestine is the terminal part of the alimentary canal. The primary function of this organ is to finish absorption of nutrients and water, synthesize certain vitamins, form feces, and eliminate feces from the body.
Structure: The large intestine runs from the appendix to the anus. It frames the small intestine on three sides. Despite its being about one-half as long as the small intestine, it is called large because it is more than twice the diameter of the small intestine, about 3 inches.
Subdivisions: The large intestine is subdivided into four main regions: the cecum, the colon, the rectum, and the anus. The ileocecal valve, located at the opening between the ileum and the large intestine, controls the flow of chyme from the small intestine to the large intestine.
1. Cecum: The first part of the large intestine is the cecum, a sac-like structure that is suspended inferior to the ileocecal valve. It is about 6 cm (2.4 in) long, receives the contents of the ileum, and continues the absorption of water and salts. The appendix (or vermiform appendix) is a winding tube that attaches to the cecum. Although the 7.6-cm (3-in) long appendix contains lymphoid tissue, suggesting an immunologic function, this organ is generally considered vestigial. However, at least one recent report postulates a survival advantage conferred by the appendix: In diarrheal illness, the appendix may serve as a bacterial reservoir to repopulate the enteric bacteria for those surviving the initial phases of the illness. Moreover, its twisted anatomy provides a haven for the accumulation and multiplication of enteric bacteria. The mesoappendix, the mesentery of the appendix, tethers it to the mesentery of the ileum.
2. Colon: The cecum blends seamlessly with the colon. Upon entering the colon, the food residue first travels up the ascending colon on the right side of the abdomen. At the inferior surface of the liver, the colon bends to the right colic flexure (hepatic flexure) and becomes the transverse colon. The region defined as hindgut begins with the last third of the transverse colon and continues on. Food residue passing through the transverse colon travels across to the left side of the abdomen, where the colon angles sharply immediately inferior to the spleen, at the left colic flexure (splenic flexure). From there, food residue passes through the descending colon, which runs down the left side of the posterior abdominal wall. After entering the pelvis inferiorly, it becomes the s-shaped sigmoid colon, which extends medially to the midline (Figure 18).
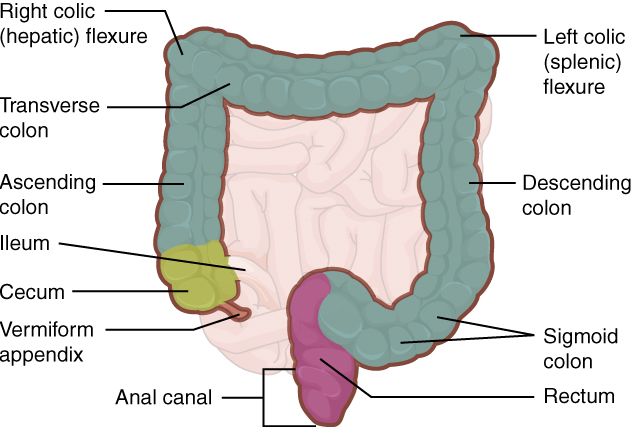
3. Rectum: Food residue leaving the sigmoid colon enters the rectum in the pelvis, near the third sacral vertebra. The final 20.3 cm (8 in) of the alimentary canal, the rectum extends anterior to the sacrum and coccyx. Even though rectum is Latin for “straight,” this structure follows the curved contour of the sacrum and has three lateral bends that create a trio of internal transverse folds called the rectal valves. These valves help separate the feces from gas to prevent the simultaneous passage of feces and gas.
4. Anal Canal: Finally, food residue reaches the last part of the large intestine, the anal canal, which is located in the perineum, completely outside of the abdominopelvic cavity. This 3.8–5 cm (1.5–2 in) long structure opens to the exterior of the body at the anus. The anal canal includes two sphincters. The internal anal sphincter is made of smooth muscle, and its contractions are involuntary. The external anal sphincter is made of skeletal muscle, which is under voluntary control. Except when defecating, both usually remain closed.
Histology: There are several notable differences between the walls of the large and small intestines (Figure 19). For example, few enzyme-secreting cells are found in the wall of the large intestine, and there are no circular folds or villi. Other than in the anal canal, the mucosa of the colon is simple columnar epithelium made mostly of enterocytes (absorptive cells) and goblet cells. In addition, the wall of the large intestine has far more intestinal glands, which contain a vast population of enterocytes and goblet cells. These goblet cells secrete mucus that eases the movement of feces and protects the intestine from the effects of the acids and gases produced by enteric bacteria. The enterocytes absorb water and salts as well as vitamins produced by your intestinal bacteria.
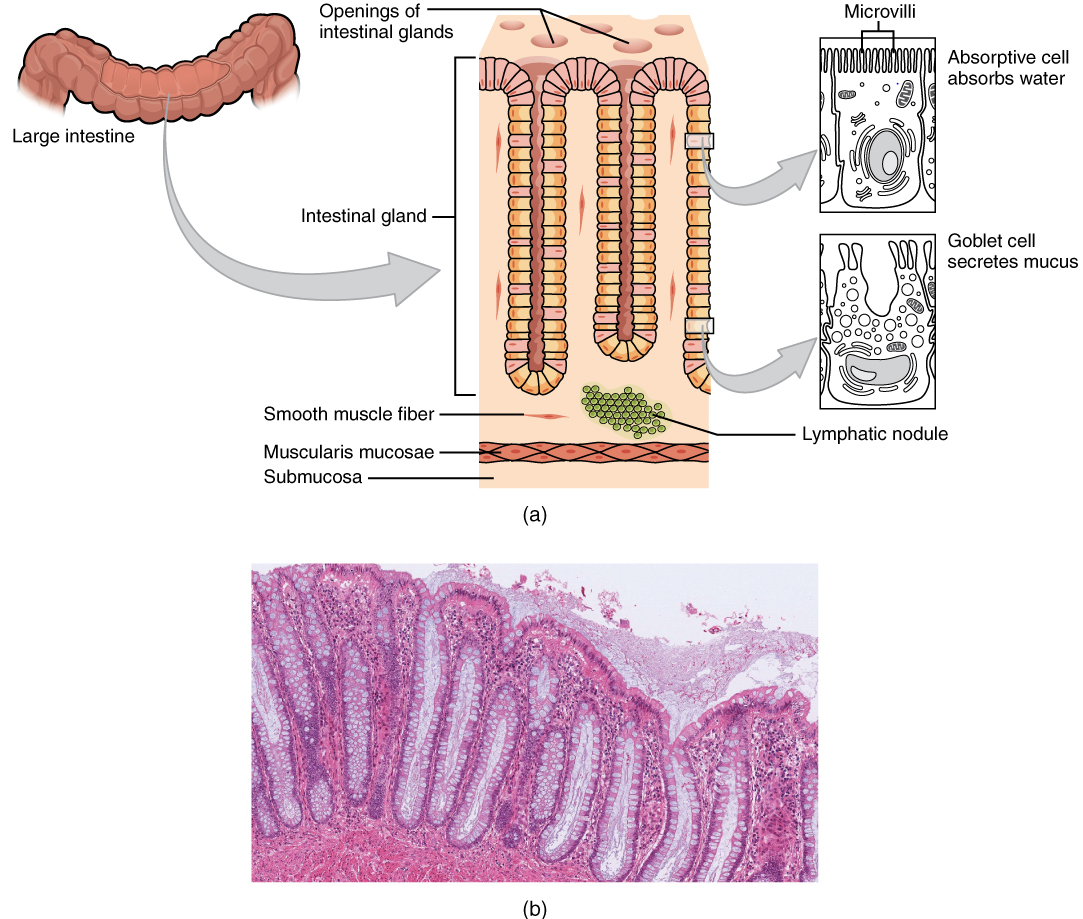
Digestive Functions of the Large Intestine: The residue of chyme that enters the large intestine contains few nutrients except water, which is reabsorbed as the residue lingers in the large intestine, typically for 12 to 24 hours. Thus, it may not surprise you that the large intestine can be completely removed without significantly affecting digestive functioning. For example, in severe cases of inflammatory bowel disease, the large intestine can be removed by a procedure known as a colectomy. Often, a new fecal pouch can be crafted from the small intestine and sutured to the anus, but if not, an ileostomy can be created by bringing the distal ileum through the abdominal wall, allowing the watery chyme to be collected in a bag-like adhesive appliance.
Mechanical Digestion: In the large intestine, mechanical digestion begins when chyme moves from the ileum into the cecum, an activity regulated by the ileocecal sphincter. Right after you eat, peristalsis in the ileum forces chyme into the cecum. When the cecum is distended with chyme, contractions of the ileocecal sphincter strengthen. Once chyme enters the cecum, colon movements begin.
Mechanical digestion in the large intestine includes a combination of three types of movements. The presence of food residues in the colon stimulates a slow-moving haustral contraction. This type of movement involves sluggish segmentation, primarily in the transverse and descending colons. When a haustrum is distended with chyme, its muscle contracts, pushing the residue into the next haustrum. These contractions occur about every 30 minutes, and each last about 1 minute. These movements also mix the food residue, which helps the large intestine absorb water. The second type of movement is peristalsis, which, in the large intestine, is slower than in the more proximal portions of the alimentary canal. The third type is a mass movement. These strong waves start midway through the transverse colon and quickly force the contents toward the rectum. Mass movements usually occur three or four times per day, either while you eat or immediately afterward. Distension in the stomach and the breakdown products of digestion in the small intestine provoke the gastrocolic reflex, which increases motility, including mass movements, in the colon. Fibre in the diet both softens the stool and increases the power of colonic contractions, optimizing the activities of the colon.
Chemical Digestion: Although the glands of the large intestine secrete mucus, they do not secrete digestive enzymes. Therefore, chemical digestion in the large intestine occurs exclusively because of bacteria in the lumen of the colon. Through the process of saccharolytic fermentation, bacteria break down some of the remaining carbohydrates. This results in the discharge of hydrogen, carbon dioxide, and methane gases that create flatus (gas) in the colon; flatulence is excessive flatus. Each day, up to 1500 mL of flatus is produced in the colon. More is produced when you eat foods such as beans, which are rich in otherwise indigestible sugars and complex carbohydrates like soluble dietary fibre.
Absorption, Feces Formation, and Defecation
The small intestine absorbs about 90 percent of the water you ingest (either as liquid or within solid food). The large intestine absorbs most of the remaining water, a process that converts the liquid chyme residue into semisolid feces (“stool”). Feces is composed of undigested food residues, unabsorbed digested substances, millions of bacteria, old epithelial cells from the GI mucosa, inorganic salts, and enough water to let it pass smoothly out of the body. Of every 500 mL (17 ounces) of food residue that enters the cecum each day, about 150 mL (5 ounces) become feces.
Feces are eliminated through contractions of the rectal muscles. You help this process by a voluntary procedure called Valsalva’s maneuver, in which you increase intra-abdominal pressure by contracting your diaphragm and abdominal wall muscles, and closing your glottis.
The process of defecation begins when mass movements force feces from the colon into the rectum, stretching the rectal wall and provoking the defecation reflex, which eliminates feces from the rectum. This parasympathetic reflex is mediated by the spinal cord. It contracts the sigmoid colon and rectum, relaxes the internal anal sphincter, and initially contracts the external anal sphincter. The presence of feces in the anal canal sends a signal to the brain, which gives you the choice of voluntarily opening the external anal sphincter (defecating) or keeping it temporarily closed. If you decide to delay defecation, it takes a few seconds for the reflex contractions to stop and the rectal walls to relax. The next mass movement will trigger additional defecation reflexes until you defecate.
If defecation is delayed for an extended time, additional water is absorbed, making the feces firmer and potentially leading to constipation. On the other hand, if the waste matter moves too quickly through the intestines, not enough water is absorbed, and diarrhea can result. This can be caused by the ingestion of foodborne pathogens. In general, diet, health, and stress determine the frequency of bowel movements. The number of bowel movements varies greatly between individuals, ranging from two or three per day to three or four per week.
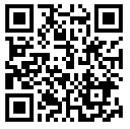
Part 6: Accessory Organs in Digestion: The Liver, Pancreas, and Gallbladder
Chemical digestion in the small intestine relies on the activities of three accessory digestive organs: the liver, pancreas, and gallbladder (Figure 20). The digestive role of the liver is to produce bile and export it to the duodenum. The gallbladder primarily stores, concentrates, and releases bile. The pancreas produces pancreatic juice, which contains digestive enzymes and bicarbonate ions, and delivers it to the duodenum.
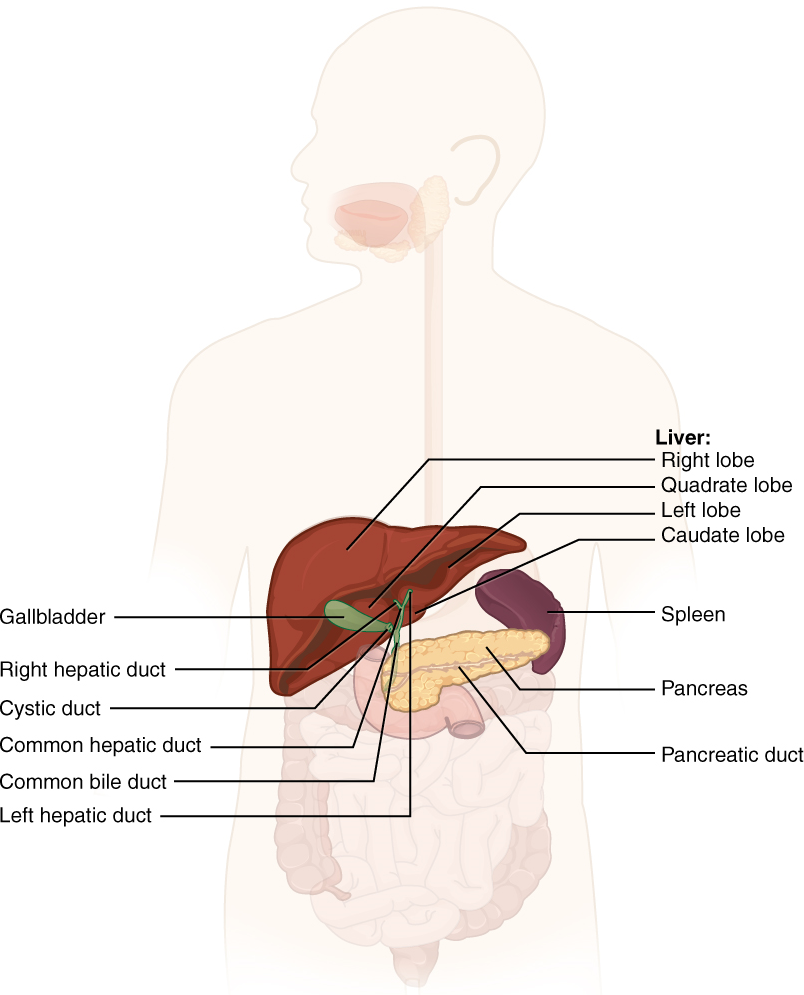
The Liver
The liver is the largest gland in the body, weighing about three pounds in an adult. It is also one of the most important organs. In addition to being an accessory digestive organ, it plays a number of roles in metabolism and regulation. The liver lies inferior to the diaphragm in the right upper quadrant of the abdominal cavity and receives protection from the surrounding ribs.
The liver is divided into two primary lobes: a large right lobe and a much smaller left lobe. In the right lobe, some anatomists also identify an inferior quadrate lobe and a posterior caudate lobe, which are defined by internal features. The liver is connected to the abdominal wall and diaphragm by five peritoneal folds referred to as ligaments. These are the falciform ligament, the coronary ligament, two lateral ligaments, and the ligamentum teres hepatis. The falciform ligament and ligamentum teres hepatis are actually remnants of the umbilical vein, and separate the right and left lobes anteriorly. The lesser omentum tethers the liver to the lesser curvature of the stomach.
The porta hepatis (“gate to the liver”) is where the hepatic artery and hepatic portal vein enter the liver. These two vessels, along with the common hepatic duct, run behind the lateral border of the lesser omentum on the way to their destinations. The hepatic artery delivers oxygenated blood from the heart to the liver (Figure 21). The hepatic portal vein delivers partially deoxygenated blood containing nutrients absorbed from the small intestine and actually supplies more oxygen to the liver than do the much smaller hepatic arteries. In addition to nutrients, drugs and toxins are also absorbed. After processing the bloodborne nutrients and toxins, the liver releases nutrients needed by other cells back into the blood, which drains into the central vein and then through the hepatic vein to the inferior vena cava. With this hepatic portal circulation, all blood from the alimentary canal passes through the liver.
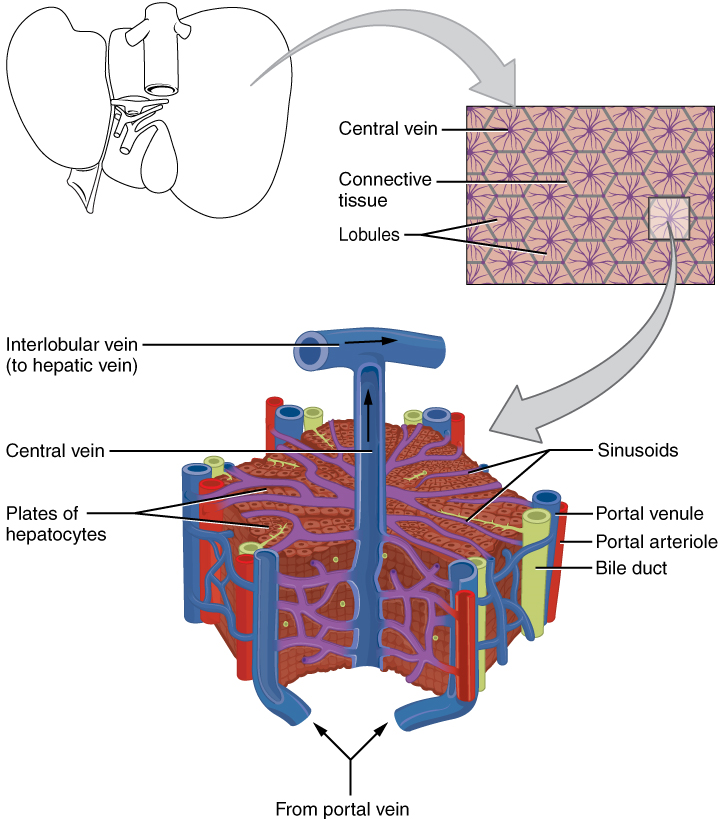
Bile: Recall that lipids are hydrophobic, that is, they do not dissolve in water. Thus, before they can be digested in the watery environment of the small intestine, large lipid globules must be broken down into smaller lipid globules, a process called emulsification. Bile is a mixture secreted by the liver to accomplish the emulsification of lipids in the small intestine.
Hepatocytes secrete about one litre of bile each day. A yellow-brown or yellow-green alkaline solution (pH 7.6 to 8.6), bile is a mixture of water, bile salts, bile pigments, phospholipids (such as lecithin), electrolytes, cholesterol, and triglycerides. The components most critical to emulsification are bile salts and phospholipids, which have a nonpolar (hydrophobic) region as well as a polar (hydrophilic) region. The hydrophobic region interacts with the large lipid molecules, whereas the hydrophilic region interacts with the watery chyme in the intestine. This results in the large lipid globules being pulled apart into many tiny lipid fragments of about 1 µm in diameter. This change dramatically increases the surface area available for lipid-digesting enzyme activity. This is the same way dish soap works on fats mixed with water.
Bile salts act as emulsifying agents, so they are also important for the absorption of digested lipids. While most constituents of bile are eliminated in feces, bile salts are reclaimed by the enterohepatic circulation. Once bile salts reach the ileum, they are absorbed and returned to the liver in the hepatic portal blood. The hepatocytes then excrete the bile salts into newly formed bile. Thus, this precious resource is recycled.
Bilirubin, the main bile pigment, is a waste product produced when the spleen removes old or damaged red blood cells from the circulation. These breakdown products, including proteins, iron, and toxic bilirubin, are transported to the liver via the splenic vein of the hepatic portal system. In the liver, proteins and iron are recycled, whereas bilirubin is excreted in the bile. It accounts for the green color of bile. Bilirubin is eventually transformed by intestinal bacteria into stercobilin, a brown pigment that gives your stool its characteristic color! In some disease states, bile does not enter the intestine, resulting in white (‘acholic’) stool with a high fat content, since virtually no fats are broken down or absorbed.
Hepatocytes work non-stop, but bile production increases when fatty chyme enters the duodenum and stimulates the secretion of the gut hormone secretin. Between meals, bile is produced but conserved. The valve-like hepatopancreatic ampulla closes, allowing bile to divert to the gallbladder, where it is concentrated and stored until the next meal.
The Pancreas
The soft, oblong, glandular pancreas lies transversely in the retroperitoneum behind the stomach. Its head is nestled into the “c-shaped” curvature of the duodenum with the body extending to the left about 15.2 cm (6 in) and ending as a tapering tail in the hilum of the spleen. It is a curious mix of exocrine (secreting digestive enzymes) and endocrine (releasing hormones into the blood) functions (Figure 22).
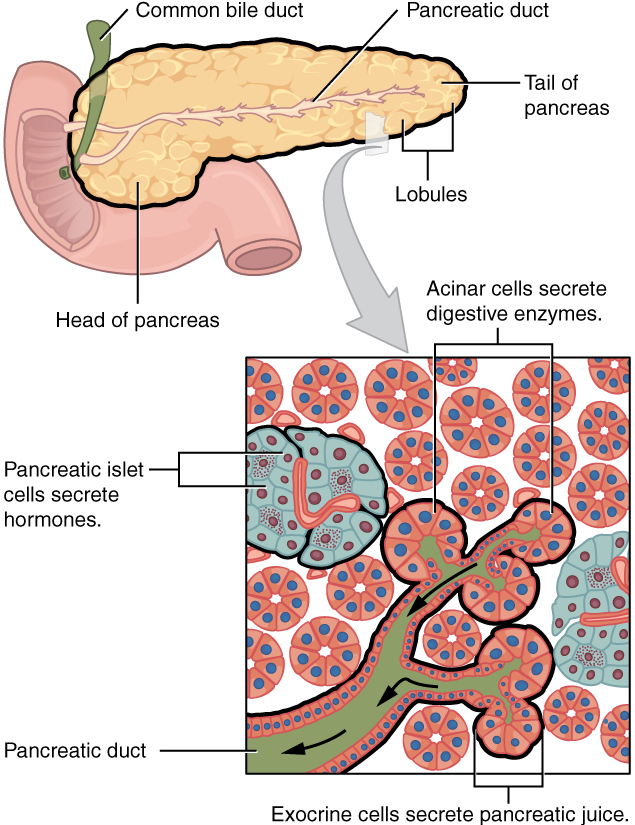
The exocrine part of the pancreas arises as little grape-like cell clusters, each called an acinus (plural = acini), located at the terminal ends of pancreatic ducts. These acinar cells secrete enzyme-rich pancreatic juice into tiny merging ducts that form two dominant ducts. The larger duct fuses with the common bile duct (carrying bile from the liver and gallbladder) just before entering the duodenum via a common opening (the hepatopancreatic ampulla). The smooth muscle sphincter of the hepatopancreatic ampulla controls the release of pancreatic juice and bile into the small intestine. The second and smaller pancreatic duct, the accessory duct (duct of Santorini), runs from the pancreas directly into the duodenum, approximately 1 inch above the hepatopancreatic ampulla. When present, it is a persistent remnant of pancreatic development.
Scattered through the sea of exocrine acini are small islands of endocrine cells, the islets of Langerhans. These vital cells produce the hormones pancreatic polypeptide, insulin, glucagon, and somatostatin.
Pancreatic Juice: The pancreas produces over a litre of pancreatic juice each day. Unlike bile, it is clear and composed mostly of water along with some salts, sodium bicarbonate, and several digestive enzymes. Sodium bicarbonate is responsible for the slight alkalinity of pancreatic juice (pH 7.1 to 8.2), which serves to buffer the acidic gastric juice in chyme, inactivate pepsin from the stomach, and create an optimal environment for the activity of pH-sensitive digestive enzymes in the small intestine. Pancreatic enzymes are active in the digestion of sugars, proteins, and fats.
The pancreas produces protein-digesting enzymes in their inactive forms. These enzymes are activated in the duodenum. If produced in an active form, they would digest the pancreas (which is exactly what occurs in the disease, pancreatitis). The intestinal brush border enzyme enteropeptidase stimulates the activation of trypsin from trypsinogen of the pancreas, which in turn changes the pancreatic enzymes procarboxypeptidase and chymotrypsinogen into their active forms, carboxypeptidase and chymotrypsin.
The enzymes that digest starch (amylase), fat (lipase), and nucleic acids (nuclease) are secreted in their active forms, since they do not attack the pancreas as do the protein-digesting enzymes.
Pancreatic Secretion: Regulation of pancreatic secretion is the job of hormones and the parasympathetic nervous system. The entry of acidic chyme into the duodenum stimulates the release of secretin, which in turn causes the duct cells to release bicarbonate-rich pancreatic juice. The presence of proteins and fats in the duodenum stimulates the secretion of cholecystokinin, which then stimulates the acini to secrete enzyme-rich pancreatic juice and enhances the activity of secretin. Parasympathetic regulation occurs mainly during the cephalic and gastric phases of gastric secretion, when vagal stimulation prompts the secretion of pancreatic juice.
Usually, the pancreas secretes just enough bicarbonate to counterbalance the amount of HCl produced in the stomach. Hydrogen ions enter the blood when bicarbonate is secreted by the pancreas. Thus, the acidic blood draining from the pancreas neutralizes the alkaline blood draining from the stomach, maintaining the pH of the venous blood that flows to the liver.
The Gallbladder
The gallbladder is 8–10 cm (~3–4 in) long and is nested in a shallow area on the posterior aspect of the right lobe of the liver. This muscular sac stores, concentrates, and, when stimulated, propels the bile into the duodenum via the common bile duct. It is divided into three regions. The fundus is the widest portion and tapers medially into the body, which in turn narrows to become the neck. The neck angles slightly superiorly as it approaches the hepatic duct. The cystic duct is 1–2 cm long and turns inferiorly as it bridges the neck and hepatic duct.
The simple columnar epithelium of the gallbladder mucosa is organized in rugae, similar to those of the stomach. There is no submucosa in the gallbladder wall. The wall’s middle, muscular coat is made of smooth muscle fibres. When these fibres contract, the gallbladder’s contents are ejected through the cystic duct and into the bile duct (Figure 23). Visceral peritoneum reflected from the liver capsule holds the gallbladder against the liver and forms the outer coat of the gallbladder. The gallbladder’s mucosa absorbs water and ions from bile, concentrating it by up to 10-fold.
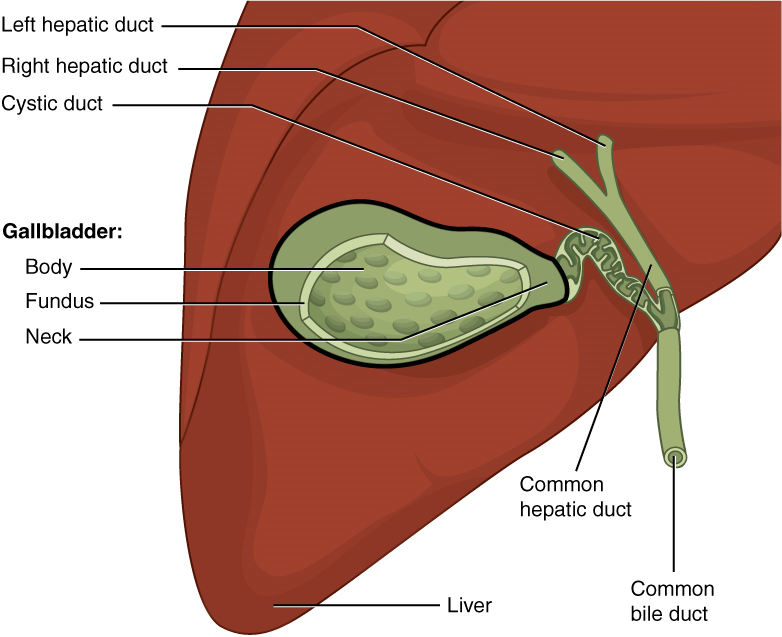
Part 7: Nutrition
Essential nutrients
In addition to providing chemical energy, ingested foodstuffs must also provide any molecules that cannot be produced fast enough (or in some cases, at all) by the body to meet the body’s needs. Such molecules are referred to as essential because they must be ingested to allow normal functioning of the human body.
There are two essential fatty acids that humans must ingest: linoleic acid (LA), an omega-6 fatty acid, and linolenic acid (ALA), an omega-3 fatty acid. These two fatty acids serve as precursor molecules that can be modified by the body, particularly in the liver, to produce other lipid molecules. However, they cannot be created from other molecules in the human body and so must be provided by consuming an external source.
There are eight essential amino acids that humans must ingest from other sources: tryptophan, methionine, valine, threonine, phenylalanine, leucine, isoleucine, and lysine. An additional two – histidine and arginine – are essential for infants but not for adults. Any protein that contains in its primary structure any of these amino acids will not be made at all in their absence. All of the essential amino acids are found in animal product proteins (e.g. eggs, milk, fish, most meats), but almost no single plant source contains all of the essential amino acids, with the exception of soybean and quinoa. However, combinations of plants can be ingested together to provide them; for example, a combination of cereal grains (e.g. corn) and legumes (e.g. beans) can provide all eight essential amino acids.
Although humans do produce it as a byproduct of cellular respiration, water is also an essential nutrient. We lose far more water through constant evaporation from our breath, mucous membranes, and sweat than is produced. Thus humans must ingest water regularly. Plant and animal cells consist largely of water, so a substantial amount of water can be obtained from (non-dehydrated) dietary sources. Nevertheless, humans living in all but the most comfortable of environments typically require access to a source of additional liquid water in addition to plant and animal sources. Excessive water loss (dehydration) can be fatal from a combination of an inability to sweat allowing a dangerous rise in body temperature and a dramatic drop in blood volume and increase in blood viscosity due to water loss from the blood plasma. Under extreme conditions (e.g. exercising strenuously in a hot environment) the lack of a reliable water sources can prove fatal within a few hours; an adult in comfortable surroundings could survive up to about a week without any water intake before succumbing. Generally, the lack of other dietary nutrients in an otherwise health human would not prove fatal nearly as quickly.
The other essential nutrients are the vitamins and minerals. Vitamins in general must be ingested directly or produced by modifying specific precursor molecules that can be ingested instead, but they are required and cannot be produced from other types of nutrients. Minerals are inorganic ions and as such cannot be ‘produced’ in the human body at all and must be ingested in an appropriate form.
Vitamins
Vitamins are organic compounds found in foods and are a necessary part of the biochemical reactions in the body. They are involved in a number of processes, including mineral and bone metabolism, and cell and tissue growth, and they act as cofactors for energy metabolism. The B vitamins play the largest role of any vitamins in metabolism (Table 4 and Table 5).
You get most of your vitamins through your diet, although some can be formed from the precursors absorbed during digestion. For example, the body synthesizes vitamin A from the β-carotene in orange vegetables like carrots and sweet potatoes. Vitamins are either fat-soluble or water-soluble. Fat-soluble vitamins A, D, E, and K are absorbed through the intestinal tract with lipids in chylomicrons. Vitamin D is also synthesized in the skin through exposure to sunlight. Because they are carried in lipids, fat-soluble vitamins can accumulate in the lipids stored in the body. If excess vitamins are retained in the lipid stores in the body, hypervitaminosis can result leading to toxic symptoms depending on the vitamin.
Water-soluble vitamins, including the eight B vitamins and vitamin C, are absorbed with water in the gastrointestinal tract. These vitamins move easily through bodily fluids, which are water based, so they are not stored in the body. Excess water-soluble vitamins are excreted in the urine. Therefore, hypervitaminosis of water-soluble vitamins rarely occurs, except with an excess of vitamin supplements.
Vitamin and alternative name | Sources | Recommended daily allowance | Functions | Problems associated with deficiency |
---|---|---|---|---|
B1
thiamine |
Whole grains, enriched bread/cereals, milk, meat | 1.1-1.2 mg | Synthesis of pyruvate dehydrogenase for carbohydrate metabolism (pyruvate → acetyl CoA) | Beriberi (decreased muscle function, mental confusion, shortness of breath during exercise, paralysis)
Wernicke-Korsakoff syndrome (balance and movement issues, cardiovascular issues, including increased blood pressure when standing) |
B2
riboflavin |
Brewer’s yeast, almonds, milk, organ meats, legumes, enriched breads/cereals, broccoli, asparagus | 1.1-1.3 mg | Synthesis of FAD for metabolism; production of erythrocytes | Fatigue, slowed growth, digestive problems, light sensitivity, epithelial problems like cracks in the corners of the mouth |
B3
niacin |
Meat, fish, poultry, enriched breads/cereals, peanuts | 14-16 mg | Synthesis of NAD+ for metabolism; nerve function, cholesterol production | Pellagra (cracked, scaly skin; mouth sores; dementia; diarrhea) |
B5
pantothenic acid |
Meat, poultry, potatoes, oats, enriched breads/cereals, tomatoes | 5 mg | Synthesis of coenzyme A for metabolism | Rare; fatigue, insomnia, depression, irritability |
B6
pyridoxine |
Potatoes, bananas, beans, seeds, nuts, meat, poultry, fish, eggs, dark green leafy vegetables, soy, organ meats | 1.3-1.5 mg | Sodium/potassium balance, erythrocyte synthesis, amino acid metabolism, glycogenolysis and gluconeogenesis, ceramide synthesis | Confusion, irritability, depression, mouth/tongue sores |
B7
biotin |
Liver, fruits, meats | 30 μg | Cell growth, fatty acid metabolism, blood cell production | Rare in developed countries; dermatitis, hair loss, loss of muscular coordination |
B9
folic acid |
Liver, legumes, dark green leafy vegetables, enriched breads/cereals, citrus fruits | 400 μg | DNA/protein synthesis | Poor growth, gingivitis, appetite loss, shortness of breath, gastrointestinal problems, mental deficits |
B12
cyanobalamin |
Fish, meat, poultry, dairy products, eggs | 2.4 μg | Fatty acid oxidation, nerve cell function, erythrocyte production | Pernicious anemia leading to nerve cell damage |
C
ascorbic acid |
Citrus fruits, red berries, peppers, tomatoes, broccoli, dark green leafy vegetables | 75-90 mg | Collagen production (for formation of connective tissues and teeth, and for wound healing) | Dry hair, gingivitis, bleeding gums, dry/scaly skin, slow wound healing, easy bruising, compromised immunity; can lead to scurvy |
Vitamin and alternative name | Sources | Recommended daily allowance | Functions | Problems associated with deficiency |
---|---|---|---|---|
A
retinal or β-carotene |
Yellow/orange fruits/vegetables, dark green leafy vegetables, eggs, milk, liver | 700-900 μg | Eye & bone development, immune function | Night blindness, epithelial changes, immune system deficiency |
D
cholecalciferol |
Dairy products, egg yolks; synthesis in skin using sunlight | 5-15 μg | Aids in calcium & phosphorus absorption, thereby promoting bone growth | Rickets, bone pain, muscle weakness, increased risk of death from cardiovascular disease, cognitive impairment, asthma in children, cancer |
E
tocopherols |
Seeds, nuts, vegetable oils, avocados, wheat germ | 15 mg | Antioxidant | Anemia |
K
phylloquinone |
Dark green leafy vegetables, broccoli, Brussels sprouts, cabbage | 90-120 μg | Blood clotting, bone health | Hemorrhagic disease of newborn in infants; uncommon in adults |
Minerals
Minerals in food are inorganic ions or compounds that work with other nutrients to ensure the body functions properly. Minerals cannot be made in the body; they come from the diet. The amount of minerals in the body is small—only 4 percent of the total body mass—and most of that consists of the minerals that the body requires in moderate quantities: potassium, sodium, calcium, phosphorus, magnesium, and chloride.
The most common minerals in the body are calcium and phosphorous, both of which are stored in the skeleton and necessary for the hardening of bones. Most minerals are ionized, and their ionic forms are used in physiological processes throughout the body. Sodium and chloride ions are electrolytes in the blood and extracellular tissues, and iron ions are critical to the formation of hemoglobin. There are additional trace minerals (not included in Table 6) that are still important to the body’s functions, but their required quantities are much lower.
Like vitamins, minerals can be consumed in toxic quantities (although it is rare). A healthy diet includes most of the minerals your body requires, so supplements and processed foods can add potentially toxic levels of minerals. Table 6 provides a summary of the major minerals and their function in the body.
Mineral | Sources | Recommended daily allowance | Functions | Problems associated with deficiency |
---|---|---|---|---|
Potassium (K+) | Meats, some fish, fruits, vegetables, legumes, dairy products | 4700 mg | Nerve & muscle function, electrolyte | Hypokalemia (weakness, fatigue, muscle cramping, gastrointestinal problems, cardiac problems) |
Sodium (Na+) | Table salt, milk, beets, celery, processed foods | 2300 mg | Blood pressure, blood volume, nerve & muscle function, electrolyte | Rare |
Calcium (Ca2+) | Dairy products, dark green leafy vegetables, blackstrap molasses, nuts, brewer’s yeast, some fish | 1000 mg | Bone structure & health; nerve & muscle functions, especially cardiac function, electrolyte | Slow growth, weak and brittle bones |
Phosphorus (P, usually as phosphate PO43-) | Meat, milk | 700 mg | Bone formation, metabolism, ATP production | Rare |
Magnesium (Mg2+) | Whole grains, nuts, leafy green vegetables | 310-420 mg | Enzyme activation, ATP production, regulation of other nutrients | Agitation, anxiety, sleep problems, nausea/vomiting, abnormal hearth rhythms, low blood pressure, muscular problems |
Chloride (Cl–) | Most foods; table salt; vegetables, especially seaweed, tomatoes, lettuce, celery, olives | 2300 mg | Balance of body fluids, digestion, electrolyte | Loss of appetite, muscle cramps |
Part 8: Chemical Digestion and Absorption: A Closer Look
As you have learned, the process of mechanical digestion is relatively simple. It involves the physical breakdown of food but does not alter its chemical makeup. Chemical digestion, on the other hand, is a complex process that reduces food into its chemical building blocks, which are then absorbed to nourish the cells of the body (Figure 24). In this section, you will look more closely at the processes of chemical digestion and absorption.
Chemical Digestion
Large food molecules (for example, proteins, lipids, nucleic acids, and starches) must be broken down into subunits that are small enough to be absorbed by the lining of the alimentary canal. This is accomplished by enzymes through hydrolysis. The many enzymes involved in chemical digestion are summarized in Table 7.
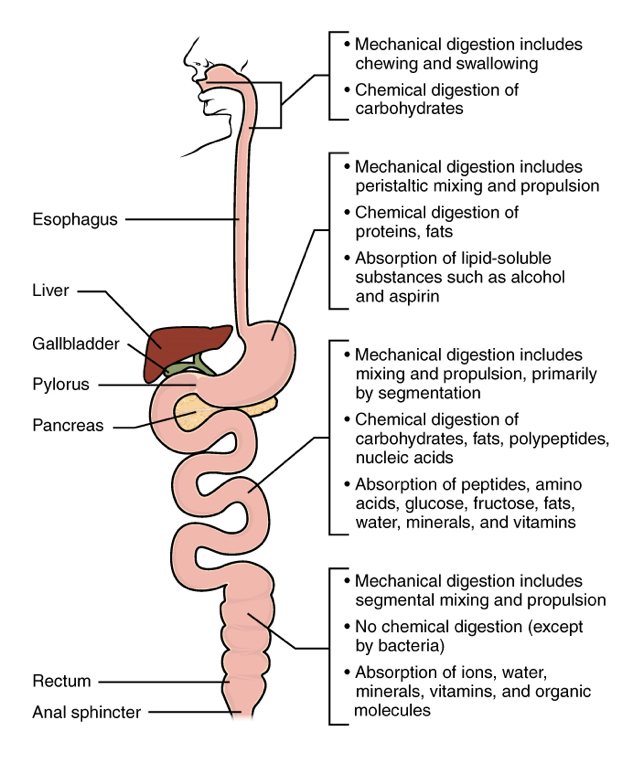
Enzyme category | Enzyme name | Source | Substrate | Product |
---|---|---|---|---|
Salivary enzymes | Lingual lipase | Lingual glands | Triglycerides | Free fatty acids + diglycerides |
Salivary enzymes | Salivary amylase | Salivary glands | Polysaccharides (starch, glycogen) | Maltose (and dextrins) |
Gastric enzymes | Gastric lipase | Chief cells | Triglycerides | Fatty acids + monoglycerides |
Gastric enzymes | Pepsin | Chief cells | Proteins | Peptides |
Brush border enzymes | Lactase | Small intestine | Lactose | Glucose + galactose |
Brush border enzymes | Maltase | Small intestine | Maltose | Glucose |
Brush border enzymes | Sucrase | Small intestine | Sucrose | Glucose + fructose |
Brush border enzymes | Nucleotidases & phosphatases | Small intestine | Nucleotides | Phosphate ions + nitrogenous bases + pentoses |
Brush border enzymes | Peptidases | Small intestine | Aminopeptidase: amino acids at amino end of peptides
Carboxypeptidase: amino acids at carboxyl end of peptides Dipeptidase: dipeptides Enteropeptidase: trypsinogen |
Aminopeptidase & carboxypeptidase: amino acids + peptides
Dipeptidase: amino acids Enteropeptidase: trypsin |
Pancreatic enzymes | Carboxypeptidase | Acinar cells | Amino acids at carboxyl end of proteins/polypeptides | Amino acids + peptides |
Pancreatic enzymes | Chymotrypsin (released as chymotrypsinogen) | Acinar cells | Proteins/polypeptides | Peptides |
Pancreatic enzymes | Trypsin (released as trypsinogen) | Acinar cells | Proteins/polypeptides (including chymotrypsinogen) | Peptides (including chymotrypsin) |
Pancreatic enzymes | Nucleases | Acinar cells | Ribonuclease: ribonucleic acids
Deoxyribonuclease: deoxyribonucleic acids |
Nucleotides |
Pancreatic enzymes | Pancreatic amylase | Acinar cells | Polysaccharides (starch, glycogen) | Maltose (and dextrins) |
Pancreatic enzymes | Pancreatic lipase | Acinar cells | Triglycerides | Free fatty acids + diglycerides |
Carbohydrate Digestion
The average Canadian diet is about 50 percent carbohydrates, which may be classified according to the number of monomers they contain of simple sugars (monosaccharides and disaccharides) and/or complex sugars (polysaccharides). Glucose, galactose, and fructose are the three monosaccharides that are commonly consumed and are readily absorbed.
Your digestive system is also able to break down the disaccharides sucrose (regular table sugar: glucose + fructose), lactose (milk sugar: glucose + galactose), and maltose (grain sugar: glucose + glucose), and the polysaccharides glycogen and starch (chains of monosaccharides). Your bodies do not produce enzymes that can break down most fibrous polysaccharides, such as cellulose. While indigestible polysaccharides do not provide any nutritional value, they do provide dietary fibre, which helps propel food through the alimentary canal.
The chemical digestion of starches begins in the mouth, where salivary amylase acts on starch (Table 3). There is little further chemical digestion of carbohydrates until they reach the small intestine.
In the small intestine, pancreatic amylase does the ‘heavy lifting’ for starch and carbohydrate digestion (Figure 25). After amylases break down starch into smaller fragments, the brush border enzymes continue the process. Three brush border enzymes hydrolyze sucrose, lactose, and maltose into monosaccharides. Sucrase splits sucrose into one molecule of fructose and one molecule of glucose; maltase breaks down maltose and maltotriose into two and three glucose molecules, respectively; and lactase breaks down lactose into one molecule of glucose and one molecule of galactose. Insufficient lactase can lead to lactose intolerance.
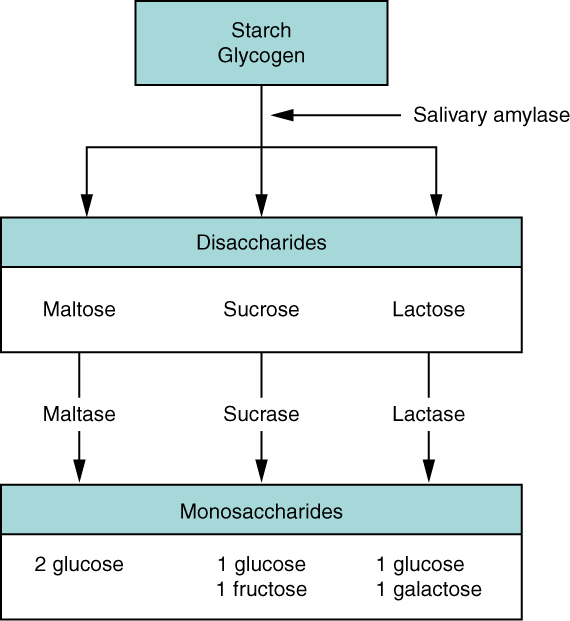
Protein Digestion
Proteins are polymers composed of amino acids linked by peptide bonds to form long chains. Digestion reduces them to their constituent amino acids. You usually consume about 15 to 20 percent of your total calorie intake as protein.
The digestion of protein starts in the stomach, where pepsin breaks proteins into smaller polypeptides, which then travel to the small intestine (Figure 26). Chemical digestion in the small intestine is continued by pancreatic enzymes, including chymotrypsin and trypsin, each of which act on specific bonds in amino acid sequences. At the same time, the cells of the brush border secrete enzymes such as aminopeptidase, carboxypeptidase and dipeptidase, which further break down peptide chains. This results in molecules small enough to enter the bloodstream (Figure 27).
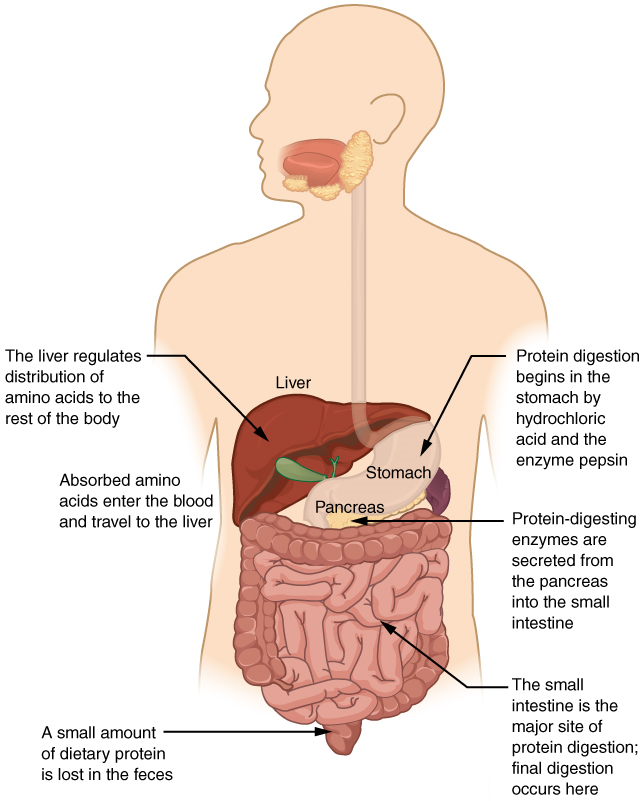
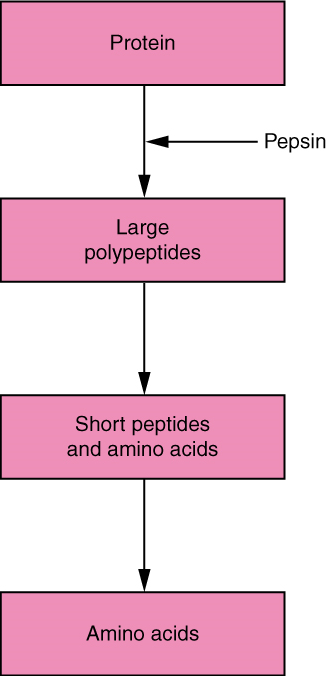
Lipid Digestion
A healthy diet limits lipid intake to 35 percent of total calorie intake. The most common dietary lipids are triglycerides, which are made up of a glycerol molecule bound to three fatty acid chains. Small amounts of dietary cholesterol and phospholipids are also consumed.
The three lipases responsible for lipid digestion are lingual lipase, gastric lipase, and pancreatic lipase. However, because the pancreas is the only consequential source of lipase, virtually all lipid digestion occurs in the small intestine. Pancreatic lipase breaks down each triglyceride into two free fatty acids and a monoglyceride.
Nucleic Acid Digestion
The nucleic acids DNA and RNA are found in most of the foods you eat. Two types of pancreatic nuclease are responsible for their digestion: deoxyribonuclease, which digests DNA, and ribonuclease, which digests RNA. The nucleotides produced by this digestion are further broken down by two intestinal brush border enzymes (nucleosidase and phosphatase) into pentoses, phosphates, and nitrogenous bases, which can be absorbed through the alimentary canal wall.
The large food molecules that must be broken down into subunits are summarized Table 8.
Source | Substance |
---|---|
Carbohydrates | Monosaccharides: glucose, galactose, fructose |
Proteins | Amino acids, dipeptides, tripeptides |
Triglycerides | Monoglycerides, glycerol, free fatty acids |
Nucleic acids | Pentose sugars, phosphates, nitrogenous bases |
Absorption
The mechanical and digestive processes have one goal: to convert food into molecules small enough to be absorbed by the epithelial cells of the intestinal villi. The absorptive capacity of the alimentary canal is almost endless. Each day, the alimentary canal processes up to 10 liters of food, liquids, and GI secretions, yet less than one litre enters the large intestine. Almost all ingested food, 80 percent of electrolytes, and 90 percent of water are absorbed in the small intestine. Although the entire small intestine is involved in the absorption of water and lipids, most absorption of carbohydrates and proteins occurs in the jejunum. Notably, bile salts and vitamin B12 are absorbed in the terminal ileum. By the time chyme passes from the ileum into the large intestine, it is essentially indigestible food residue (mainly plant fibres like cellulose), some water, and millions of bacteria (Figure 28).
Absorption can occur through five mechanisms: (1) active transport, (2) passive diffusion, (3) facilitated diffusion,(4) co-transport (or secondary active transport), and (5) endocytosis (Review 1103/1109 Cell Membrane transport unit here: https://pressbooks.bccampus.ca/dcbiol110311092nded/chapter/unit-5-cell-biology-membrane-transport/). As you will recall, active transport refers to the movement of a substance across a cell membrane going from an area of lower concentration to an area of higher concentration (up the concentration gradient). In this type of transport, proteins within the cell membrane act as “pumps,” using cellular energy (ATP) to move the substance. Passive diffusion refers to the movement of substances from an area of higher concentration to an area of lower concentration, while facilitated diffusion refers to the movement of substances from an area of higher to an area of lower concentration using a carrier protein in the cell membrane. Co-transport uses the movement of one molecule through the membrane from higher to lower concentration to power the movement of another from lower to higher. Finally, endocytosis is a transportation process in which the cell membrane engulfs material. It requires energy, generally in the form of ATP.
Because the cell’s plasma membrane is made up of hydrophobic phospholipids, water-soluble nutrients must use transport molecules embedded in the membrane to enter cells. Moreover, many substances cannot pass between the epithelial cells of the intestinal mucosa because these cells are bound together by tight junctions. Thus, nutrients generally enter blood capillaries by passing through the apical surface of epithelial cells and then out the basal surface into the interstitial fluid. Water-soluble nutrients then enter the capillary blood in the villi and travel to the liver via the hepatic portal vein.
In contrast to the water-soluble nutrients, lipid-soluble nutrients can diffuse through the plasma membrane of an intestinal epithelial cell. Once inside the cell, they are packaged for transport, exit via the base of the cell, and then enter the lacteals of the villi to be transported by lymphatic vessels to the systemic circulation via the thoracic duct. The absorption of most nutrients through the mucosa of the intestinal villi requires active transport fueled by ATP. The routes of absorption for each food category are summarized in Table 9.
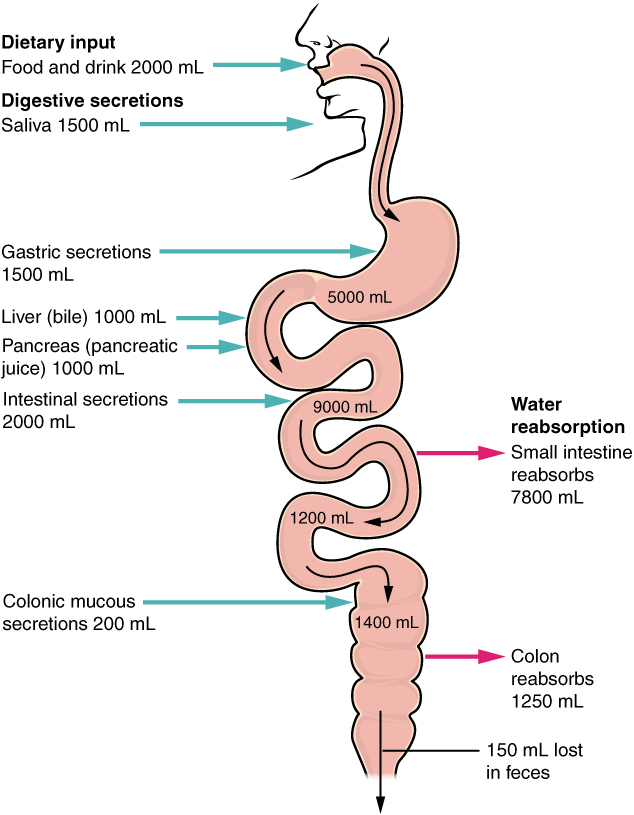
Carbohydrate Absorption: All carbohydrates are absorbed in the form of monosaccharides. The small intestine is highly efficient at this, absorbing monosaccharides at an estimated rate of 120 grams per hour. All normally digested dietary carbohydrates are absorbed; indigestible fibres are eliminated in the feces. The monosaccharides glucose and galactose are transported into the epithelial cells by common protein carriers via secondary active transport (that is, co-transport with sodium ions). The monosaccharides leave these cells via facilitated diffusion and enter the capillaries through intercellular clefts. The monosaccharide fructose (which is in fruit) is absorbed and transported by facilitated diffusion alone. The monosaccharides combine with the transport proteins immediately after the disaccharides are broken down.
Protein Absorption: Active transport mechanisms, primarily in the duodenum and jejunum, absorb most proteins as their breakdown products, amino acids. Almost all (95 to 98 percent) protein is digested and absorbed in the small intestine. The type of carrier that transports an amino acid varies. Most carriers are linked to the active transport of sodium. Short chains of two amino acids (dipeptides) or three amino acids (tripeptides) are also transported actively. However, after they enter the absorptive epithelial cells, they are broken down into their amino acids before leaving the cell via facilitated diffusion.
Food | Breakdown products | Absorption mechanism | Entry to bloodstream | Destination |
Carbohydrates | Glucose | Co-transport with Na+, facilitated diffusion out of intestinal epithelial cells | Diffusion through pores of fenestrated capillaries in villi | Liver (via hepatic portal vein) |
Carbohydrates | Galactose | Co-transport with Na+, facilitated diffusion out of intestinal epithelial cells | Diffusion through pores of fenestrated capillaries in villi | Liver (via hepatic portal vein) |
Carbohydrates | Fructose | Facilitated diffusion | Diffusion through pores of fenestrated capillaries in villi | Liver (via hepatic portal vein) |
Protein | Amino acids | Co-transport with Na+, facilitated diffusion out of intestinal epithelial cells | Diffusion through pores of fenestrated capillaries in villi | Liver (via hepatic portal vein) |
Lipids | Long-chain fatty acids | Simple diffusion into intestinal epithelial cells, exocytosis of chylomicrons out of intestinal epithelial cells | Paracellular transport into lacteals in villi, to left subclavian vein via lymphatic vessels | Systemic circulation via lymph entering thoracic duct |
Lipids | Monoglycerides | Simple diffusion into intestinal epithelial cells, exocytosis of chylomicrons out of intestinal epithelial cells | Paracellular transport into lacteals in villi, to left subclavian vein via lymphatic vessels | Systemic circulation via lymph entering thoracic duct |
Lipids | Short-chain fatty acids | Simple diffusion | Simple diffusion into, and diffusion through pores of, fenestrated capillaries in villi | Liver (via hepatic portal vein) |
Lipids | Glycerol | Simple diffusion | Simple diffusion into, and diffusion through pores of, fenestrated capillaries in villi | Liver (via hepatic portal vein) |
Nucleic acids | Nitrogenous bases, ribose, deoxyribose, phosphate | Active transport into intestinal epithelial cells, facilitated diffusion out of intestinal epithelial cells; also paracellular transport | Diffusion through pores of fenestrated capillaries in villi | Liver (via hepatic portal vein) |
Lipid Absorption: About 95 percent of lipids are absorbed in the small intestine. Bile salts not only speed up lipid digestion, they are also essential to the absorption of the end products of lipid digestion. Short-chain fatty acids (under 6 carbon atoms in length) are relatively water soluble and can enter the absorptive cells (enterocytes) directly. Despite being hydrophobic, the small size of short-chain fatty acids enables them to be absorbed by enterocytes via simple diffusion, and then take the same path as monosaccharides and amino acids into the blood capillary of a villus.
The large and hydrophobic long-chain fatty acids and monoacylglycerides are not so easily suspended in the watery intestinal chyme. However, bile salts and lecithin resolve this issue by enclosing them in a micelle, which is a tiny sphere with polar (hydrophilic) ends facing the watery environment and hydrophobic tails turned to the interior, creating a receptive environment for the long-chain fatty acids. The core also includes cholesterol and fat-soluble vitamins. Without micelles, lipids would sit on the surface of chyme and never come in contact with the absorptive surfaces of the epithelial cells. Micelles can easily squeeze between microvilli and get very near the luminal cell surface. At this point, lipid substances exit the micelle and are absorbed via simple diffusion.
The free fatty acids and monoacylglycerides that enter the epithelial cells are reincorporated into triglycerides. The triglycerides are mixed with phospholipids and cholesterol, and surrounded with a protein coat. This new complex, called a chylomicron, is a water-soluble lipoprotein. After being processed by the Golgi apparatus, chylomicrons are released from the cell (Figure 29). Too big to pass through the basement membranes of blood capillaries, chylomicrons instead enter the large pores of lacteals. The lacteals come together to form the lymphatic vessels. The chylomicrons are transported in the lymphatic vessels and empty through the thoracic duct into the subclavian vein of the circulatory system. Once in the bloodstream, the enzyme lipoprotein lipase breaks down the triglycerides of the chylomicrons into free fatty acids and glycerol. These breakdown products then pass through capillary walls to be used for energy by cells or stored in adipose tissue as fat. Liver cells combine the remaining chylomicron remnants with proteins, forming lipoproteins that transport cholesterol in the blood.
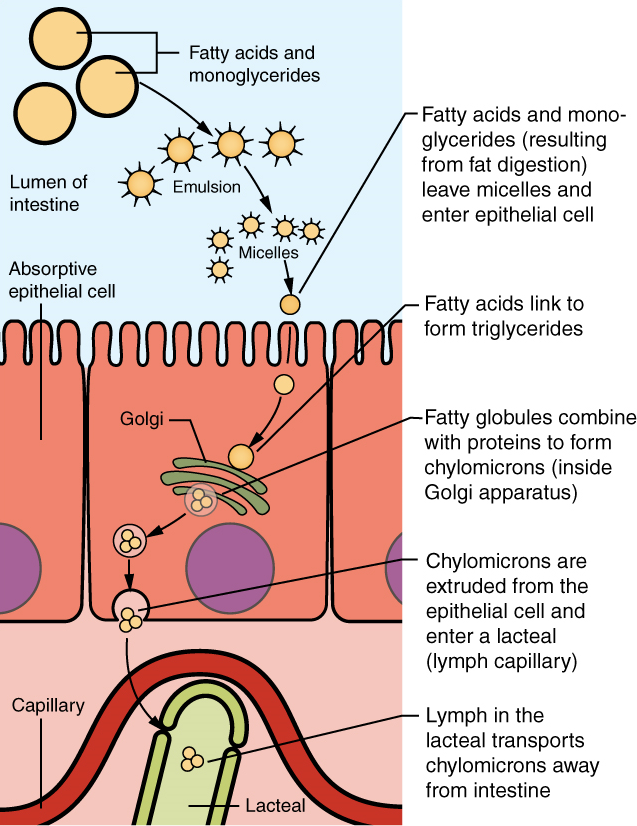
Nucleic Acid Absorption: The products of nucleic acid digestion—pentose sugars, nitrogenous bases, and phosphate ions—are transported by carriers across the villus epithelium via active transport. These products then enter the bloodstream.
Mineral Absorption: The electrolytes absorbed by the small intestine are from both GI secretions and ingested foods. Since electrolytes dissociate into ions in water, most are absorbed via active transport throughout the entire small intestine. During absorption, co-transport mechanisms result in the accumulation of sodium ions inside the cells, whereas anti-port mechanisms reduce the potassium ion concentration inside the cells. To restore the sodium-potassium gradient across the cell membrane, a sodium-potassium pump requiring ATP pumps sodium out and potassium in.
In general, all minerals that enter the intestine are absorbed, whether you need them or not. Iron and calcium are exceptions; they are absorbed in the duodenum in amounts that meet the body’s current requirements.
Vitamin Absorption: The small intestine absorbs the vitamins that occur naturally in food and supplements. Fat-soluble vitamins (A, D, E, and K) are absorbed along with dietary lipids in micelles via simple diffusion. This is why you are advised to eat some fatty foods when you take fat-soluble vitamin supplements. Most water-soluble vitamins (including most B vitamins and vitamin C) are absorbed by facilitated diffusion. An exception is vitamin B12, which is a very large molecule. Intrinsic factor secreted in the stomach binds to vitamin B12, preventing its digestion and creating a complex that binds to mucosal receptors in the terminal ileum, where it is taken up by endocytosis.
Water Absorption: Each day, about nine liters of fluid enter the small intestine. About 2.3 liters are ingested in foods and beverages, and the rest is from GI secretions. About 90 percent of this water is absorbed in the small intestine. Water absorption is driven by the concentration gradient of the water: The concentration of water is higher in chyme than it is in epithelial cells. Thus, water moves down its concentration gradient from the chyme into cells. As noted earlier, much of the remaining water is then absorbed in the colon.
Part 1: Overview of the Digestive System
Part 2: Digestive System Processes and Regulation
Part 3: The Mouth, Pharynx, and Esophagus
Part 4: The Stomach
Part 5: The Small and Large Intestines
Part 6: Accessory Organs in Digestion: The Liver, Pancreas, and Gallbladder
Part 7: Nutrition
Part 8: Chemical Digestion and Absorption: A Closer Look
Fluid contained within the lymphatic system.
Secretion of an endocrine organ that travels via the bloodstream or lymphatics to induce a response in target cells or tissues in another part of the body.
Accessory digestive organ that secretes pancreatic juice, and hormones (glucagon and insulin). Located between the stomach and duodenum in the epigastric and left hypochondriac regions of the abdomen.
Alimentary canal organ that contributes to chemical and mechanical digestion of food from the esophagus before releasing it, as chyme, to the small intestine.
Section of the alimentary canal where most digestion and absorption occurs.
Sum of all catabolic and anabolic reactions that take place in the body.
Continuous muscular digestive tube that extends from the mouth to the anus.
Digestive organ that food doesn't pass directly through. Includes teeth, tongue, salivary glands, gallbladder, liver, and pancreas.
Region of the conducting zone that forms a tube of skeletal muscle lined with respiratory epithelium; located between the nasal conchae and the esophagus and trachea.
Chewing, mixing, and segmentation that prepares food for chemical digestion.
Enzymatic breakdown of food.
Accessory digestive organ that stores and concentrates bile. Located beneath the right lobe of the liver.
Largest gland in the body, overlying the stomach, whose functions include bile production and a number of metabolic and regulatory functions.
Alkaline solution produced by the liver and important for the emulsification of lipids.
Molecule (usually a protein) that catalyzes chemical reactions.
Innermost lining of the alimentary canal.
Connective tissue underlying a mucosa.
Muscle (skeletal or smooth) layer of the alimentary canal wall.
Outermost layer of the alimentary canal wall present in regions within the abdominal cavity.
Double-layered extension of the peritoneum that supports most abdominal organs.
Part of a mucous membrane (composed of loose connective tissue) that supports an epithelium.
Layer of skin between the epidermis and hypodermis, composed mainly of connective tissue and containing blood vessels, hair follicles, sweat glands, and other structures.
Type of tissue that serves primarily as a covering or lining of body parts, protecting the body; it also functions in absorption, transport, and secretion.
Muscular tube that runs from the pharynx to the stomach.
Mucous producing cell (a unicellular gland).
Space inside of a tube, hollow organ or blood vessel.
Type of muscle tissue found primarily in the walls of hollow organs.
(Also, plexus of Meissner) nerve supply that regulates activity of glands and smooth muscle.
Voluntary skeletal muscle sphincter in the anal canal.
One of three smooth muscle bands that make up the longitudinal muscle layer of the muscularis in all of the large intestine except the terminal end.
Division of the anterior (ventral) cavity that houses the abdominal viscera.
The most abundant of three protein fibres found in the extracellular matrix of connective tissues.
Lymphatic capillary in the villi.
Alternating contractions and relaxations of non-adjacent segments of the intestine that move food forward and backward, breaking it apart and mixing it with digestive juices.
Referring to solute concentration.
Class of nonpolar organic compounds built from hydrocarbons and distinguished by the fact that they are not soluble in water.
Class of organic compounds that are composed of many amino acids linked together by peptide bonds.
Muscular contractions and relaxations that propel food through the GI tract.
Peptide hormone that stimulates secretion of hydrochloric acid and gut motility.
Gastric gland cell that secretes hydrochloric acid and intrinsic factor.
A hormone produced by enteroendocrine cells of the duodenum in response to acidity. Stimulates liver and pancreatic digestive secretions.
A hormone secreted by enteroendocrine cells of the duodenum in response amino acids, fatty acids and HCl. Stimulate gall bladder contraction (release of stored bile).
(Also, buccal cavity) mouth.
Lip (plural = labia)
Midline mucous membrane fold that attaches the inner surface of the lips to the gums.
Part of the mouth bounded externally by the cheeks and lips, and internally by the gums and teeth.
Opening between the oral cavity and the oropharynx.
Taking food into the GI tract through the mouth.
Digestive enzyme from glands in the tongue that acts on triglycerides.
Bone that forms the lower jaw bone; the only moveable bone of the skull.
Downward projecting, elongated bony process located on the inferior aspect of the skull.
Paired bones that form the lateral, inferior portions of the skull, with squamous, mastoid, and petrous portions.
Small, U-shaped bone located in upper neck that does not contact any other bone.
For gustation (taste), a bump-like projection on the surface of the tongue that contains taste buds.
An exocrine gland that secretes a digestive fluid called saliva.
One of a pair of major salivary glands located in the floor of the mouth.
One of a pair of major salivary glands located beneath the tongue.
One of a pair of major salivary glands located inferior and anterior to the ears.
Proteins with short polysaccharide chains attached.
Digestive enzyme in saliva that acts on starch (a polysaccharide carbohydrate).
HCO3-, an ion produced as a byproduct of metabolism and crucial to regulating pH as part of a chemical buffer system in blood.
PO4-, an ion that is an important component of bone, plasma membranes (phospholipids) and ATP. Regulated by parathyroid hormone (PTH).
(Also, immunoglobulin) antigen-specific protein secreted by plasma cells.
Digestive enzyme with bactericidal properties.
Midline, chisel-shaped tooth used for cutting into food.
(Also, canine) pointed tooth used for tearing and shredding food.
(Also, bicuspid) transitional tooth used for mastication, crushing, and grinding food.
Class of organic compounds built from sugars, molecules containing carbon, hydrogen, and oxygen in a 1-2-1 ratio.
First part of the small intestine, which starts at the pyloric sphincter and ends at the jejunum.
Soupy liquid created (first in the stomach) when food is mixed with digestive juices.
(Also, cardiac region) part of the stomach surrounding the cardiac orifice (esophageal hiatus).
General anatomical term for the part of a hollow organ furthest from its mouth. In the stomach: the uppermost portion, above and to the left of the cardia. In the uterus: domed portion superior to the uterine tubes.
Mid-portion of the stomach.
Lower, funnel-shaped part of the stomach that is continuous with the duodenum.
Wider, more superior part of the pylorus.
Sphincter that controls stomach emptying.
Series of ridges formed by the folding of an organ wall (in stomach for example)(plural= rugae).
Specialized hormone-secreting cell found in the gastrointestinal tract and pancreas.
Glycoprotein required for vitamin B12 absorption in the small intestine.
Gastric gland cell that secretes pepsinogen.
Inactive form of pepsin.
Digestive enzyme that digests protein into peptides and amino acids. Secreted by the chief cells in the stomach, pepsin is the active form of pepsinogen (after it comes in contact with HCl).
Cell that is oligo-, multi-, or pleuripotent that has the ability to produce additional stem cells rather than becoming further specialized.
Vitamin found in meat, fish, dairy and eggs that is necessary for neuron function, hematopoiesis and fatty acid oxidation.
Lipid compound composed of a glycerol molecule bonded with three fatty acid chains.
Serous membrane that lines the abdominopelvic cavity and covers the organs found there.
(Also, ampulla of Vater) bulb-like point in the wall of the duodenum where the bile duct and main pancreatic duct unite.
Tube through which bile travels from the liver and gall bladder to the hepatopancreatic ampulla.
A ring-shaped smooth muscle that can open or close a passage in the body.
Middle part of the small intestine between the duodenum and the ileum.
End of the small intestine between the jejunum and the large intestine.
Sphincter located where the small intestine joins with the large intestine.
Branch of the autonomic nervous system associated with resting systems ("rest and digest").
Branch of the autonomic nervous system associated with emergency systems ("fight of flight").
Projection of the mucosa of the small intestine (plural= villi).
Small projection of the plasma membrane of the absorptive cells of the small intestinal mucosa (plural= microvilli)
(Also, plica circulare) deep fold in the mucosa and submucosa of the small intestine.
Small vessel leading from the capillaries to veins.
Lymphatic capillary in the villi of the intestine.
That part of a cell or tissue which, in general, faces an open space.
The thinnest of the cytoskeletal filaments; composed of actin subunits that function in muscle contraction and cellular structural support.
Fuzzy appearance of the small intestinal mucosa created by microvilli.
(Also, crypt of Lieberkühn) gland in the small intestinal mucosa that secretes intestinal juice.
Mixture of water and mucus that helps absorb nutrients from chyme.
(Also, Brunner’s gland) mucous-secreting gland in the duodenal submucosa.
Hormone that initiates migrating motility complexes.
Pouch forming the beginning of the large intestine.
Diffusion of water molecules down their concentration gradient across a selectively permeable membrane.
Describes a solution concentration that is higher than a reference concentration.
Organic compound required by the body to perform biochemical reactions like metabolism and bone, cell, and tissue growth.
Part of the large intestine between the cecum and the rectum.
Part of the large intestine between the sigmoid colon and anal canal.
Describes a position below or lower than another part of the body proper; near or toward the tail (in humans, the coccyx, or lowest part of the spinal column); also referred to as caudal.
Referring to the sacrum (bone).
Single bone located near the inferior end of the adult vertebral column that is formed by the fusion of five sacral vertebrae; forms the posterior portion of the pelvis.
Small bone located at inferior end of the adult vertebral column that is formed by the fusion of four coccygeal vertebrae; also referred to as the “tailbone”.
One of three transverse folds in the rectum where feces is separated from flatus.
Referring to nervous control of functions without conscious thought,
Relating to or found in the intestines.
Slow segmentation in the large intestine.
Long, slow, peristaltic wave in the large intestine.
Propulsive movement in the colon activated by the presence of food in the stomach.
Anaerobic decomposition of carbohydrates.
Gas in the intestine.
Voluntary contraction of the diaphragm and abdominal wall muscles and closing of the glottis, which increases intra-abdominal pressure and facilitates defecation.
Opening between the vocal folds through which air passes when producing speech.
End portion of the colon, which terminates at the rectum.
Involuntary smooth muscle sphincter in the anal canal.
An infectious agent that causes disease, typically a bacterium, virus, fungus, or microscopic parasite.
Ligament connecting the liver to the anterior body wall.
Double layer of peritoneum extending from the stomach and duodenum to the liver.
“Gateway to the liver” where the hepatic artery and hepatic portal vein enter the liver.
Artery that supplies oxygenated blood to the liver.
Vein that supplies deoxygenated nutrient-rich blood to the liver.
Duct formed by the merger of the two hepatic ducts.
Process of mixing liquids that don't normally mix.
An ionic solution with basic properties (pH >7).
An amphipathic lipid molecule containing a phosphate head (polar) and two fatty acid tails (non-polar). The major molecule comprising plasma membranes.
A solution containing ions.
Recycling mechanism that conserves bile salts.
Main bile pigment, which is responsible for the brown color of feces.
Secondary lymphoid organ that filters pathogens from the blood (white pulp) and removes degenerating or damaged blood cells (red pulp).
Cluster of glandular epithelial cells in the pancreas that secretes pancreatic juice in the pancreas.
Intestinal brush-border enzyme that activates trypsinogen to trypsin.
Enzyme that digests proteins in the small intestine. Activated form of trypsinogen (Activated by enteropeptidase).
Precursor of the digestive enzyme trypsin.
Structure formed by the union of the common hepatic duct and the gallbladder’s cystic duct.
Duct through which bile drains and enters the gallbladder.
A nutrient that cannot be produced by the body (or, at least, in sufficient quantities).
Consisting of a carboxyl group (COOH) and an unbranched hydrocarbon chain. A non-polar component of all lipids.
Building block of proteins; characterized by an amino and carboxyl functional groups and a variable side-chain.
Production of ATP from glucose oxidation via glycolysis, the Krebs cycle, and oxidative phosphorylation.
Inorganic ions or compounds that work with other nutrients to carry out important body functions.
Substance that contains both carbon and hydrogen.
Chemical substance lacking carbon-hydrogen bonds.
Atom with an overall positive or negative charge. Many function as electrolytes.
Oxygen-carrying compound in erythrocytes.
Chemical reaction in which water is used to break one or more chemical bonds.
A "building block" of complex organic molecules. Complex organic molecules are usually composed of a number of similar monomers (e.g. amino acids in protein).
Carbohydrate-digesting enzyme secreted by the pancreas and active in the small intestine.
Nucleotide containing ribose and an adenine base that is essential in energy transfer.