Unit 2: The Heart
Unit outline
- Location of the heart
- Chambers and circulation through the heart
- Membranes, surface features, and layers
- Internal structure of the heart
- Heart valve structure and function
- Coronary circulation
Part 2: Cardiac Muscle and Electrical Activity
Practice Questions
Learning Objectives
At the end of this unit, you should be able to:
I. Describe the anatomy of the human heart with respect to the following: location, size, and shape.
II. Define and describe the location of the following: pericardium, epicardium, myocardium, endocardium.
III. Describe the anatomy, and relationship to each other, of the four chambers of the heart including the location and general makeup of all valves.
IV. Describe the double circulation and blood flow through the heart and explain the role of the four valves in controlling the direction of blood flow.
V. Briefly describe the major components of the coronary circulation and parts of the heart that they feed: left and right coronary artery, coronary veins, coronary sinus.
VI. Specify the components of the conduction system of the heart and describe their functions in the normal conduction of an electrical impulse through the heart and explain the events which constitute and complete the heart beat (i.e. cardiac cycle).
VII. Describe the major components of the human electrocardiogram (ECG) and relate these to the electrical and mechanical events of the heart.
VIII. Describe the following major mechanisms that control heart rate: autonomic system, hormones, ionic composition of the blood, and body temperature.
Learning Objectives and Guiding Questions
At the end of this unit, you should be able to complete all the following tasks, including answering the guiding questions associated with each task.
I. Describe the anatomy of the human heart with respect to the following: location, size, and shape.
- Use correct anatomical terms and complete sentences to describe the position of the human heart in relation to the lungs, diaphragm, vertebral column and thoracic cavity.
II. Define and describe the location of the following: pericardium, epicardium, myocardium, endocardium.
- Draw a simple diagram of the heart wall showing all the following structures, and on your diagram wherever possible, identify the specific tissue type each layer is composed of:
- Pericardium
- Epicardium
- Myocardium
- Endocardium
III. Describe the anatomy and relationship to each other of the four chambers of the heart including the location and general makeup of all valves.
- Distinguish between the upper and lower chambers of the heart
- Describe the partitioning of the heart into left and right chambers.
- Name and describe the structure and location of the valves of the heart
IV. Describe the double circulation and blood flow through the heart and explain the role of the four valves in controlling the direction of blood flow.
- State the names of the two parts of the circulation and their general function in terms of where blood flow is conducted.
- Clearly state the function of each of the four valves found in the human heart. Your description of their function should make reference to the specific location where blood is moving from and to as it passes through each valve.
- Describe the location and function of each of the two main arteries that carry blood out of the heart, and the main veins that carry blood into the human heart.
- List, in order, all the structures through which blood passes as it moves through the heart until it exits, starting from:
- Its entrance into the heart from the venae cavae and coronary sinus
- Its entrance into the heart from the pulmonary veins
- Draw a simplified diagram of the human heart showing the vessels connected directly to its chambers. Show and label all of the following components:
-
- Pericardium
- Epicardium
- Myocardium
- Endocardium
- Right atrium
- Left atrium
- Right ventricle
- Left ventricle
- Tricuspid valve
- Bicuspid valve
- Coronary sinus
- Aorta
- Superior vena cava
- Inferior vena cava
- Chordae tendinae
- Aortic semilunar valve
- Pulmonary semilunar valve
- Pulmonary trunk
- Right pulmonary arteries
- Left pulmonary arteries
- Right pulmonary veins
- Left pulmonary veins
V. Briefly describe the major components of the coronary circulation and parts of the heart that they feed: left and right coronary artery, coronary veins, coronary sinus.
- How are the cardiac muscle fibres of the heart supplied with nutrients?
- How is waste removed from the cardiac muscle fibres of the heart?
VI. Specify the components of the conduction system of the heart and describe their functions in the normal conduction of an electrical impulse through the heart and explain the events which constitute and complete the heart beat (i.e. cardiac cycle).
- Describe, briefly, the general properties of the sinoatrial node (SA Node), atrioventricular node (AV Node), atrioventricular bundle (the bundle of His), right and left bundle branches, and the Purkinje fibres, and the role of each of these in the conduction of a cardiac impulse.
- Describe one heartbeat in detail. Include in your description all the events of the conduction system and the heart muscle, and all the structures the blood passes through (in order!) as it moves through the heart.
- Describe what would happen if the sequence described above is not followed.
- The physical events in the heart that underlie the ‘lub’ and ‘dub’ sounds of a heartbeat, and the relative times when they can be heard.
- Define the terms systole and diastole in relation to contraction of the chambers of the heart.
VII. Describe the major components of the human electrocardiogram (ECG) and relate these to the electrical and mechanical events of the heart.
- Draw and label the major components (P wave, QRS wave, T wave, and the appropriate gaps between them) of a normal ECG tracing, then describe the electrical events in the heart that underlie each wave.
VIII. Describe the following major mechanisms that control heart rate: autonomic system, hormones, ionic composition of the blood, and body temperature.
- Describe the influence of proprioceptors, chemoreceptors, and baroreceptors on the cardiovascular centres in the medulla.
- Describe how hormones modify heart rate.
- Describe how the ionic composition of the blood influences heart rate.
- Describe how body temperature affects heart rate.
In this unit, you will explore the remarkable pump that propels the blood into the vessels. There is no single better word to describe the function of the heart other than “pump,” since its contraction develops the pressure that ejects blood into the major vessels: the aorta and pulmonary trunk. From these vessels, the blood is distributed to the remainder of the body. Although the connotation of the term “pump” suggests a mechanical device made of steel and plastic, the anatomical structure is a living, sophisticated muscle. As you read this chapter, try to keep these twin concepts in mind: pump and muscle.
Although the term “heart” is an English word, cardiac (heart-related) terminology can be traced back to the Latin term, “kardia.” Cardiology is the study of the heart, and cardiologists are the physicians who deal primarily with the heart.
Part 1: Heart Anatomy
The vital importance of the heart is obvious. If one assumes an average rate of contraction of 75 contractions per minute, a human heart would contract approximately 108,000 times in one day, more than 39 million times in one year, and nearly 3 billion times during a 75-year lifespan. Each of the major pumping chambers of the heart ejects approximately 70 mL blood per contraction in a resting adult. This would be equal to 5.25 litres of fluid per minute and approximately 14,000 litres per day. Over one year, that would equal 10,000,000 litres (2.6 million gallons) of blood sent through roughly 96,000 km (60,000 miles) of vessels. In order to understand how that happens, it is necessary to understand the anatomy and physiology of the heart.
Location of the Heart
The human heart is located within the thoracic cavity, medially between the lungs in the space known as the mediastinum (Figure 1). Within the mediastinum, the heart is separated from the other structures by a tough membrane known as the pericardium, or pericardial sac, and sits in its own space called the pericardial cavity. The dorsal surface of the heart lies near the bodies of the vertebrae, and its anterior surface sits deep to the sternum and costal cartilages. The great veins, the superior and inferior venae cavae, and the great arteries, the aorta and pulmonary trunk, are attached to the superior surface of the heart, called the base. The base of the heart is located at the level of the third rib (Figure 1). The inferior tip of the heart, the apex, lies just to the left of the sternum between the junction of the fourth and fifth ribs. The right side of the heart is angled anteriorly, and the left side is angled posteriorly. It is important to remember the position and orientation of the heart when placing a stethoscope on the chest of a patient and listening for heart sounds, and also when looking at images taken from a midsagittal perspective. The slight deviation of the apex to the left is reflected in a depression in the medial surface of the inferior lobe of the left lung, called the cardiac notch (for this structure, refer to Figure 9, Unit 6 – The Respiratory System- https://pressbooks.bccampus.ca/dcbiol120312094thed/chapter/unit-6-the-respiratory-system/).
Shape and Size of the Heart: The shape of the heart is similar to a pinecone, rather broad at the superior surface and tapering to the apex (Figure 1). A typical heart is approximately the size of your fist: 12 cm in length, 8 cm wide, and 6 cm in thickness. Given the size difference between most members of the sexes, the weight of a female heart is approximately 250–300 grams, and the weight of a male heart is approximately 300–350 grams. The heart of a well-trained athlete, especially one specializing in aerobic sports, can be considerably larger than this. Cardiac muscle responds to exercise in a manner similar to that of skeletal muscle. That is, exercise results in the addition of protein myofilaments that increase the size of the individual cells without increasing their numbers, a concept called hypertrophy. Hearts of athletes can pump blood more effectively at lower rates than those of nonathletes.
Chambers and Circulation through the Heart
The human heart consists of four chambers: The left side and the right side each have one atrium and one ventricle. Each of the upper chambers, the right atrium (plural = atria) and the left atrium, acts as a receiving chamber and contracts to push blood into the lower chambers, the right ventricle and the left ventricle, respectively. The ventricles serve as the primary pumping chambers of the heart, propelling blood out to the lungs or to the rest of the body. The atria receive venous blood on a nearly continuous basis, preventing venous flow from stopping while the ventricles are contracting. While most ventricular filling occurs while the atria are relaxed, the atria do demonstrate a contractile phase and actively pump blood into the ventricles just prior to ventricular contraction.
There are two distinct but linked circuits in the human circulation called the pulmonary and systemic circuits. Although both circuits transport blood and everything it carries, we can initially view the circuits from the point of view of gases. The pulmonary circuit transports blood a short distance to and from the lungs, where it picks up oxygen and delivers carbon dioxide for exhalation. The systemic circuit, a longer pathway, transports oxygenated blood to virtually all of the tissues of the body and returns relatively deoxygenated blood and carbon dioxide to the heart to be sent back to the pulmonary circulation.
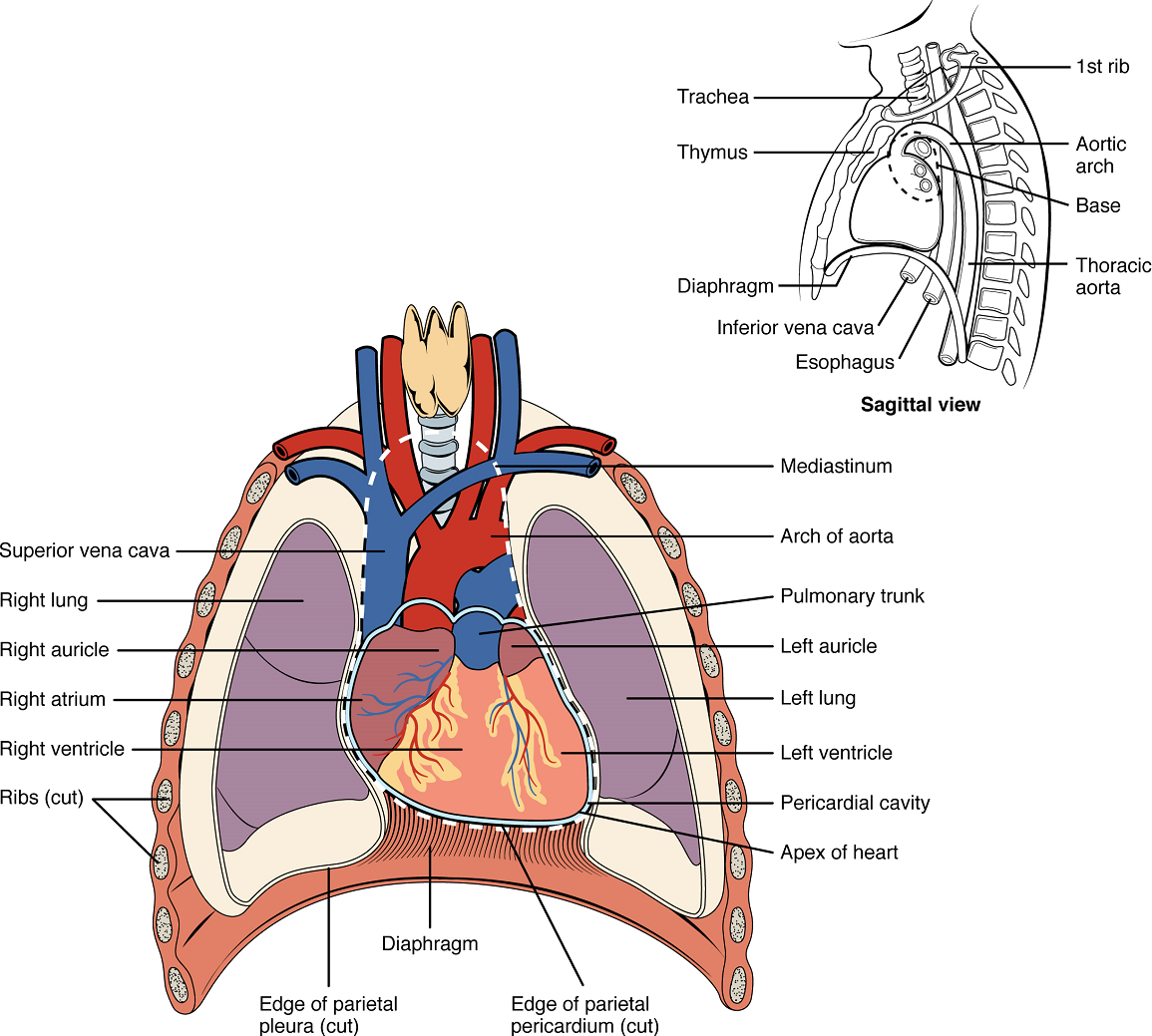
The right ventricle pumps deoxygenated blood into the pulmonary trunk, which leads toward the lungs and bifurcates into the left and right pulmonary arteries. These vessels in turn branch many times before reaching the pulmonary capillaries, where gas exchange occurs: Carbon dioxide exits the blood and oxygen enters. The pulmonary trunk arteries and their branches are the only arteries in the post-natal body that carry relatively deoxygenated blood. Highly oxygenated blood returning from the pulmonary capillaries in the lungs passes through a series of vessels that join together to form the pulmonary veins—the only post-natal veins in the body that carry highly oxygenated blood. The pulmonary veins conduct blood into the left atrium, which pumps the blood into the left ventricle, which in turn pumps oxygenated blood into the aorta and on to the many branches of the systemic circuit. Eventually, these vessels will lead to the systemic capillaries, where exchange with the tissue fluid and cells of the body occurs. In this case, oxygen and nutrients exit the systemic capillaries to be used by the cells in their metabolic processes, and carbon dioxide and waste products will enter the blood.
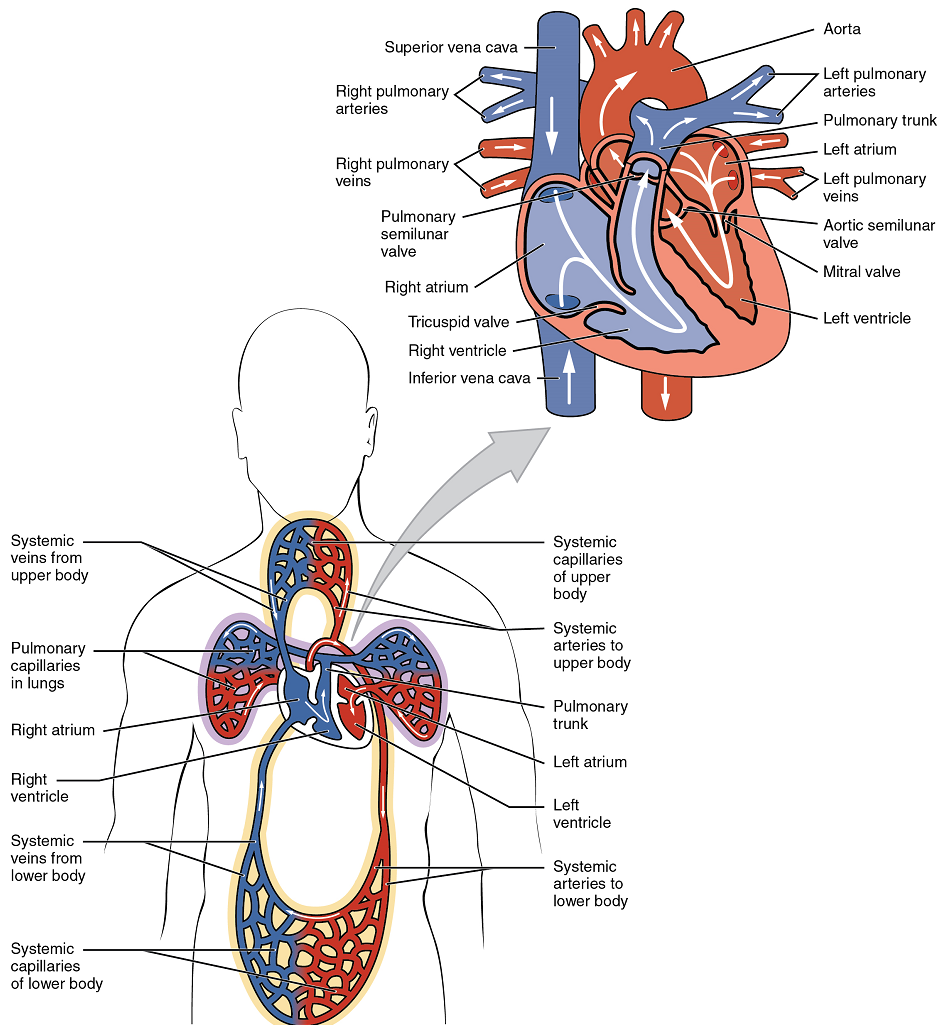
The blood exiting the systemic capillaries is lower in oxygen concentration than when it entered. The capillaries will ultimately unite to form venules, joining to form ever-larger veins, eventually flowing into the two major systemic veins, the superior vena cava and the inferior vena cava, which return blood to the right atrium. The blood in the superior and inferior venae cavae flows into the right atrium, which pumps blood into the right ventricle. This process of blood circulation continues as long as the individual remains alive. Understanding the flow of blood through the pulmonary and systemic circuits is critical to all health professions (Figure 2).
Membranes, Surface Features, and Layers
Our exploration of more in-depth heart structures begins by examining the membrane that surrounds the heart, the prominent surface features of the heart, and the layers that form the wall of the heart. Each of these components plays its own unique role in terms of function.
Membranes: The membrane that directly surrounds the heart and defines the pericardial cavity is called the pericardium or pericardial sac (Figure 3). It also surrounds the “roots” of the major vessels, or the areas of closest proximity to the heart. The pericardium, which literally translates as “around the heart,” consists of two distinct sublayers. The sturdy outer layer is the fibrous pericardium, made of tough, dense connective tissue that protects the heart and maintains its position in the thorax. The inner serous pericardium consists of two layers: the outer parietal pericardium, which is fused to the fibrous pericardium, and an inner visceral pericardium, or epicardium, which is fused to the heart and is part of the heart wall. The pericardial cavity, filled with lubricating serous fluid, lies between the epicardium (visceral pericardium) and the parietal pericardium. Like the other serous membranes in the body, both the visceral and parietal pericardium consist of simple squamous epithelium, but unlike many of these membranes these are reinforced with connective tissue (loose irregular and areolar). The epithelial tissue of the epicardium (visceral pericardium) secretes the lubricating serous fluid that fills the pericardial cavity and reduces friction as the heart contracts.
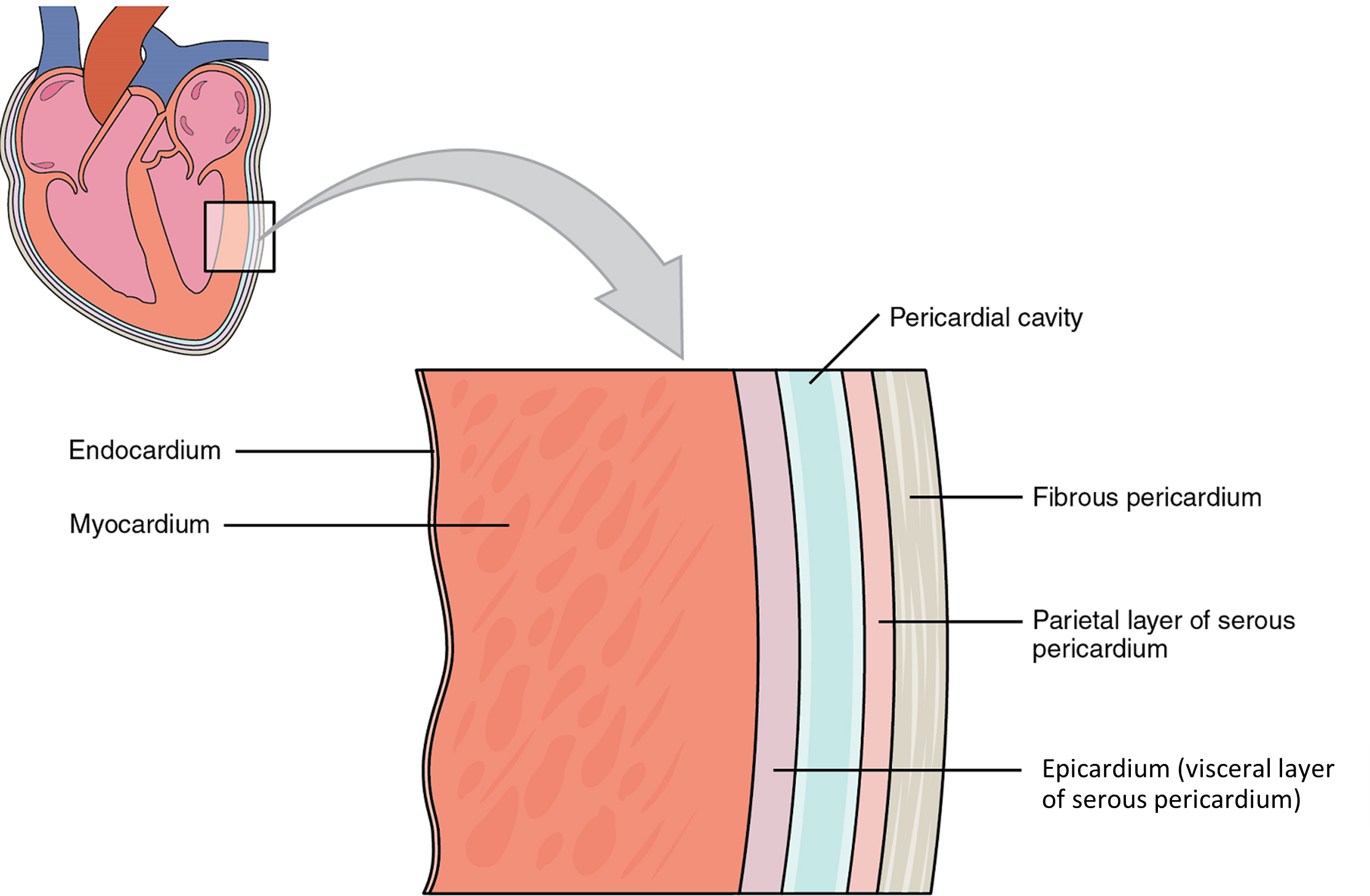
Surface Features of the Heart: Inside the pericardium, the surface features of the heart are visible, including the four chambers (Figure 4). There is a superficial leaf-like extension of the atria near the superior surface of the heart, one on each side, called an auricle—a name that means “ear like”—because its shape resembles the external ear of a human. Auricles are relatively thin-walled structures that can fill with blood and empty into the atria or upper chambers of the heart. You may also hear them referred to as atrial appendages.
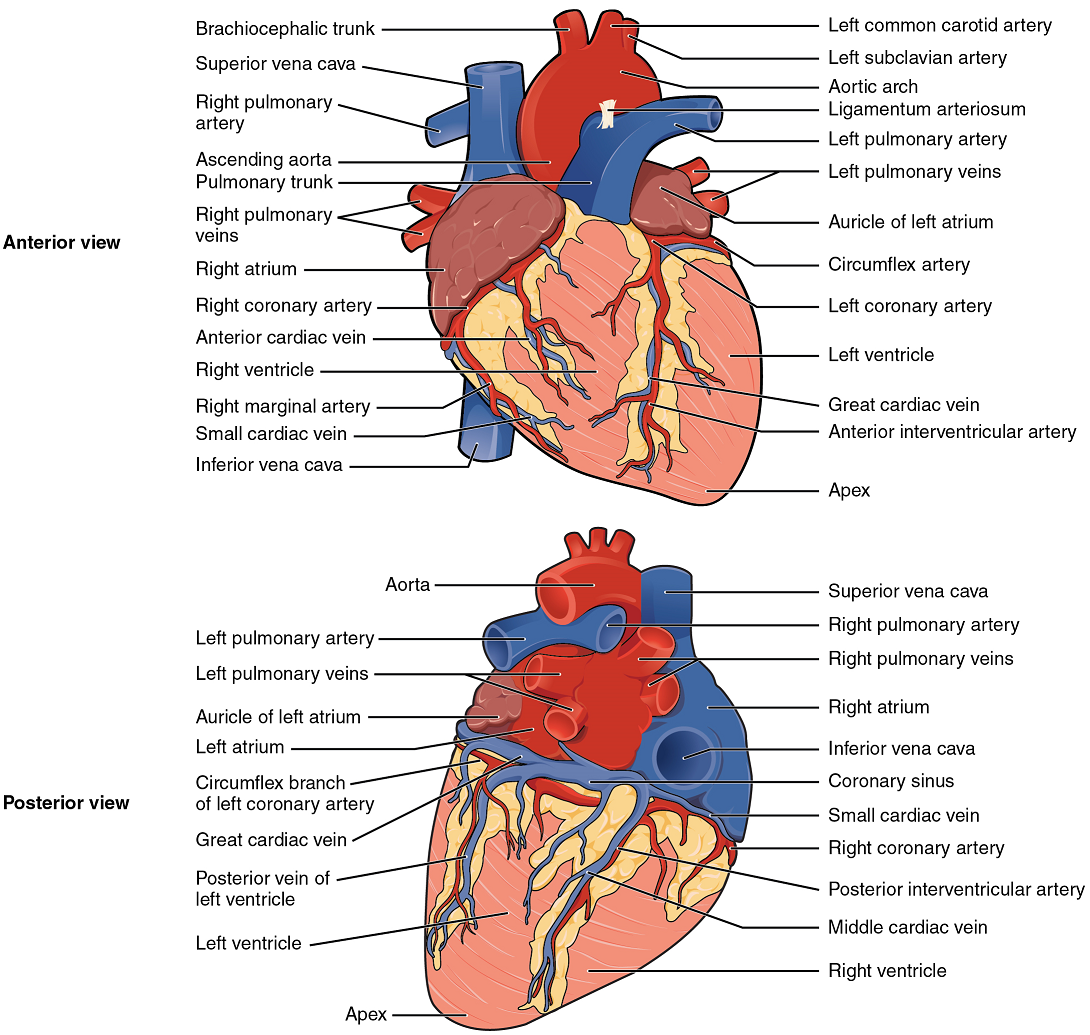
Layers: The wall of the heart is composed of three layers of unequal thickness. From superficial to deep, these are the epicardium, the myocardium, and the endocardium (Figure 3). The outermost layer of the wall of the heart is also the innermost layer of the pericardium, the epicardium, or the visceral pericardium discussed earlier.
The middle and thickest layer is the myocardium, made largely of cardiac muscle cells. It is built upon a framework of collagenous fibres, plus the blood vessels that supply the myocardium and the nerve fibres that help regulate the heart. It is the contraction of the myocardium that pumps blood through the heart and into the major arteries. The muscle pattern is elegant and complex, as the muscle cells swirl and spiral around the chambers of the heart (Figure 5). They form a figure 8 pattern around the atria and around the bases of the great vessels. Deeper ventricular muscles also form a figure 8 around the two ventricles and proceed toward the apex. More superficial layers of ventricular muscle wrap around both ventricles. This complex swirling pattern allows the heart to pump blood more effectively than a simple linear pattern would.
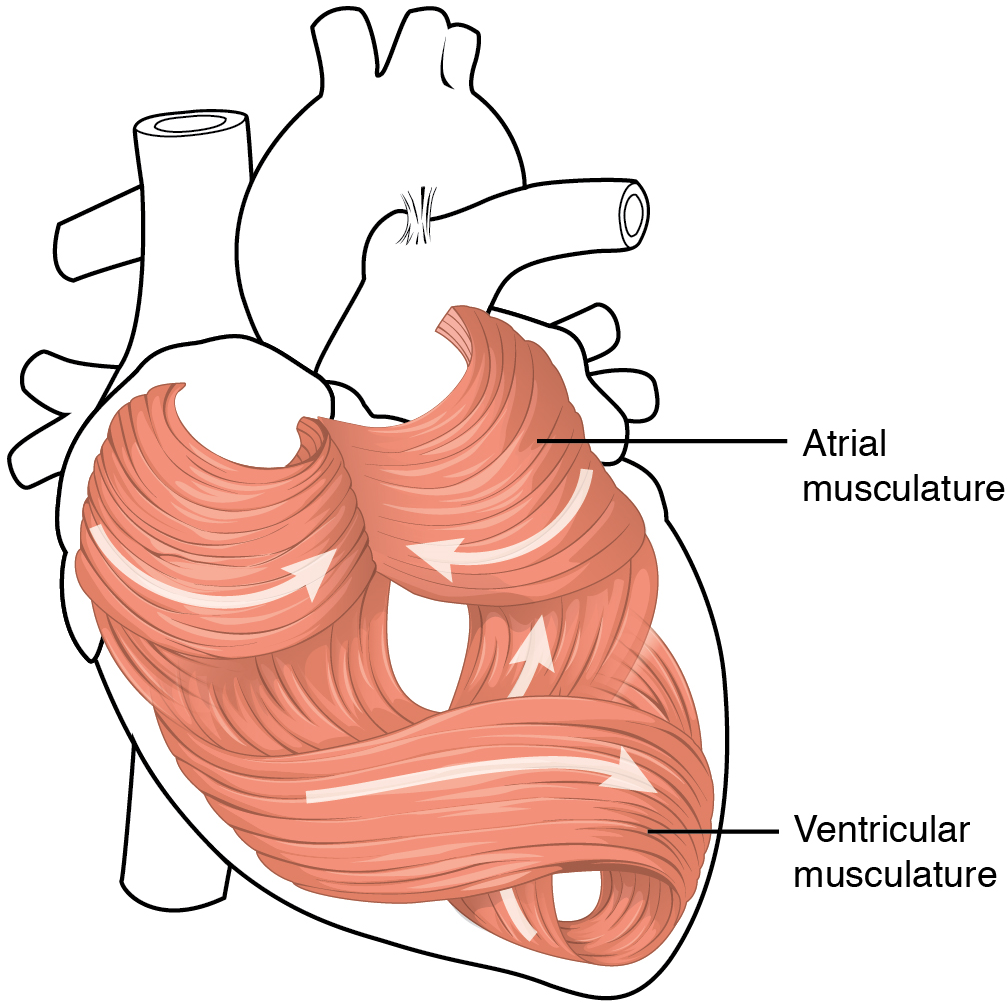
Although the ventricles on the right and left sides pump the same amount of blood per contraction, the muscle of the left ventricle is much thicker and better developed than that of the right ventricle (Figure 6). In order to overcome the high resistance required to pump blood into the long systemic circuit, the left ventricle must generate a greater amount of pressure. The right ventricle does not need to generate as much pressure, since the pulmonary circuit is shorter and provides less resistance.
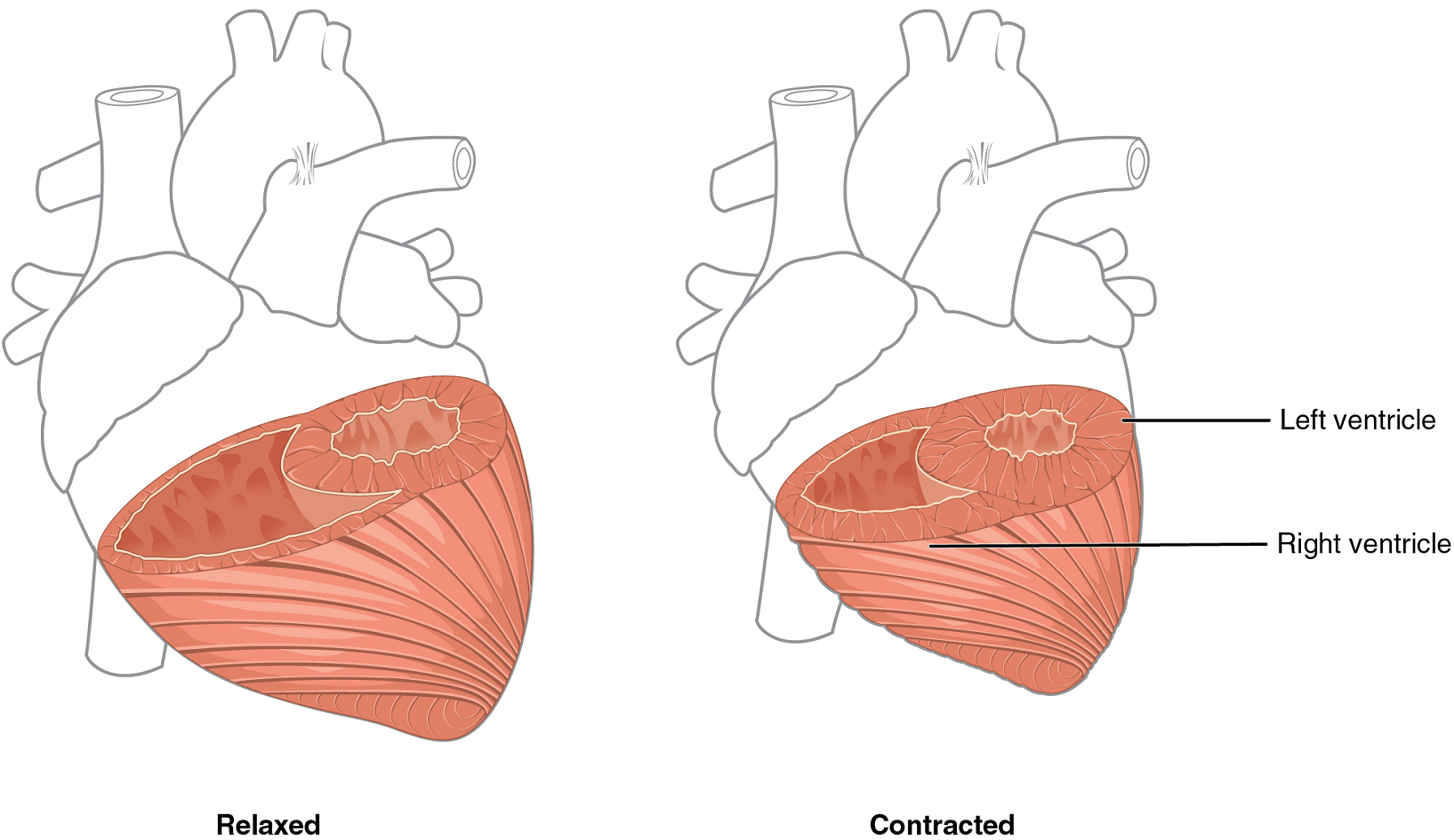
Note the differences in the relative size of the lumens, the region inside each ventricle where the blood is contained.
The innermost layer of the heart wall, the endocardium, is joined to the myocardium with a thin layer of connective tissue. The endocardium lines the chambers where the blood circulates and covers the heart valves. It is made of simple squamous epithelium called endothelium, which is continuous with the endothelial lining of the blood vessels (Figure 3).
Once regarded as a simple lining layer, recent evidence indicates that the endothelium of the endocardium and the coronary capillaries may play active roles in regulating the contraction of the muscle within the myocardium.
Internal Structure of the Heart
Recall that the heart’s contraction cycle follows a dual pattern of circulation—the pulmonary and systemic circuits— because of the pairs of chambers that pump blood into the circulation. In order to develop a more precise understanding of cardiac function, it is first necessary to explore the internal anatomical structures in more detail.
Septa of the Heart: The word septum is derived from the Latin for “something that encloses;” in this case, a septum (plural = septa) refers to a wall or partition that divides the heart into chambers. The septa are physical extensions of the myocardium lined with endocardium. Located between the two atria is the interatrial septum. Normally in an adult heart, the interatrial septum bears an oval-shaped depression known as the fossa ovalis, a remnant of an opening in the fetal heart known as the foramen ovale. The foramen ovale allowed blood in the fetal heart to pass directly from the right atrium to the left atrium, allowing some blood to bypass the pulmonary circuit. Within seconds after birth, a flap of tissue known as the septum primum (that previously acted as a valve) closes the foramen ovale and establishes the typical cardiac circulation pattern.
Between the two ventricles is a second septum known as the interventricular septum (Figure 7). Unlike the interatrial septum, the interventricular septum is normally intact after its formation during fetal development. It is substantially thicker than the interatrial septum, since the ventricles generate far greater pressure when they contract.
The septum between the atria and ventricles is known as the atrioventricular septum. It is marked by the presence of four openings that allow blood to move from the atria into the ventricles and from the ventricles into the pulmonary trunk and aorta. Located in each of these openings between the atria and ventricles is a valve, a specialized structure that ensures one-way flow of blood. The valves between the atria and ventricles are known generically as atrioventricular valves. The valves at the openings that lead to the pulmonary trunk and aorta are known generically as semilunar valves. Since these openings and valves structurally weaken the atrioventricular septum, the remaining tissue is heavily reinforced with dense connective tissue called the cardiac skeleton, or skeleton of the heart.
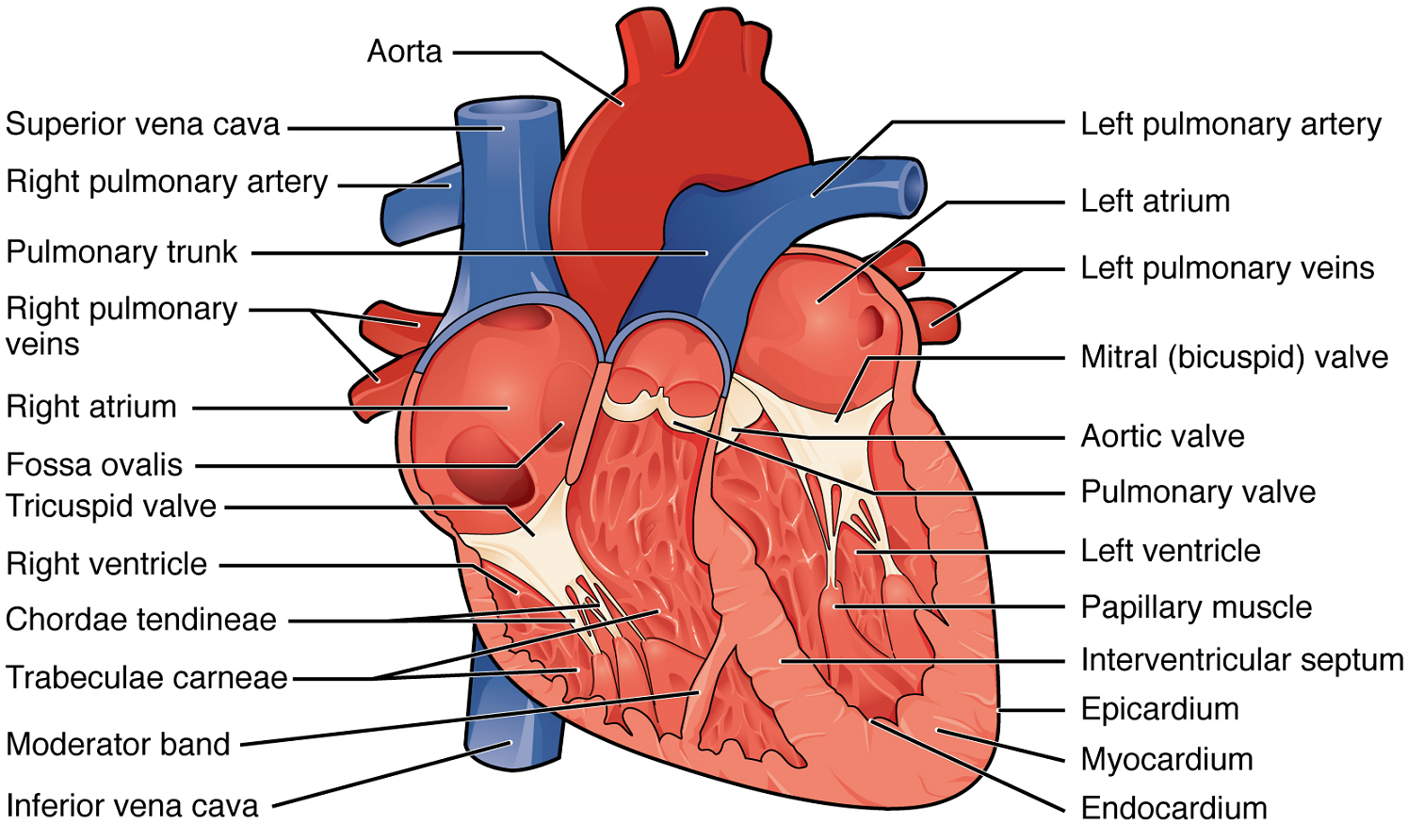
Right Atrium: The right atrium serves as the receiving chamber for blood returning to the heart from the systemic circulation (Figure 7). The two major systemic veins, the superior and inferior venae cavae, and the large coronary vein called the coronary sinus that drains the heart myocardium all empty into the right atrium (Figure 7). The superior vena cava drains blood from regions superior to the diaphragm: the head, neck, upper limbs, and the thoracic region. It empties into the superior and posterior portions of the right atrium. The inferior vena cava drains blood from areas inferior to the diaphragm: the lower limbs and abdominopelvic region of the body. It, too, empties into the posterior portion of the atria, but inferior to the opening of the superior vena cava. Immediately superior and slightly medial to the opening of the inferior vena cava on the posterior surface of the atrium is the opening of the coronary sinus. This thin-walled vessel drains most of the coronary veins that return systemic blood from the heart.
The atria receive venous blood on a nearly continuous basis, preventing venous flow from stopping while the ventricles are contracting. While most ventricular filling occurs while the atria are relaxed, they do demonstrate a contractile phase and actively pump blood into the ventricles just prior to ventricular contraction. The opening between the atrium and ventricle is guarded by the tricuspid valve.
Right Ventricle: The right ventricle receives blood from the right atrium through the tricuspid valve (Figure 7). Each flap of the valve is attached to strong strands of connective tissue, the chordae tendineae, literally “tendinous cords,” or sometimes more poetically referred to as “heart strings” (Figure 8). There are several chordae tendineae associated with each of the flaps. They are composed of approximately 80% collagenous fibres with the remainder consisting of elastic fibres and endothelium. They connect each of the flaps to a papillary muscle that extends from the inferior ventricular surface (Figure 8). There are three papillary muscles in the right ventricle, called the anterior, posterior, and septal muscles, which correspond to the three sections of the valves.
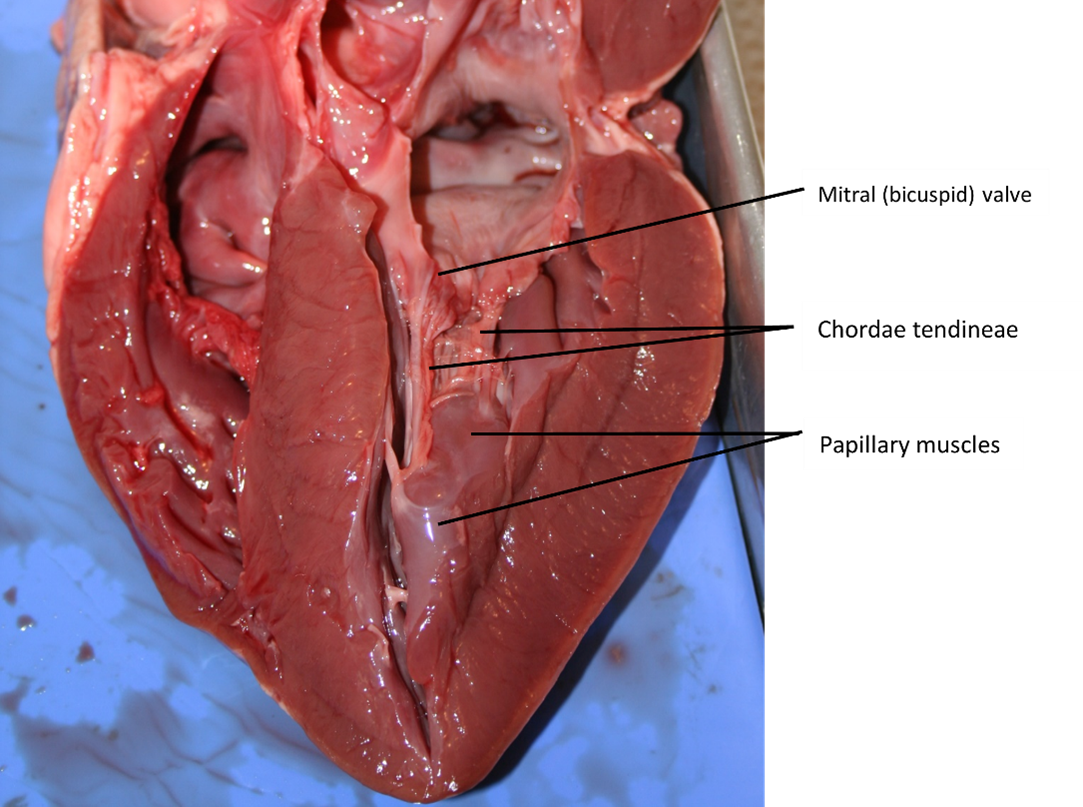
When the myocardium of the ventricle contracts, pressure within the ventricular chamber rises. Blood, like any fluid, flows from higher pressure to lower pressure areas, in this case, toward the pulmonary trunk and the atrium. To prevent any potential backflow, the tricuspid valve also closes. The papillary muscles also contract, generating tension on the chordae tendineae. This prevents the flaps of the valves from being forced into the atria and regurgitation of the blood back into the atria during ventricular contraction.
Left Atrium: After exchange of gases in the pulmonary capillaries, blood returns to the left atrium high in oxygen via one of the four pulmonary veins (Figure 7). Blood flows nearly continuously from the pulmonary veins back into the atrium, which acts as the receiving chamber, and from here through an opening into the left ventricle. Most blood flows passively into the heart while both the atria and ventricles are relaxed, but toward the end of the ventricular relaxation period, the left atrium will contract, pumping blood into the ventricle. This atrial contraction accounts for approximately 20% of ventricular filling. The opening between the left atrium and ventricle is guarded by the mitral valve.
Left Ventricle: Recall that, although both sides of the heart will pump the same amount of blood, the muscular layer is much thicker in the left ventricle compared to the right (Figure 13). The mitral valve is connected to papillary muscles via chordae tendineae. There are two papillary muscles on the left – the anterior and posterior – as opposed to three on the right. The left ventricle is the major pumping chamber for the systemic circuit; it ejects blood into the aorta through the aortic semilunar valve.
Heart Valve Structure and Function
A transverse section through the heart slightly above the level of the atrioventricular septum reveals all four heart valves along the same plane (Figure 9). The valves ensure unidirectional blood flow through the heart. Between the right atrium and the right ventricle is the right atrioventricular valve, or tricuspid valve. It typically consists of three cusps or flaps, or leaflets, made of endocardium reinforced with additional connective tissue. Each flap of the valve is attached to strong strands of connective tissue, the chordae tendineae, literally “tendinous cords,” or sometimes more poetically referred to as “heart strings” (Figure 8). There are several chordae tendineae associated with each of the flaps. They are composed of approximately 80% collagenous fibres with the remainder consisting of elastic fibres and endothelium. They connect each of the flaps to a papillary muscle that extends from the inferior ventricular surface (Figure 8).
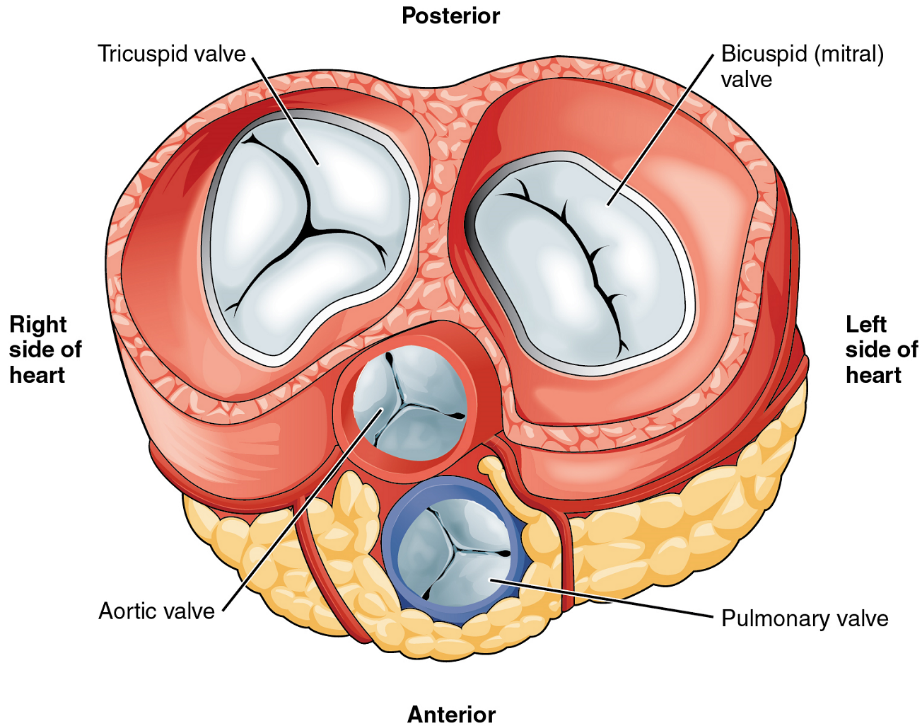
Emerging from the right ventricle at the base of the pulmonary trunk is the pulmonary semilunar valve, or the pulmonary valve; it is also known as the pulmonic valve or the right semilunar valve. The pulmonary valve is comprised of three small flaps of endothelium reinforced with connective tissue. When the ventricle relaxes, the pressure differential causes blood to flow back into the ventricle from the pulmonary trunk. This flow of blood fills the pocket-like flaps of the pulmonary valve, causing the valve to close and producing an audible sound. Unlike the atrioventricular valves, there are no papillary muscles or chordae tendineae associated with the pulmonary valve.
Located at the opening between the left atrium and left ventricle is the mitral valve, also called the bicuspid valve or the left atrioventricular valve. Structurally, this valve consists of two cusps, compared to the three cusps of the tricuspid valve. In a clinical setting, the valve is referred to as the mitral valve, rather than the bicuspid valve. The two cusps of the mitral valve are attached by chordae tendineae to two papillary muscles that project from the wall of the ventricle.
At the base of the aorta is the aortic semilunar valve, or the aortic valve, which prevents backflow from the aorta. It normally is composed of three flaps. When the ventricle relaxes and blood attempts to flow back into the ventricle from the aorta, blood will fill the cusps of the valve, causing it to close and producing an audible sound.
When both atria and ventricles are relaxed, and when the atria contract to pump blood into the ventricles, the atrioventricular valves are open and the semilunar valves are closed (Figure 10).
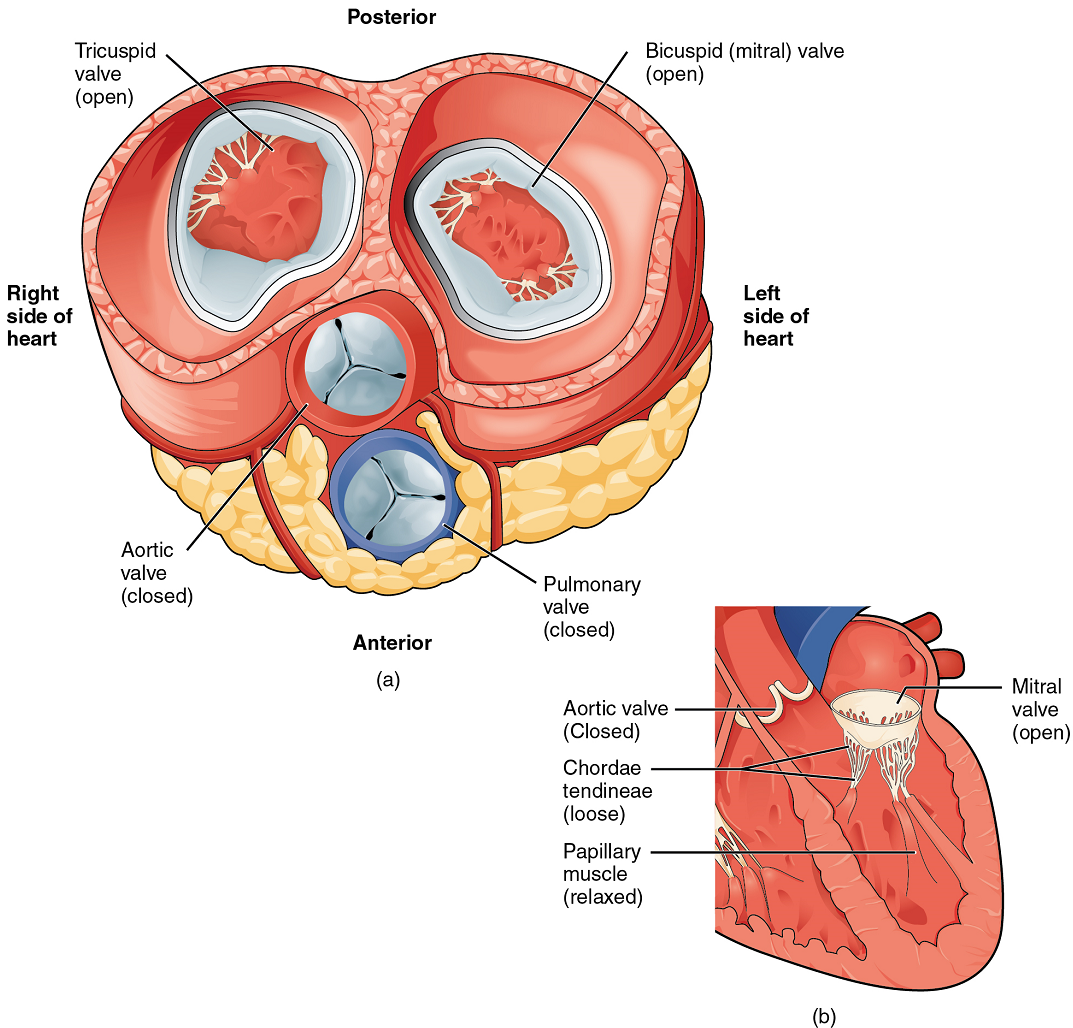
When the ventricles contract to eject blood into the pulmonary trunk and aorta, the atrioventricular valves close and the two semilunar valves open (Figure 11). Closure of the two atrioventricular valves prevents blood from being forced back into the atria.
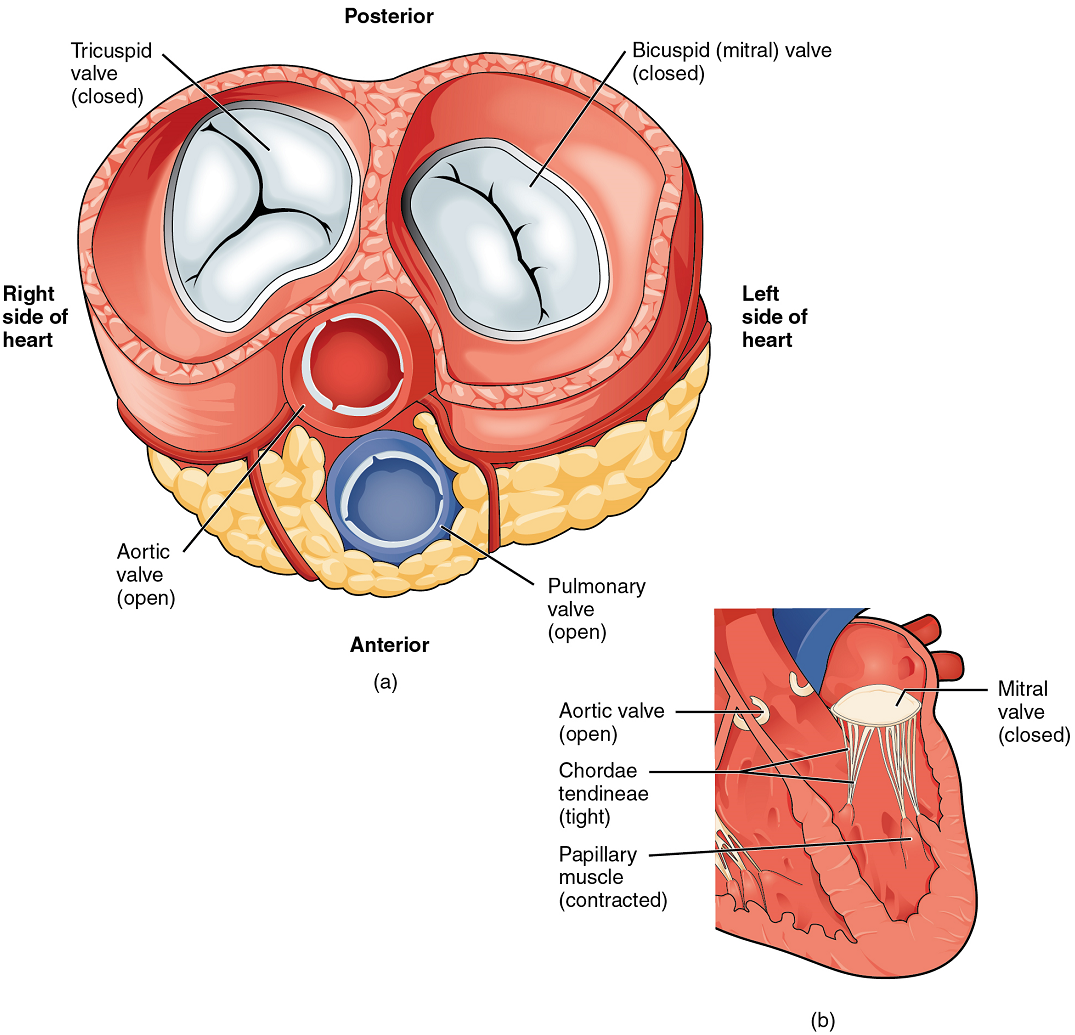
Coronary Circulation
You will recall that the heart is a remarkable pump composed largely of cardiac muscle cells that are incredibly active throughout life. Like all other cells, a cardiomyocyte (cardiac muscle cell) requires a reliable supply of oxygen and nutrients, and a way to remove wastes, so it needs a dedicated, complex, and extensive coronary circulation. And because of the critical and nearly ceaseless activity of the heart throughout life, this need for a blood supply is even greater than for a typical cell. However, coronary circulation is not continuous; rather, it cycles, reaching a peak when the heart muscle is relaxed and nearly ceasing while it is contracting.
Coronary Arteries: Coronary arteries supply blood to the myocardium and other components of the heart. The first portion of the aorta after it arises from the left ventricle gives rise to the left and right coronary arteries (Figure 12).
The left coronary artery distributes blood to the left side of the heart, the left atrium and ventricle, and the interventricular septum. The right coronary artery proceeds along the coronary sulcus and distributes blood to the right atrium, portions of both ventricles, and the heart conduction system (Figure 13). Complete blockage of any coronary artery leads to tissue death and a heart attack (myocardial infarction).
Coronary Veins: Coronary veins drain the heart and generally parallel the large surface arteries (Figure 12). Most drain into the coronary sinus. The coronary sinus is a large, thin-walled vein on the posterior surface of the heart lying within the coronary sulcus and emptying directly into the right atrium.
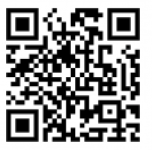
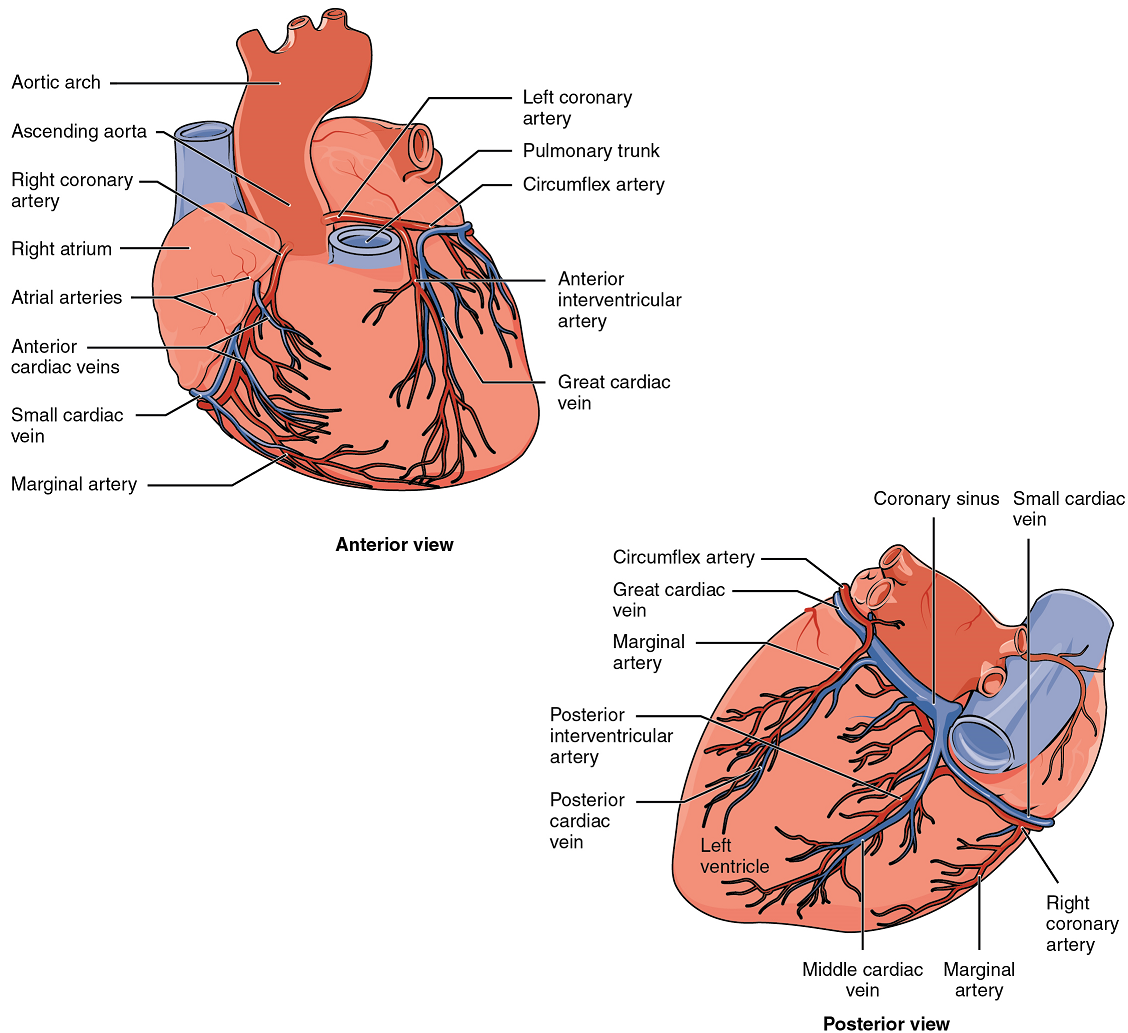
Part 2: Cardiac Muscle and Electrical Activity
Recall that cardiac muscle shares a few characteristics with both skeletal muscle and smooth muscle, but it has some unique properties of its own. Not the least of these exceptional properties is its ability to initiate an electrical potential at a fixed rate that spreads rapidly from cell to cell to trigger the contractile mechanism. This property is known as autorhythmicity. Neither smooth nor skeletal muscle can do this. Even though cardiac muscle has autorhythmicity, heart rate is modulated by the endocrine and nervous systems.
There are two major types of cardiac muscle cells: myocardial contractile cells and myocardial conducting cells. The myocardial contractile cells constitute the bulk (99%) of the cells in the atria and ventricles.
Contractile cells conduct impulses and are responsible for contractions that pump blood through the body. The myocardial conducting cells (1% of the cells) form the conduction system of the heart. Except for Purkinje fibres, they are generally much smaller than the contractile cells and have few of the myofibrils or filaments needed for contraction. Their function is similar in many respects to neurons, although they are specialized muscle cells. Myocardial conduction cells initiate and propagate the action potential (the electrical impulse) that travels throughout the heart and triggers the contractions that propel the blood.
Conduction System of the Heart
If embryonic heart cells are separated into a Petri dish and kept alive, each is capable of generating its own electrical impulse followed by contraction. When two independently beating embryonic cardiac muscle cells are placed together, the cell with the higher inherent rate sets the pace, and the impulse spreads from the faster to the slower cell to trigger a contraction. As more cells are joined together, the fastest cell continues to assume control of the rate. A fully developed adult heart maintains the capability of generating its own electrical impulse, triggered by the fastest cells, as part of the cardiac conduction system. The components of the cardiac conduction system include the sinoatrial node (SA node), the atrioventricular node (AV node), the atrioventricular bundle (bundle of His), the atrioventricular bundle branches, and the Purkinje fibres (Figure 13).
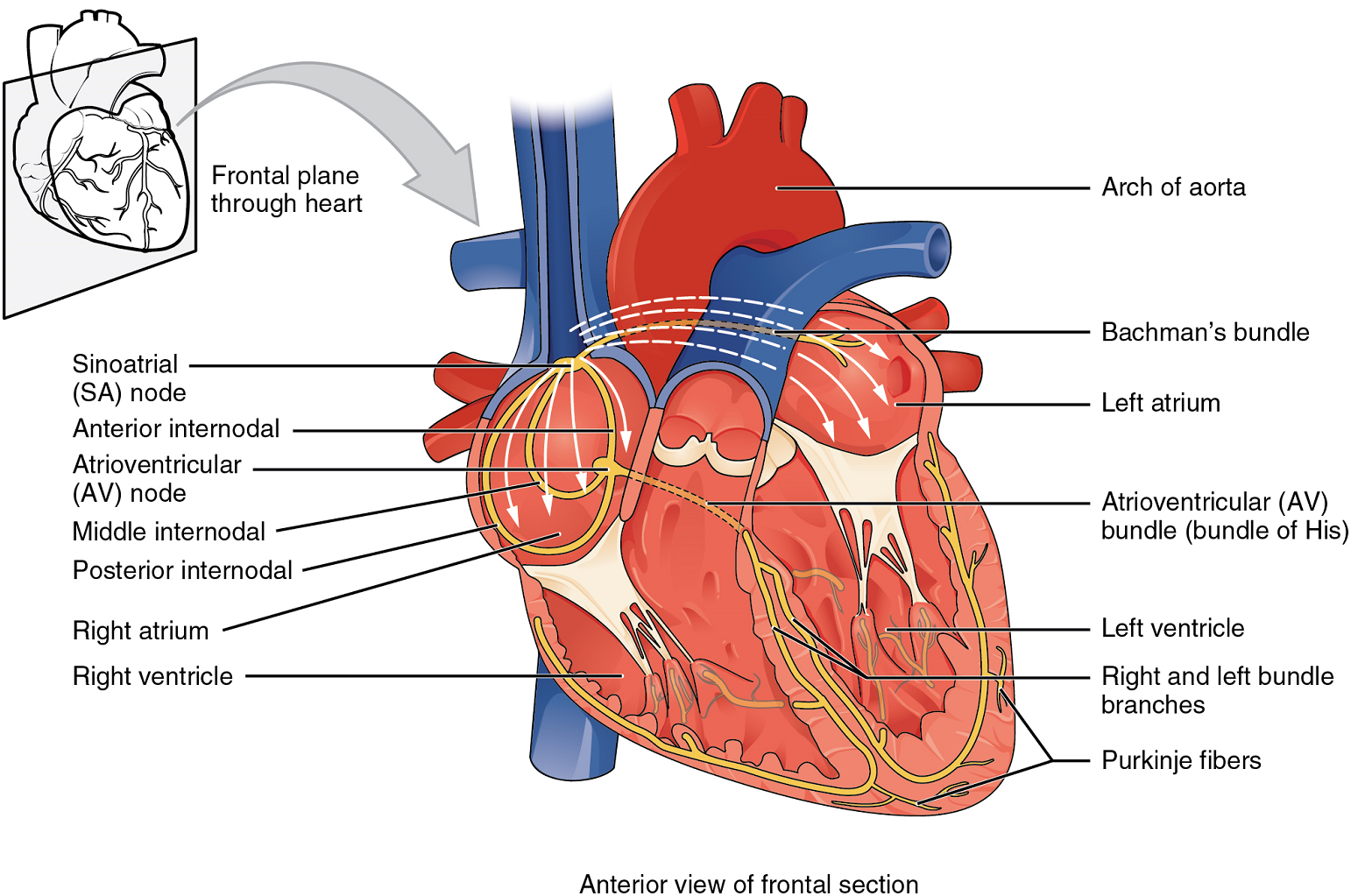
Sinoatrial (SA) Node: Normal cardiac rhythm is established by the sinoatrial (SA) node, a specialized clump of myocardial conducting cells located in the superior and posterior walls of the right atrium in close proximity to the orifice of the superior vena cava. The sinoatrial node has the highest inherent rate of depolarization and is known as the pacemaker of the heart. It initiates the sinus rhythm, or normal electrical pattern followed by contraction of the heart.
This impulse spreads from its initiation in the sinoatrial node throughout the atria to the atrial myocardial contractile cells and the atrioventricular node (Figure 13). The impulse takes approximately 50 ms (milliseconds) to travel between these two nodes. When the impulse reaches the atrioventricular septum, the connective tissue of the cardiac skeleton prevents the impulse from spreading into the myocardial cells in the ventricles except at the atrioventricular node.
The electrical event, the wave of depolarization, is the trigger for muscular contraction. The wave of depolarization begins in the right atrium, and the impulse spreads across the superior portions of both atria and then down through the contractile cells. The contractile cells then begin contraction from the superior to the inferior portions of the atria, efficiently pumping blood into the ventricles.
Atrioventricular (AV) Node: The atrioventricular (AV) node is a second clump of specialized myocardial conductive cells, located in the inferior portion of the right atrium within the atrioventricular septum. The septum prevents the impulse from spreading directly to the ventricles without passing through the atrioventricular node. There is a critical pause before the atrioventricular node depolarizes and transmits the impulse to the atrioventricular bundle (Figure 14).
This delay in transmission is partially attributable to the small diameter of the cells of the node, which slow the impulse. Also, conduction between nodal cells is less efficient than between cardiomyocytes to complete their contraction that pumps blood into the ventricles before the impulse is transmitted to the cells of the ventricle itself. With extreme stimulation by the sinoatrial node, the atrioventricular node can transmit impulses maximally at 220 per minute. This establishes the typical maximum heart rate in a healthy young individual. Damaged hearts or those stimulated by drugs can contract at higher rates, but at these rates, the heart can no longer effectively pump blood. It takes the impulse approximately 100 ms to pass through the atrioventricular node. This pause is critical to heart function, as it allows the atria to empty their blood into the ventricles.
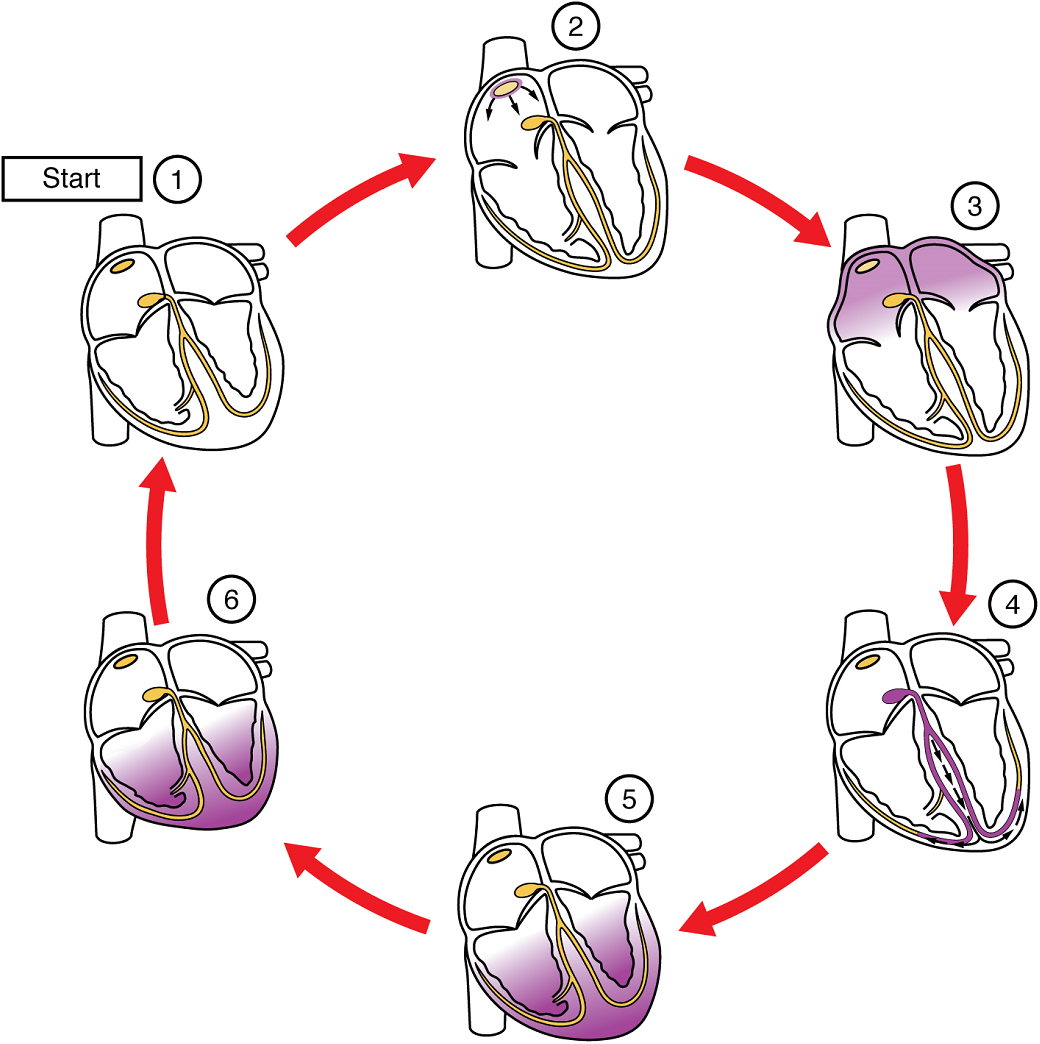
(5) The impulse spreads to the contractile fibres of the ventricle. (6) Ventricular contraction begins.
Atrioventricular Bundle (Bundle of His), Bundle Branches, and Purkinje Fibres: Arising from the atrioventricular node, the atrioventricular bundle, or bundle of His, proceeds through the interventricular septum before dividing into two atrioventricular bundle branches, commonly called the left and right bundle branches. The left bundle branch supplies the left ventricle, and the right bundle branch the right ventricle. Both bundle branches descend and reach the apex of the heart where they connect with the Purkinje fibres (Figure 14). This passage takes approximately 25 ms.
The Purkinje fibres are additional myocardial conductive fibres that spread the impulse to the myocardial contractile cells in the ventricles. They extend throughout the myocardium from the apex of the heart toward the atrioventricular septum and the base of the heart. The Purkinje fibres have a fast inherent conduction rate, and the electrical impulse reaches all of the ventricular muscle cells in about 75 ms (Figure 14). Since the electrical stimulus begins at the apex, the contraction also begins at the apex and travels toward the base of the heart, similar to squeezing a tube of toothpaste from the bottom. However, the contraction of the ventricles is asynchronous with the right ventricle contracting slightly ahead of the left ventricle at the apex. This causes a twisting of the ventricles pushing blood towards major vessels leaving the heart. This allows the blood to be pumped out of the ventricles and into the aorta and pulmonary trunk in a more efficient manner. The total time elapsed from the initiation of the impulse in the sinoatrial node until depolarization of the ventricles is approximately 225 ms.
Electrocardiogram
By careful placement of surface electrodes on the body, it is possible to record the complex, compound electrical signal of the heart. This tracing of the electrical signal is the electrocardiogram (ECG), also commonly abbreviated EKG (K coming from kardiology, from the German term for cardiology). Careful analysis of the ECG reveals a detailed picture of both normal and abnormal heart function, and is an indispensable clinical diagnostic tool. The standard electrocardiograph (the instrument that generates an ECG) uses 3, 5, or 12 leads. The greater the number of leads an electrocardiograph uses, the more information the ECG provides. The term “lead” may be used to refer to the cable from the electrode to the electrical recorder, but it typically describes the voltage difference between two of the electrodes. The 12-lead electrocardiograph uses 10 electrodes placed in standard locations on the patient’s skin (Figure 15).
There are five prominent points on the ECG: the P wave, the QRS complex, and the T wave (Figure 16). The small P wave represents the depolarization of the atria. The atria begin contracting approximately 25 ms after the start of the P wave. The large QRS complex represents the depolarization of the ventricles, which requires a much stronger electrical signal because of the larger size of the ventricular cardiac muscle. The ventricles begin to contract as the QRS reaches the peak of the R wave. Lastly, the T wave represents the repolarization of the ventricles. The repolarization of the atria occurs during the QRS complex, which masks it on an ECG.
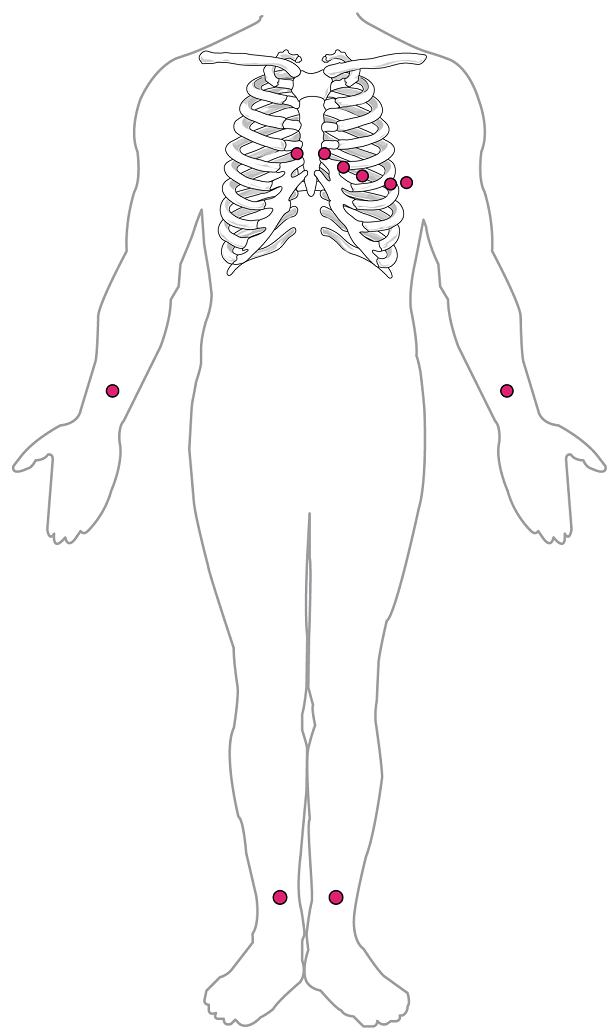
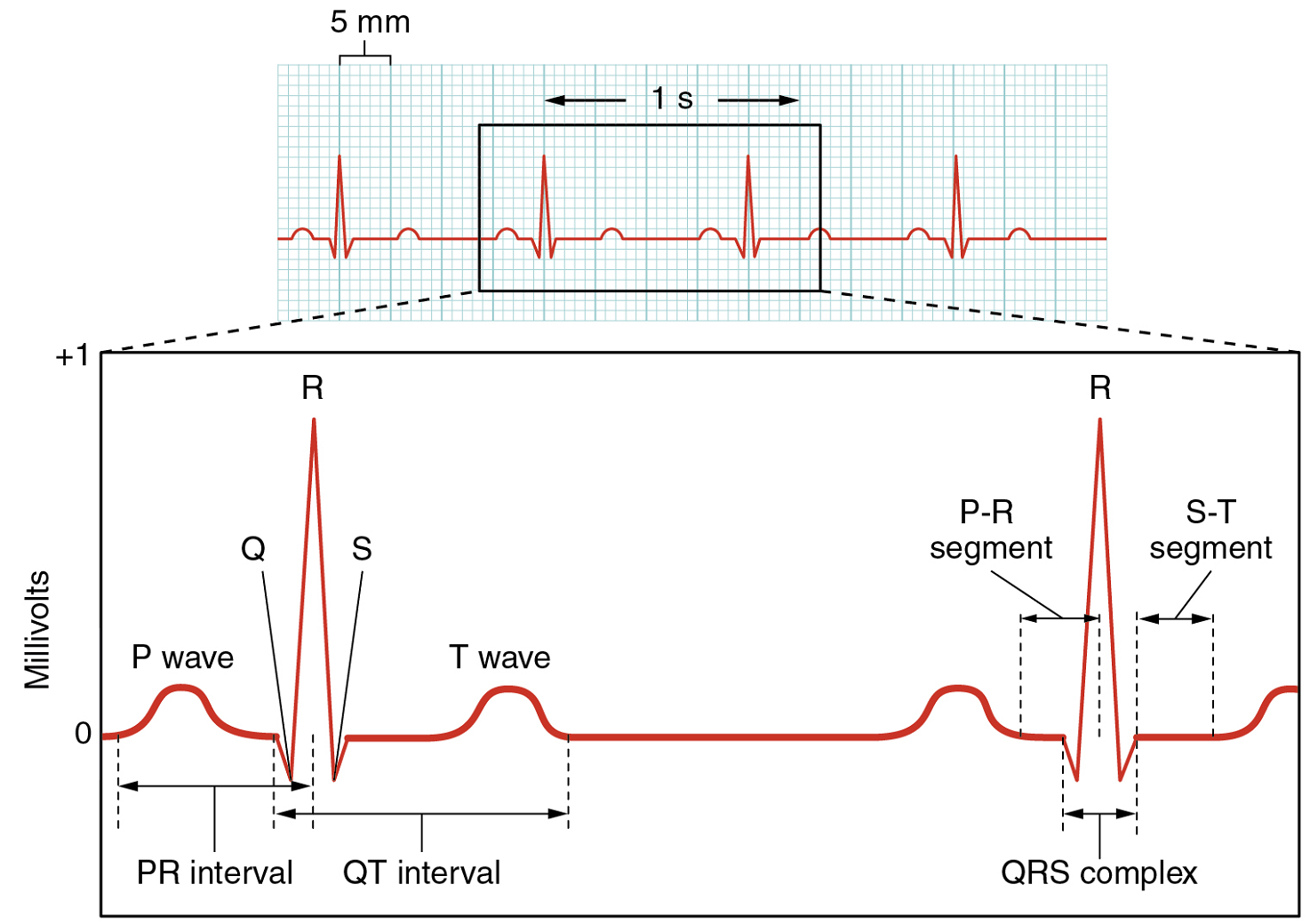
The depolarization events that appear on an ECG tracing should result in contraction of the corresponding chambers (Figure 17). Repolarization events measured then correspond with relaxation of the corresponding chambers.
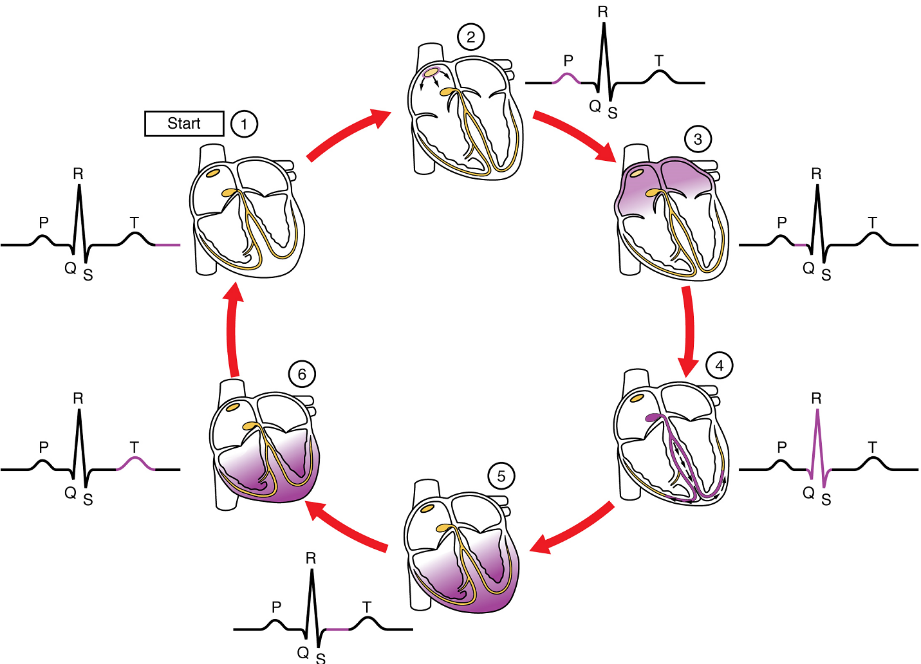
Part 3: Cardiac Cycle
The period of time that begins with contraction of the atria and ends with ventricular relaxation is known as the cardiac cycle (Figure 18). The period of contraction that the heart undergoes while it pumps blood into circulation is called systole. The period of relaxation that occurs as the chambers fill with blood is called diastole. Both the atria and ventricles undergo systole and diastole, and it is essential that these components be carefully regulated and coordinated to ensure blood is pumped efficiently to the body.
Pressures and Flow
Fluids, whether gases or liquids, are materials that flow according to pressure gradients—that is, they move from regions that are higher in pressure to regions that are lower in pressure. Accordingly, when the heart chambers are relaxed (diastole), blood will flow into the atria from the veins, which are higher in pressure. As blood flows into the atria, the pressure will rise, so the blood will initially move passively from the atria into the ventricles. When the action potential triggers the muscles in the atria to contract (atrial systole), the pressure within the atria rises further, pumping blood into the ventricles. During ventricular systole, pressure rises in the ventricles, pumping blood into the pulmonary trunk from the right ventricle and into the aorta from the left ventricle. Again, as you consider this flow and relate it to the conduction pathway, the elegance of the system should become apparent.
At the beginning of the cardiac cycle, both the atria and ventricles are relaxed (diastole). Blood is flowing into the right atrium from the superior and inferior venae cavae and the coronary sinus. Blood flows into the left atrium from the four pulmonary veins. The two atrioventricular valves, the tricuspid and mitral valves, are both open, so blood flows unimpeded from the atria and into the ventricles. Approximately 70–80% of ventricular filling occurs by this method. The two semilunar valves, the pulmonary and aortic valves, are closed, preventing backflow of blood into the right and left ventricles from the pulmonary trunk on the right and the aorta on the left.
Atrial Systole and Diastole: Contraction of the atria follows depolarization, represented by the P wave of the ECG (Figure 25). As the atrial muscles contract from the superior portion of the atria toward the atrioventricular septum, pressure rises within the atria and blood is pumped into the ventricles through the open atrioventricular (tricuspid, and mitral or bicuspid) valves. At the start of atrial systole, the ventricles are normally filled with approximately 70–80% of their capacity due to inflow during diastole. Atrial contraction, also referred to as the “atrial kick,” contributes the remaining 20–30% of filling (Figure 24). Atrial systole lasts approximately 100 ms and ends prior to ventricular systole, as the atrial muscle returns to diastole.
Ventricular Systole: Ventricular systole (Figure 24) follows the depolarization of the ventricles and is represented by the QRS complex in the ECG (Figure 25).
Initially, as the muscles in the ventricle contract, the pressure of the blood within the chamber rises, but it is not yet high enough to open the semilunar (pulmonary and aortic) valves and be ejected from the heart. However, blood pressure quickly rises above that of the atria that are now relaxed and in diastole. This increase in pressure causes blood to flow back toward the atria, closing the tricuspid and mitral valves.
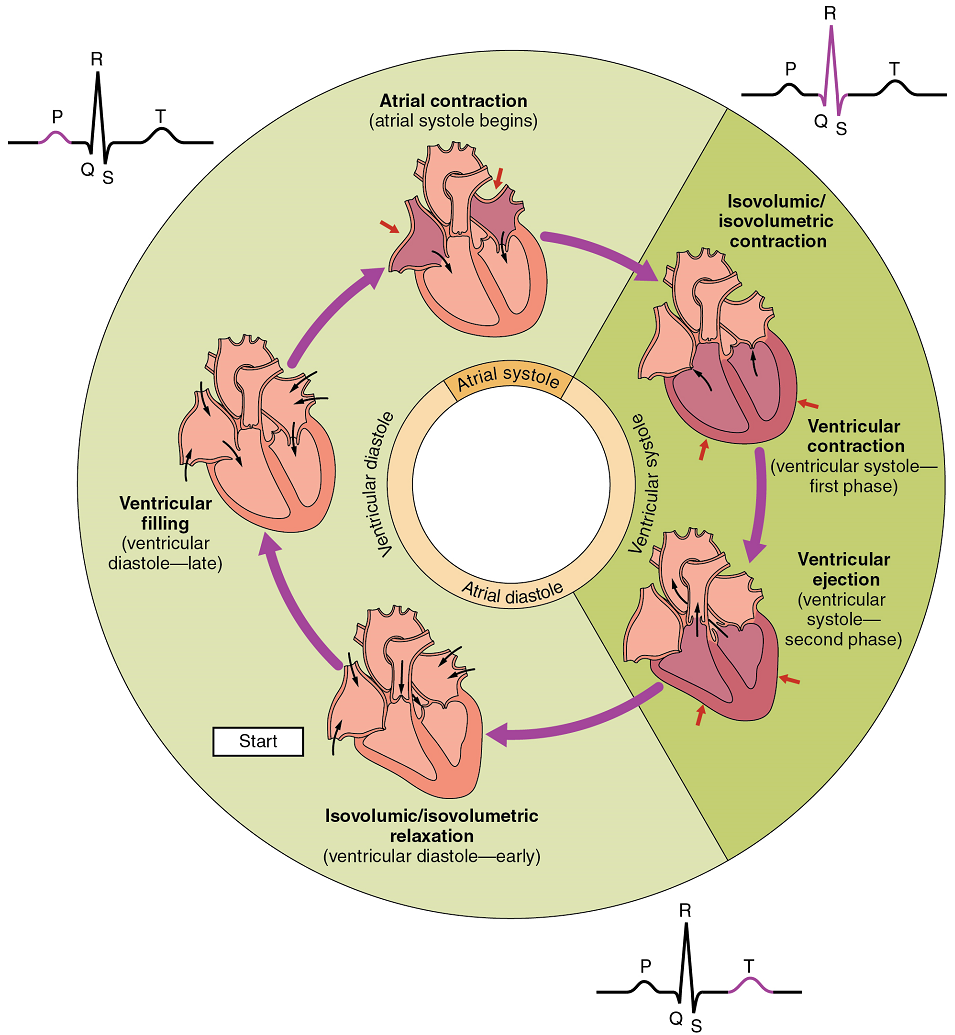
Eventually, the contraction of the ventricular muscle has raised the pressure within the ventricle to the point that it is greater than the pressures in the pulmonary trunk and the aorta. Blood is pumped from the heart, pushing open the pulmonary and aortic semilunar valves. Pressure generated by the left ventricle will be appreciably greater than the pressure generated by the right ventricle, since the existing pressure in the aorta will be so much higher. Nevertheless, both ventricles pump the same amount of blood. This quantity is referred to as stroke volume.
Ventricular Diastole: Ventricular relaxation, or diastole, follows repolarization of the ventricles and is represented by the T wave of the ECG (Figure 19). As the ventricular muscle relaxes, pressure on the remaining blood within the ventricle begins to fall. When pressure within the ventricles drops below pressure in both the pulmonary trunk and aorta, the semilunar valves close to prevent backflow into the heart (Figure 18).
As the ventricular muscle relaxes further, pressure on the blood within the ventricles drops even further. Eventually, it drops below the pressure in the atria. When this occurs, blood flows from the atria into the ventricles, pushing open the tricuspid and mitral valves. As pressure drops within the ventricles, blood flows from the major veins into the relaxed atria and from there into the ventricles. Both chambers are in diastole, the atrioventricular valves are open, and the semilunar valves remain closed (Figure 18). The cardiac cycle is complete.
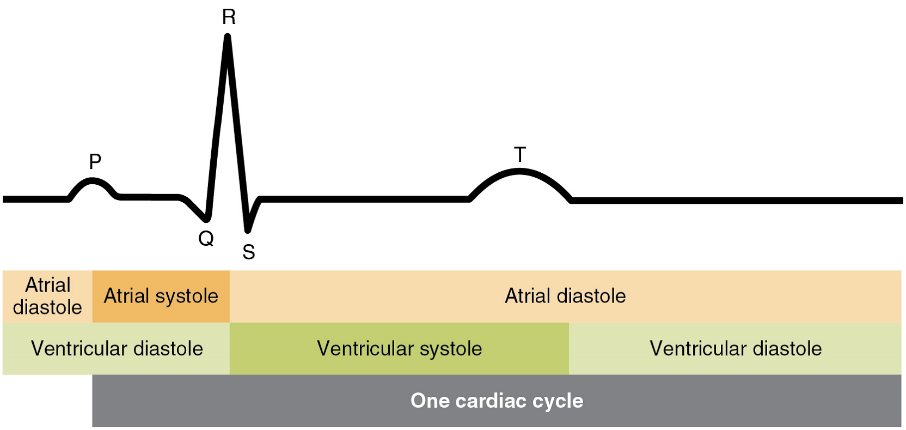
Heart Sounds
One of the simplest, yet effective, diagnostic techniques applied to assess the state of a patient’s heart is auscultation using a stethoscope.
In a normal, healthy heart, there are only two audible heart sounds: S1 and S2. S1 is the sound created by the closing of the atrioventricular valves during ventricular contraction and is normally described as a “lub,” or first heart sound. The second heart sound, S2, is the sound of the closing of the semilunar valves during ventricular diastole and is described as a “dub”). In both cases, as the valves close, the openings within the atrioventricular septum guarded by the valves will become reduced, and blood flow through the opening will become more turbulent until the valves are fully closed.
Part 4: Cardiac Physiology
The autorhythmicity inherent in cardiac cells keeps the heart beating at a regular pace; however, the heart is regulated by and responds to outside influences as well). Neural and endocrine controls are vital to the regulation of cardiac function. In addition, the heart is sensitive to several environmental factors, including electrolytes.
Heart Rates
Heart rates vary considerably, not only with exercise and fitness levels, but also with age. Newborn resting heart rates may be 120 bpm. Heart rate gradually decreases until young adulthood and then gradually increases again with age.
Bradycardia (resting heart rate below 60 bpm) may be caused by either inherent factors or causes external to the heart. While the condition may be inherited, typically it is acquired in older individuals.
Tachycardia (resting heart rate above 100 bpm) is not normal in a resting patient but may be detected in pregnant women or individuals experiencing extreme stress. The normal resting heart rates of children are often above 100 bpm, but this is not considered to be tachycardia.
Cardiovascular Centres
Nervous control over heart rate is centralized within the two paired cardiovascular centres of the medulla oblongata (Figure 20). The cardioacceleratory regions stimulate activity via sympathetic stimulation of the cardioacceleratory nerves, and the cardioinhibitory centres inhibit heart rate via parasympathetic stimulation as one component of the vagus nerve, cranial nerve X.
During rest, both centres provide slight stimulation to the heart, contributing to autonomic tone. This is a similar concept to tone in skeletal muscles. Normally, vagal stimulation predominates; left unregulated, the sinoatrial node would initiate a sinus rhythm of approximately 100 bpm.
The ventricles are more richly innervated by sympathetic fibres than parasympathetic fibres. Sympathetic stimulation causes the release of the neurotransmitter norepinephrine (NE) at the neuromuscular junction of the cardiac nerves. Norepinephrine shortens the repolarization period, thus speeding the rate of depolarization and force of contraction, which results in an increase in heart rate.
Norepinephrine binds mainly to the beta-1 receptors in the heart but there are also beta-2 receptors and norepinephrine’s effect is similar on these. Some cardiac medications (for example, beta blockers) work by blocking these receptors, thereby slowing heart rate and are one possible treatment for hypertension. Overuse of these drugs may lead to bradycardia and even stoppage of the heart with chronic use.
Parasympathetic stimulation originates from the cardioinhibitory region with impulses traveling via the vagus nerve (cranial nerve X). The vagus nerve sends branches to both the sinoatrial and atrioventricular nodes, and to portions of both the atria and ventricles. Parasympathetic stimulation releases the neurotransmitter acetylcholine (ACh) at the neuromuscular junction, and ACh slows heart rate. Without any nervous stimulation, the sinoatrial node would establish a sinus rhythm of approximately 100 bpm. Since resting rates are considerably less than this, it becomes evident that parasympathetic stimulation normally slows heart rate.
Input to the Cardiovascular Centre: The cardiovascular centre receives input from a series of visceral receptors with impulses traveling through visceral sensory fibres within the vagus and sympathetic nerves via the cardiac plexus. Among these receptors are various proprioceptors, baroreceptors, and chemoreceptors, plus stimuli from the limbic system. Collectively, these inputs normally enable the cardiovascular centres to regulate heart function precisely, a process known as cardiac reflexes. Increased physical activity results in increased rates of firing by various proprioreceptors located in muscles, joint capsules, and tendons. Any such increase in physical activity would logically warrant increased blood flow. The cardiac centres monitor these increased rates of firing, and suppress parasympathetic stimulation and increase sympathetic stimulation as needed in order to increase blood flow.
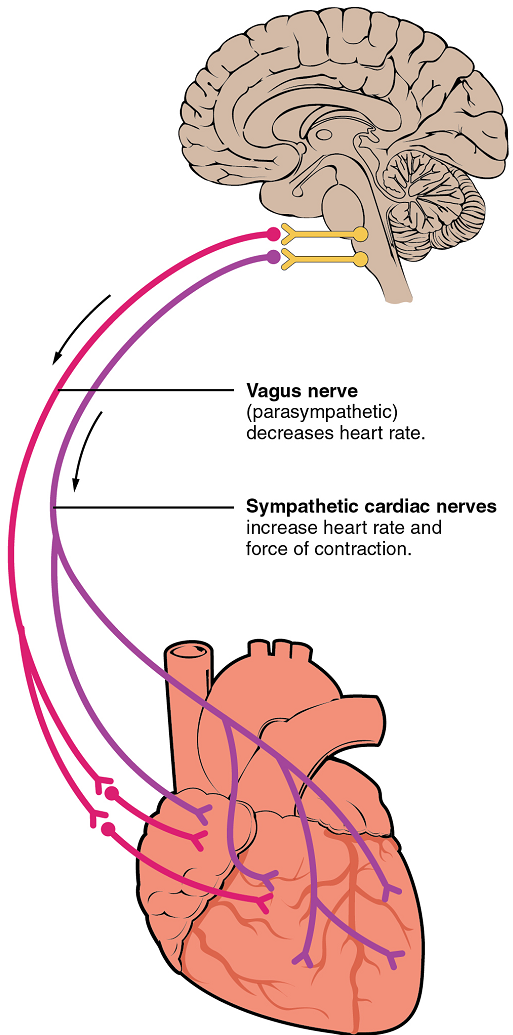
Cardioacceleratory and cardioinhibitory centres of the brain innervate the heart via sympathetic cardiac nerves that increase cardiac activity and part of the vagus (parasympathetic) nerve that slows cardiac activity.
Similarly, baroreceptors are stretch receptors located in the aortic sinus, carotid bodies, the venae cavae, and other locations, including pulmonary vessels and the right side of the heart itself. Rates of firing from the baroreceptors represent blood pressure, level of physical activity, and the relative distribution of blood. The cardiac centres monitor baroreceptor firing to maintain cardiac homeostasis, a mechanism called the baroreceptor reflex. With increased pressure and stretch, the rate of baroreceptor firing increases, and the cardiac centres decrease sympathetic stimulation and increase parasympathetic stimulation. As pressure and stretch decrease, the rate of baroreceptor firing decreases, and the cardiac centres increase sympathetic stimulation and decrease parasympathetic stimulation (Table 1 and Table 2).
Increased metabolic byproducts associated with increased activity, such as carbon dioxide, hydrogen ions, and lactic acid, plus falling oxygen levels, are detected by a suite of chemoreceptors innervated by the glossopharyngeal and vagus nerves. These chemoreceptors provide feedback to the cardiovascular centres about the need for increased or decreased blood flow, based on the relative levels of these substances (Table 1 and Table 2).
Baroreceptors (aorta, carotid arteries, venae cavae, and atria) | Chemoreceptors (both central nervous system and in proximity to baroreceptors) | |
---|---|---|
Sensitive to | Decreasing stretch | Decreasing O2 and increasing CO2, H+, and lactic acid |
Target | Parasympathetic stimulation suppressed | Sympathetic stimulation increased |
Response of heart | Increasing heart rate and increasing stroke volume | Increasing heart rate and increasing stroke volume |
Overall effect | Increasing blood flow and pressure due to increasing cardiac output | Increasing blood flow and pressure due to increasing cardiac output |
Baroreceptors (aorta, carotid arteries, venae cavae, and atria) | Chemoreceptors (both central nervous system and in proximity to baroreceptors) | |
---|---|---|
Sensitive to | Increasing stretch | Increasing O2 and decreasing CO2, H+, and lactic acid |
Target | Parasympathetic stimulation increased | Sympathetic stimulation suppressed |
Response of heart | Decreasing heart rate and decreasing stroke volume | Decreasing heart rate and decreasing stroke volume |
Overall effect | Decreasing blood flow and pressure due to decreasing cardiac output | Decreasing blood flow and pressure due to decreasing cardiac output |
Other Factors Influencing Heart Rate
Using a combination of autorhythmicity and innervation, the cardiovascular centre is able to provide relatively precise control over heart rate. However, there are a number of other factors that have an impact on heart rate as well, including epinephrine, norepinephrine, and thyroid hormones; levels of various ions including calcium, potassium, and sodium; body temperature; hypoxia; and pH (Table 3).
Epinephrine and Norepinephrine: The catecholamines (epinephrine and norepinephrine) secreted by the adrenal medulla form one component of the extended fight-or-flight mechanism. The other component is sympathetic stimulation. Epinephrine and norepinephrine have similar effects. There is no parasympathetic stimulation to the adrenal medulla.
Thyroid Hormones: In general, increased levels of thyroid hormone, or thyroxin, increase both the heart rate and the force of contraction (contractility). The impact of thyroid hormone is typically of a much longer duration than that of the catecholamines.
Calcium: Calcium ion levels have great impacts upon both heart rate and contractility; as the levels of calcium ions increase, so do heart rate and contractility. Extremely high levels of calcium may induce cardiac arrest.
Factor | Effect |
---|---|
Increased sympathetic nervous system activity | Increased heart rate and contractility; norepinephrine release |
Increased parasympathetic nervous system activity | Decreased heart rate |
Epinephrine adrenalin and norepinephrine/noradrenalin | Increased heart rate and contractility |
Thyroxine | Increased heart rate and contractility |
Blood Ca2+ concentration | High levels increase heart rate and contractility; Low levels decrease heart rate and contractility |
Blood K+ concentration | Low levels increase heart rate and contractility; High levels decrease heart rate and contractility |
High body temperature | Increased heart rate |
Low body temperature | Decreased heart rate and contractility |
Part 1: Heart Anatomy
Part 2: Cardiac Muscle and Electrical Activity
Part 3: Cardiac Cycle
Part 4: Cardiac Physiology
A central compartment in the thoracic cavity located intermediate to the left and right pleural cavities.
Cavity surrounding the heart filled with a lubricating serous fluid that reduces friction as the heart contracts.
Flattened bone located at the center of the anterior chest.
Hyaline cartilage structure attached to the anterior end of each rib that provides for either direct or indirect attachment of most ribs to the sternum.
Large systemic vein that returns blood to the heart from the inferior portion of the body.
Parts of the circulatory system involving blood flow to and from the lungs.
Parts of the circulatory system involving blood flow to and from almost all the tissues in the body (other than the pulmonary circuit)
The single large vessel exiting the right ventricle that divides to form the right and left pulmonary arteries.
A wall or divider. In heart, specifically: the wall or partitions that divides the heart into chambers (plural= septa).
Oval-shaped depression in the interatrial septum that marks the former location of the foramen ovale.
Opening in the fetal heart that allows blood to flow directly from the right atrium to the left atrium, bypassing the fetal pulmonary circuit.
One-way valves located between the atria and ventricles; the valve on the right is called the tricuspid valve, and the one on the left is the mitral or bicuspid valve.
Reinforced connective tissue located within the atrioventricular septum; includes four rings that surround the openings between the atria and ventricles, and the openings to the pulmonary trunk and aorta; the point of attachment for the heart valves.
Large, thin-walled vein on the posterior surface of the heart that lies within the atrioventricular sulcus and drains the heart myocardium directly into the right atrium.
String-like extensions of tough connective tissue that extend from the flaps of the atrioventricular valves to the papillary muscles.
Extension of the myocardium in the ventricles to which the chordae tendineae attach.
(Also, left atrioventricular valve or bicuspid valve) valve located between the left atrium and ventricle; consists of two flaps of tissue.
Valves located at the base of the pulmonary trunk and at the base of the aorta.
Layer of smooth, simple squamous epithelium that lines the endocardium and blood vessels.
Branches of the ascending aorta that supply blood to the heart; the left coronary artery feeds the left side of the heart, the left atrium and ventricle, and the interventricular septum; the right coronary artery feeds the right atrium, portions of both ventricles, and the heart conduction system.
Sulcus (groove) that marks the boundary between the atria and ventricles
Ability of cardiac muscle to initiate its own electrical impulse that triggers the mechanical contraction that pumps blood at a fixed pace without nervous or endocrine control.
Specialized myocardial conduction fibres that arise from the bundle branches and spread the impulse to the myocardial contraction fibers of the ventricles.
Known as the pacemaker, a specialized clump of myocardial conducting cells located in the superior portion of the right atrium that has the highest inherent rate of depolarization that then spreads throughout the heart.
Clump of myocardial cells located in the inferior portion of the right atrium within the atrioventricular septum; receives the impulse from the SA node, pauses, and then transmits it into specialized conducting cells within the interventricular septum.
(also, bundle of His) group of specialized myocardial conductile cells that transmit the impulse from the AV node through the interventricular septum; form the left and right atrioventricular bundle branches
(Also, left or right bundle branches) specialized myocardial conductile cells that arise from the bifurcation of the atrioventricular bundle and pass through the interventricular septum; lead to the Purkinje fibers and also to the right papillary muscle via the moderator band.
Large systemic vein that returns blood to the heart from the superior portion of the body.
Change in a cell membrane potential from rest toward or above zero (as during an action potential).
Normal contractile pattern of the heart.
Surface recording of the electrical activity of the heart that can be used for diagnosis of irregular heart function; also abbreviated as EKG.
Component of the electrocardiogram that represents the depolarization of the atria.
Component of the electrocardiogram that represents the depolarization of the ventricles and includes, as a component, the repolarization of the atria.
Component of the electrocardiogram that represents the repolarization of the ventricles.
Period of time between the onset of atrial contraction (atrial systole) and ventricular relaxation (ventricular diastole).
Period of time when the heart muscle is contracting.
Period of time when the heart muscle is relaxed and the chambers fill with blood.
Sounds heard with a stethoscope of the closing of the atrioventricular valves (“lub”) and semilunar valves (“dub”).
Abnormally low heart rate (<60 beats per minute).
Abnormally high heart rate (>100 beats per minute).
Tenth cranial nerve; responsible for the autonomic control of organs in the thoracic and upper abdominal cavities.
Contractile state during resting cardiac activity produced by mild sympathetic and parasympathetic stimulation.
Branch of the autonomic nervous system associated with emergency systems ("fight of flight").
Branch of the autonomic nervous system associated with resting systems ("rest and digest").
Secondary catecholamine hormone secreted by the adrenal medulla in response to short-term stress; also called noradrenaline.
Synapse between the axon terminal of a motor neuron and the section of the membrane of a muscle fiber with receptors for the acetylcholine released by the terminal.
Return of the membrane potential to its normally negative voltage at the end of the action potential.
Sensory receptors providing information about location and movement of body parts; the “sense of the self”.