1.5 Three Big Ideas: Geological Time, Uniformitarianism, and Plate Tectonics
In geology there are three big ideas that are fundamental to the way we think about how Earth works. The ideas are like the sound track to a movie—sometimes we might not even notice them, but they nevertheless affect our perception of what’s happening. In the rest of this book these ideas may be mentioned explicitly in some cases, but in other cases they won’t be discussed by name, and it will be helpful for you to realize that they’re relevant.
Geological Time (Deep Time)
Earth is approximately 4.6 billion years old (4,600,000,000 years), which is a long time for geological events to unfold and changes to happen. The changes themselves might be tiny—over a year, a chemical reaction might eat away a few layers of atoms at the surface of a rock. Over hundreds of millions of years, however, the chemical reaction could cause a mountain range to crumble into grains of sand, and be swept away by rivers.
For geologists who study very, very slow processes, 10 million years might be a short time, and 1 million years might be trivial. For these geologists, intervals of 1 million years aren’t even useful to consider, because the changes over that time are too small to see in the rocks that accumulated.
As you read through this book, keep in mind that the well of geologic time is indeed deep, and “ancient” is defined in a whole new way.
Need Some Help Visualizing Geological Time?
Watch the animation Four Ways to Understand the Earth’s Age to see four analogies for geological time.
Expressing Geological Time in Numbers
Special notation is used for geological time because, as you might imagine, writing all those zeroes can become tiresome. Table 1.1 shows common abbreviations you will see throughout this book.
Table 1.1 Abbreviations Used to Describe Geological Time | ||
---|---|---|
Abbreviation | Meaning | Example |
Ga | giga annum or billions of years |
Earth is 4.6 Ga old. |
Ma | mega annum or millions of years |
Earth is 4,600 Ma old. |
ka | kilo annum or thousands of years | The last glacial cycle ended 11,700 years ago, or 11.7 ka. |
How Many Years Is That?
Expressing Geological Time Using the Geological Time Scale
The geological time scale (Figure 1.7) is a way of breaking down geological time according to important events in Earth’s history. Time is divided into eons, eras, periods, and epochs, and these intervals are referred to by names rather than by years. Giving intervals of geologic time names rather than using numbers makes sense because we won’t always know the age in years (the absolute age) of a rock or fossil, but we can place it in context based on our knowledge of the geological record. We can describe its relative age by saying that it’s older than or younger than another rock or fossil.
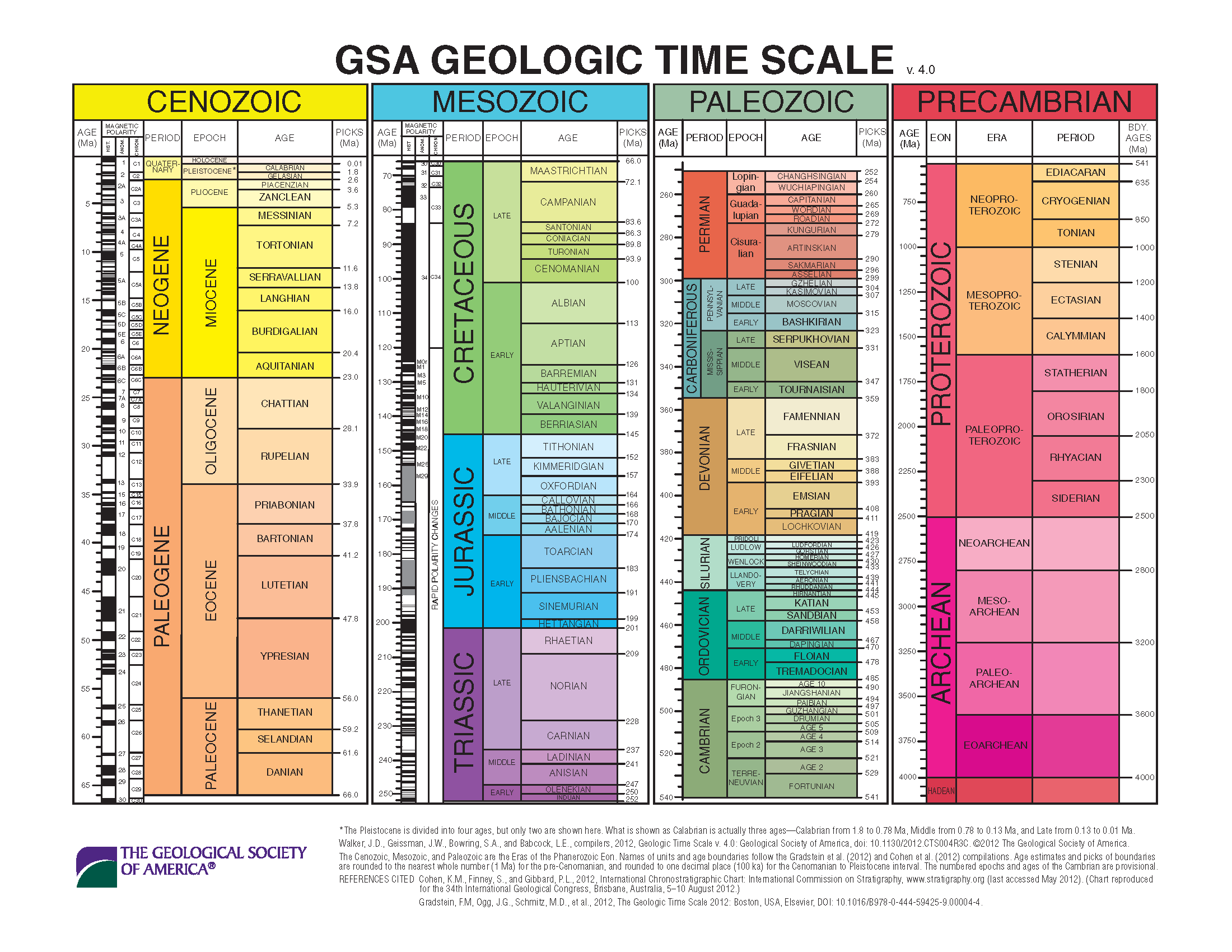
The tricky thing about the geologic time scale is that the boundaries are always changing. As our knowledge of the absolute age of an event improves with new discoveries, it might be necessary to nudge a boundary earlier or later. Sometimes the original reason for defining a boundary no longer holds, but we agree to use it anyway. For example, the Phanerozoic Eon (the last 542 million years) is named for the time during which visible (phaneros) life (zoi) is present in the geological record, and its start was meant to mark the first appearance of these organisms. We now have evidence that large organisms—those that leave fossils visible to the naked eye—have existed longer than that, first appearing by 600 Ma at the latest.
An Early Definition of the Proterozoic
Notice that in Figure 1.7 the Proterozoic Eon precedes the Phanerozoic Eon. This was not always the case. Figure 1.8 shows an excerpt from a periodical published in 1879, in which the Proterozoic is defined as covering the Cambrian through Silurian. The author refers to “the most extreme adherents of the Murchisonian party in geology,” a reference to the contentious assertion by Scottish geologist Roderick Murchison (1792-1871) that the Silurian Period should encompass the Cambrian and Ordovician periods as well.
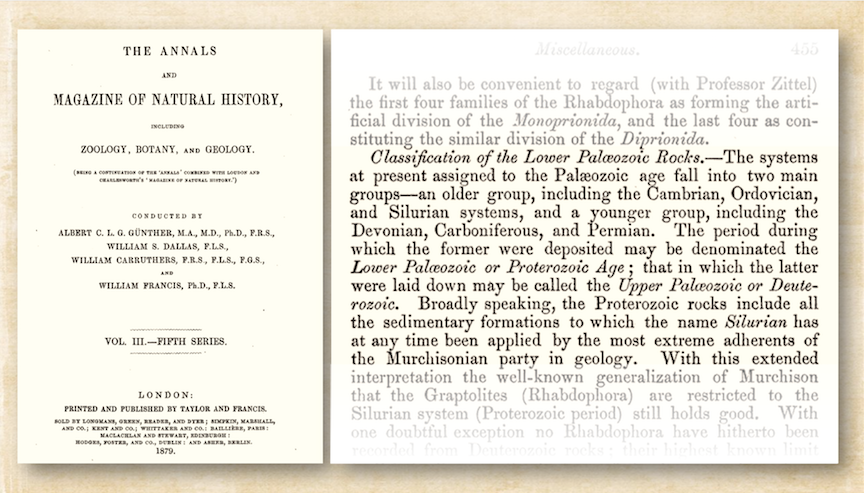
A Way To Think About Geological Time
A useful mechanism for understanding geological time is to scale it down into one year. The origin of the solar system and Earth at 4.6 Ga would be represented by January 1, and the present year would be represented by the last tiny fraction of a second on New Year’s Eve. At this scale, each day of the year represents 12.5 million years; each hour represents about 500,000 years; each minute represents 8,694 years; and each second represents 145 years. Some significant events in Earth’s history, as expressed on this time scale, are summarized in Table 1.2.
Table 1.2 Some Important Dates Expressed As If All of Geological Time Were Condensed Into One Year | ||
---|---|---|
Event | Approximate Date | Calendar Equivalent |
Formation of oceans and continents | 4.5 – 4.4 Ga | first week of January |
Evolution of the first primitive life forms | 3.8 Ga | end of February |
Formation of British Columbia’s oldest rocks | 2.0 Ga | July |
Evolution of the first multi-celled animals | 600 Ma | beginning of November |
Animals first crawled onto land | 360 Ma | end of November |
Vancouver Island reached North America and the Rocky Mountains were formed | 90 Ma | December 16 |
Extinction of the non-avian dinosaurs | 66 Ma | December 18 |
The last time atmospheric CO2 levels were above 400 ppm (Tripati et al., 2009) | 16 Ma | 6:20 p.m. December 30 |
Beginning of the Pleistocene ice age | 2 Ma | 10:10 p.m., December 31 |
Oldest radiocarbon date from people living in Canada (British Columbia) | 13.8 ka | 11:58 p.m., December 31 |
First indication of fossil fuel impacts on atmospheric CO2 levels (~280 ppm) | 221 years ago | 1.5 seconds before midnight, December 31 |
Atmospheric CO2 levels exceed 400 ppm | 8 years ago | 0.06 seconds before midnight, December 31 |
Source: Karla Panchuk (2021) CC BY 4.0, modified after Steven Earle (2015) CC BY 4.0 view original |
Uniformitarianism
Uniformitarianism is the notion that the geological processes occurring on Earth today are the same ones that occurred in the past. This is an important idea because it means that observations we make today about geological processes can be used to interpret and understand the rock record. While this idea might not seem remarkable today, it was ground breaking and even controversial for its time. Many people who heard about it for the first time thought about the age of the Earth in thousands of years, but uniformitarianism required them to think on timescales almost too vast to comprehend. For some, this implied questioning their most deeply held religious beliefs.
The idea of uniformitarianism can be traced back to James Hutton, first in a paper he read to the Royal Society in 1784, and later in his book Theory of the Earth, first published in 1788. (Read this book at Project Gutenberg.) In 1788 he explained, “In examining things present, we have data from which to reason with regard to what has been; and, from what has actually been, we have data for concluding with regard to that which is to happen hereafter.”
Charles Lyell, also a Scottish geologist, expanded on Hutton’s ideas and incorporated those of may other early geologists in his own book Principles of Geology. According to Lyell, “religious prejudice” was a major stumbling block to the acceptance of these ideas, but also Hutton and other scientists should have written less wordy books, and included more pictures.
Charles Lyell’s thinking about uniformitarianism is paraphrased as “the present is the key to the past.” As he put it in his own less-wordy more-pictures book, a belief in the “permanency of the laws of nature” means that a geologist
“will deem it incumbent on him to examine with minute attention all the changes now in progress on the Earth, and will regard every fact collected respecting the causes in diurnal action, as affording him a key to the interpretation of some mystery in the archives of remote ages.”
In other words, learning how things work today will tell you about how they worked in the past.
Paraphrasing uniformitarianism as “the present is the key to the past” has led some to view it as an oversimplification, because not all geological processes occurring today occurred at all times in the geological past. Some important chemical reactions that happen on Earth’s surface today require abundant oxygen in the atmosphere, and could not have occurred prior to Earth developing an oxygen-rich atmosphere. Furthermore, there was a time in Earth’s history when continents as we know them hadn’t yet developed. Some events, such as devastating impacts by objects from space, have never been witnessed on the same scale by humans. We must be cognizant of the fact that conditions were different at different times in Earth’s history, and take that into account when interpreting the rock record.
Despite the different past conditions on Earth as a whole, there still exist environments today where some of these conditions are present. These environments are like tiny samples of what Earth used to be like. This means we can still use present conditions to inform us about the past, but we have to think carefully about ways that such environments today differ from the ancient environments that no longer exist.
Arguably, saying that “the present is the key to the past” is an oversimplification on Lyell’s part isn’t exactly fair to Lyell, given his phrasing about the “permanency of the laws of nature.” (Author’s note: I have been unable to find that exact “key to the past” quote so far, so at this point we have to allow for the possibility that Lyell didn’t oversimplify at all, and he’s getting in trouble for what someone else said. I will update this if I find out differently). If we take his meaning to be that the basic way that physics and chemistry work has always been the same, then we are still using that principle to interpret the Mars rocks in Figure 1.2. Imagine what Lyell would have thought about this idea being used to infer the presence of an ancient river on Mars!
Check Your Understanding
Plate Tectonics
The theory of plate tectonics—the idea that Earth’s surface is broken into large moving fragments, called plates—profoundly changed our perspective on how the Earth works. Before plate tectonic theory, we couldn’t answer questions like “How did that mountain range get there?” and “Why do earthquakes happen where they do?” This critical framework is also very recent—the papers that led to the widespread acceptance of plate tectonics were published at about the same time that we got cordless power tools, contact lenses, and satellite television.
Earth has 15 large tectonic plates (Figure 1.9), and numerous smaller ones. (A more detailed map of Earth’s tectonic plates can be found at here.) Tectonic plates move in a variety of directions and at a variety of speeds, although on average they move a few millimetres per year. In Figure 1.9, the black arrows show the direction of motion. The size of arrows indicates how fast the plate is moving. Plates with arrows going in more than one direction are rotating. Plate tectonic traffic is complicated!
The explanatory power of plate tectonic theory comes from what happens when plates interact along their margins. Plate boundary interactions (red arrows) include collisions (→ ←), separation (← →), or sliding along each other (↗ ↙).
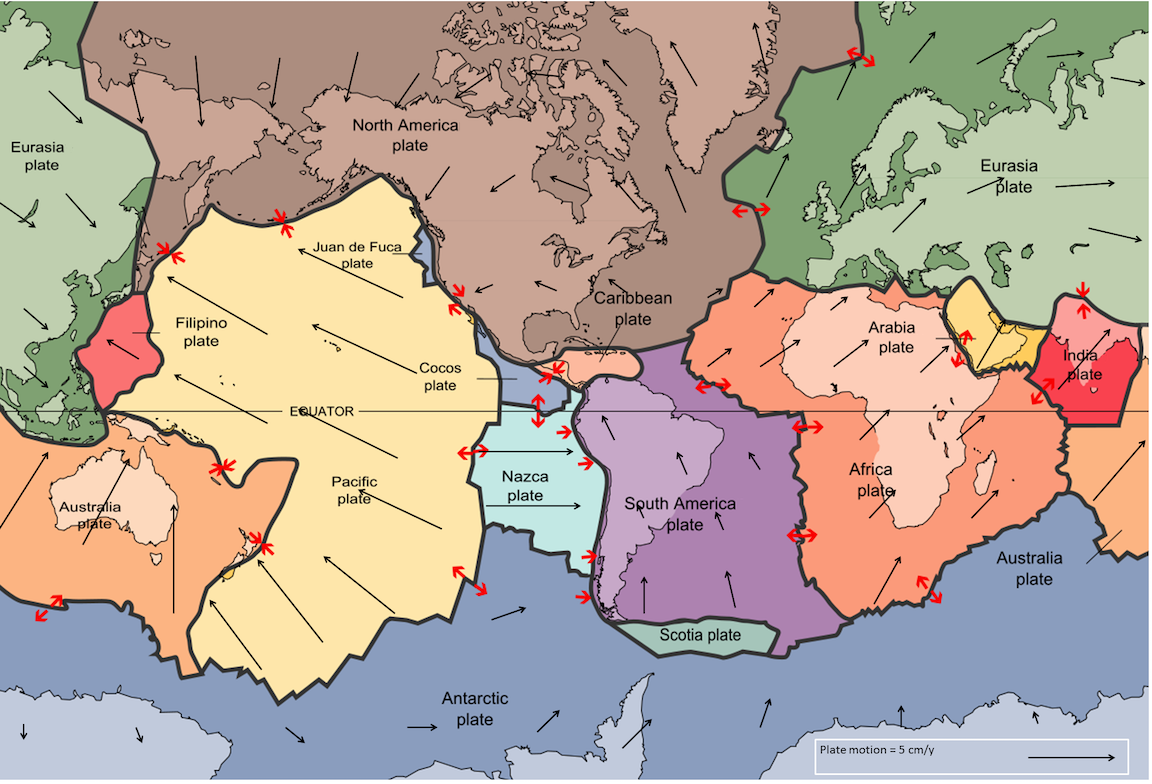
Before plate tectonic theory, we made observations but could only guess at mechanisms. It was like watching the hands on a clock and trying to guess what moves them. After plate tectonics it was like being able to open the clock and not only watch the gears turn, but realize for the first time that there are such things as gears. Plate tectonics not only explains why things have happened, but also allows us to predict what might happen in the future.
Plate tectonics is covered in more detail later, however the key point is that Earth’s outer layer consists of rigid plates that are constantly interacting with each other as they move around the Earth. The plates can move because they are floating on a layer of weak rock that deforms as the plates travel, much the same way the filling in a peanut butter and jelly sandwich allows you to slide the top layer of bread across the bottom layer.
Whether the plates move away from each other, collide, or just slide past each other determines things like the locations of mountain belts and volcanoes, where earthquakes happen, and the shapes and sizes of oceans and continents.
Check Your Understanding