14.5 Flooding
Why Floods Happen
Floods occur when water overwhelms a landscape because more water is supplied than can be contained within a stream channel, or than can be removed by infiltration into the ground. Floods can be caused by excessive rain, rapid snowmelt, or breaching of structures such as dams or levees.
Some floods develop over the course of days, and can takes weeks to abate. In western Canada, this is the case with seasonal floods in the spring, when there is too much snowmelt for channels to carry away, and still-frozen ground prevents water from soaking in. Rapid melt and frozen ground were factors that led to extreme flooding in Manitoba in 2009 (Figure 14.27). The problem was compounded by ice jams, in which chunks of river ice piled up and blocked the channel, as well as by rain and snow storms.
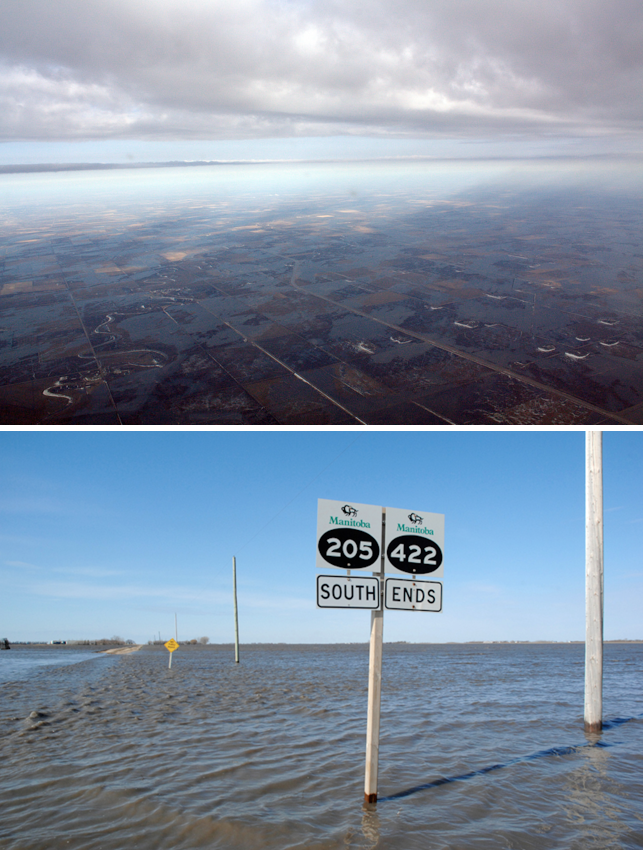
Flash floods are very rapid floods. The onset of a flash flood may take only minutes, and flooding may last only a few hours. Flash floods can result from failures of structures such as dams or levees. Another cause is heavy rainfall in terrain where drainage occurs very rapidly, or in urban areas where the storm drain system can’t handle the runoff.
Predicting Floods
Measuring Streams
Measuring streams is an important part of understanding how a particular stream behaves, and monitoring it for flooding. Throughout the US and Canada, major streams are fitted with stream gauges to measure the stream’s height. The stream gauges make measurements automatically at regular intervals, and data are transmitted via telephone lines, cellular signals, or satellite to data processing centres.
Stream gauges measure a stream’s stage, the height of the water surface measured relative to a pre-determined baseline. Bankfull stage is the elevation of the water surface when the water is the same height as the bank. Flood stage is the elevation of the water surface at which people and property are at risk. The flood stage of a stream may not be the same as its bankfull stage. For example, a community could have regulations that prevent people from building structures on lower ground near the stream. In that case, the stream could rise above its bank and still not pose a problem for people or buildings.
The crest of a flood is the highest elevation that the water will reach during a flooding event.
The height of a stream might seem like the most pertinent information to know during a flood, particularly if you are personally faced with rising waters. However, the information that is needed for flood prediction is not the height of a stream, but its discharge. Discharge is the volume of water that moves past a point in a given amount of time, expressed as m3/s. Stream gauges don’t measure discharge, so scientists must measure the stream flow directly when the stream is at different stages. They must also measure the channel itself to make a volume calculation. By calculating discharge at known stages, they are able to determine a relationship between the stream’s stage, and its discharge.
information about discharge can be combined with information about precipitation to determine the lag time of a stream. The lag time is the time between the peak of a precipitation event and the peak discharge rate after that event. In other words, it indicates how long it takes runoff to get to a stream. The longer the lag time, the lower the risk of flooding because there is more time to disperse the runoff. The lag time is determined by plotting precipitation and discharge in a hydrograph (Figure 14.28).
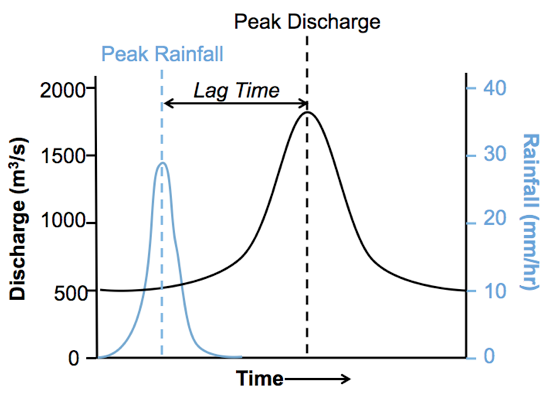
Stream Discharge is Highly Variable
The discharge levels of streams vary considerably depending on the time of year and on variations in the weather from one year to the next. In Canada, most streams show discharge variability similar to that of the Stikine River in northwestern BC, illustrated in Figure 14.29. The Stikine River has its lowest discharge levels in the depths of winter when freezing conditions persist throughout most of its drainage basin. Discharge starts to rise slowly in May, and then rises dramatically through the late spring and early summer as the winter snow melts. For the year shown, the minimum discharge of the Stikine River was 56 m3/s in March, and the maximum was 37 times higher at 2,470 m3/s in May.
![Figure 13.24 Variations in discharge of the Stikine River during 2013. [SE from data at Water Survey of Canada, Environment Canada, http://www.ec.gc.ca/rhc-wsc/]](https://pressbooks.bccampus.ca/knowinghome/wp-content/uploads/sites/1304/2017/11/Stikine-River.png)
Streams in coastal areas of southern British Columbia show a very different pattern from those in most of the rest of the country. In this region, the drainage basins receive a lot of rain (rather than snow) during the winter and also do not remain entirely frozen throughout the winter. The Qualicum River on Vancouver Island typically has its highest discharge levels in January or February and its lowest levels in late summer (Figure 14.30). In 2013, the minimum discharge of the Qualicum River was 1.6 m3/s in August, and the maximum was 34 times higher at 53 m3/s in March.
![Figure 13.25 Variations in discharge of the Qualicum River during 2013. [SE from data at Water Survey of Canada, Environment Canada, http://www.ec.gc.ca/rhc-wsc/]](https://pressbooks.bccampus.ca/knowinghome/wp-content/uploads/sites/1304/2021/03/Qualicum-River.png)
When a stream’s discharge increases, both the water level (stage) and the velocity increase as well. Rapidly flowing streams become muddy, and large volumes of sediment are transported both in suspension and along the stream bed. In extreme situations, the water level reaches the top of the stream’s banks (the bank-full stage), and if it rises further, it will overflow the banks and floods the surrounding terrain.
In the case of mature or old-age streams, the surrounding terrain could include a vast area of relatively flat ground known as a flood plain, which is the area that is typically covered with water during a major flood. Since fine river sediments are deposited on flood plains, they are ideally suited for agriculture, and thus are typically occupied by farms and residences, and in many cases, by towns or cities. Such infrastructure is highly vulnerable to damage from flooding, and the people that live and work there are at risk.
Discharge Variability and Flooding of Streams in Canada
Most streams in Canada have the greatest risk of flooding in the late spring and early summer when stream discharges rise in response to melting snow. In some cases, this is exacerbated by spring storms. In years when melting is especially fast and/or spring storms are particularly intense, flooding can be very severe.
One of the worst floods in Canadian history took place in the Fraser Valley of BC in late May and early June of 1948. The early spring of that year had been cold, and a large snow pack in the interior was slow to melt. In mid-May, temperatures rose quickly and melting was accelerated by rainfall. Fraser River discharge levels rose rapidly over several days during late May, and the dykes built to protect the valley were breached in a dozen places. Approximately one-third of the flood plain was inundated, and many homes and other buildings were destroyed, but there were no deaths.
The Fraser River flood of 1948, which was the worst flood in the Fraser Valley in the past century, was followed by very high river levels in 1950 and 1972, and by relatively high levels several times since then, the most recent being 2007 (Table 14.1). In the years following 1948, millions of dollars were spent repairing and raising the existing dykes and building new ones. Since then damage from flooding in the Fraser Valley has been relatively limited.
Table 14.1 Ranking of the maximum stage and discharge values for the Fraser River at Hope between 1948 and 2008. Typical discharge levels are ~1,000 m3/s.
Source: Data from Mannerstrom (2008) Comprehensive Review of Fraser River at Hope Flood Hydrology and Flows Scoping Study, Report prepared for the B.C. Ministry of the Environment. View source. |
|||||
Rank | Year | Month | Date | Stage (m) | Discharge (m3/s) |
1 | 1948 | May | 31 | 11.0 | 15,200 |
2 | 1972 | Jun | 16 | 10.1 | 12,900 |
3 | 1950 | Jun | 20 | 9.9 | 12,500 |
4 | 1964 | Jun | 21 | 9.6 | 11,600 |
5 | 1997 | Jun | 5 | 9.5 | 11,300 |
6 | 1955 | Jun | 29 | 9.4 | 11,300 |
7 | 1999 | Jun | 22 | 9.4 | 11,000 |
8 | 2007 | Jun | 10 | 9.3 | 10,850 |
9 | 1974 | Jun | 22 | 9.3 | 10,800 |
10 | 2002 | Jun | 21 | 9.2 | 10,600 |
Serious flooding occurred in July 1996 in the Saguenay-Lac St. Jean region of Quebec. In this case, the floods were caused by two weeks of heavy rainfall followed by one day of exceptional rainfall. On July 19, 1996 there was 270 mm of rain, equivalent to the region’s normal rainfall for the entire month of July. Ten deaths were attributed to the Saguenay floods, and the economic toll was estimated at $1.5 billion.
Just a year after the Saguenay floods, the Red River in Minnesota, North Dakota, and Manitoba reached its highest level since 1826. As is typical for the Red River, the 1997 flooding was due to rapid snowmelt. Due to the south to north flow of the river, the flooding starts in Minnesota and North Dakota, where melting begins earlier, then extends northwards. The residents of Manitoba had plenty of warning that the 1997 flood was coming because there was severe flooding at several locations on the U.S. side of the border.
After the 1950 Red River flood, the Manitoba government built a channel around the city of Winnipeg to reduce the potential of flooding in the city (Figure 14.31). Known as the Red River Floodway, the channel was completed in 1964 at a cost of $63 million. Since then it has been used many times to alleviate flooding in Winnipeg, and is estimated to have saved many billions of dollars in flood damage. The massive 1997 flood (Figure 14.31, right side) was almost too much for the floodway; in fact the amount of water diverted was greater than the designed capacity. The floodway has recently been expanded so that it can be used to divert even more of the Red River’s flow away from Winnipeg.
![Figure 13.26 Map of the Red river Floodway around Winnipeg, Manitoba (left), and aerial view of the southern (inlet) end of the floodway (right). [Map from http://en.wikipedia.org/wiki/1997_Red_River_Flood#/media/File:Rednorthfloodwaymap.png and photo from Natural Resources Canada 2012, courtesy of the Geological Survey of Canada (Photo 2000-118 by G.R. Brooks).]](https://pressbooks.bccampus.ca/knowinghome/wp-content/uploads/sites/1304/2021/03/Red-river-Floodway.png)
Canada’s most costly flood ever was the June 2013 flood in southern Alberta. The flooding was initiated by snowmelt and worsened by heavy rains in the Rockies due to an anomalous flow of moist air from the Pacific and the Caribbean. At Canmore, AB rainfall amounts exceeded 200 mm in 36 hours, and at High River, AB 325 mm of rain fell in 48 hours.
![Figure 13.27 Map of the communities most affected by the 2013 Alberta floods (in orange) [SE]](https://pressbooks.bccampus.ca/knowinghome/wp-content/uploads/sites/1304/2021/03/Alberta-floods.png)
In late June and early July, the discharges of several rivers in the area, including the Bow River in Banff, Canmore, and Exshaw, the Bow and Elbow Rivers in Calgary, the Sheep River in Okotoks, and the Highwood River in High River, reached levels that were 5 to 10 times higher than normal for that time of year. Large areas of Calgary, Okotoks, and High River were flooded, and five people died (see Figures 14.30 and 14.31). The cost of the 2013 flood is estimated to be approximately $5 billion.
![Figure 13.28 Flooding in Calgary (June 21, left) and Okotoks (June 20, right) during the 2013 southern Alberta flood [http://upload.wikimedia.org/wikipedia/commons/6/6a/Riverfront_Ave_Calgary_Flood_2013.jpg http://upload.wikimedia.org/wikipedia/en/9/9b/Okotoks_-_June_20%2C_2013_-_Flood_waters_in_local_campground_playground-03.JPG]](https://pressbooks.bccampus.ca/knowinghome/wp-content/uploads/sites/1304/2021/03/Flooding-in-Calgary.png)
One of the things that the 2013 flood of the Bow River teaches us is that we cannot predict when a flood will occur nor how big it will be, so in order to minimize damage and casualties we need to be prepared. Some ways of preparing include:
- Mapping flood plains and not building within them
- Building dykes or dams where necessary
- Monitoring the winter snowpack, the weather, and stream discharges
- Creating emergency plans
- Educating the public on how to prepare for and respond to the threat of flooding
Flood Frequency
Have you ever heard of a major flood referred to as a 100-year flood, or maybe even a 500-year flood? What does that actually mean?
When a flood is referred to as a 500-year flood, this means that in the known history of the stream, the discharge that caused the flood occurred once every 500 years, on average. The recurrence interval of a flood of that magnitude is 500 years. A recurrence interval of 500 years is the same as saying that every year, there is a 1 in 500 chance (0.2%) that a flood of that size will happen. That will be true regardless of whether a 500-year flood occurred the year before. In other words, if a 500-year flood occurred one year, a flood of the same magnitude could still happen the following year.
Recurrence intervals are determined by noting how often a stream has a particular discharge rate. Having many years of data for a stream is important to establish the behaviour of the stream. However, basing predictions on the past history of the stream means assuming that the conditions that determine the discharge of the stream have not changed. For example, in the Canadian prairies it was once common practice to drain wetlands so the land could be used for farming. Wetlands can store additional water during snowmelt or heavy rains. Over time as wetlands became fewer, it meant that under the same conditions, more water entered streams than in the past, resulting in higher discharge more often than in the past. In that case, historical data would underestimate the frequency of higher discharge events.
Urbanization also changes the conditions that affect discharge. The concrete and asphalt surfaces common in most towns and cities do not permit water to flow through them and into the ground. Storm drain systems rapidly route storm water to streams. This means water gets to the stream much faster than if it had to move as groundwater. As a result, where in the past discharge would have increased slowly some time after the precipitation event, and be spread out over time, runoff now hits streams rapidly and focused over a shorter period of time.