Hormonal Regulation
Unit 1: The Endocrine System
Unit Outline
Part 1: General properties of the endocrine system
- Endocrine vs Exocrine glands
- General functions of hormones
- Hormone secretion: regulation and stimuli
- Types of hormones
- Types of receptors
- Endocrine vs Nervous systems
Part 2: Major endocrine organs and their secretions
- Hypothalamus and pituitary glands
- Thyroid gland
- Parathyroid gland
- Adrenal cortex and adrenal medulla
- Pancreas
- Ovaries and testes
- Stomach and duodenum
- Thymus and pineal gland
- The special nature of prostaglandins
Learning Objectives
At the end of this unit, you should be able to:
I. Define “gland”.
II. Distinguish between endocrine glands and exocrine glands.
III. Describe the purpose and regulation of hormone secretion.
IV. Describe stimuli for hormone secretion.
V. Describe the main categories of hormones, and how this relates to their receptors and signaling pathways.
VI. Compare and contrast the nervous and endocrine systems.
VII. Identify on a diagram of the human body the locations of important endocrine glands.
VIII. Describe the hypothalamus and pituitary glands and their interrelationship.
IX. Describe the function and secretion of hormones released by the pituitary gland.
X. Describe the function and secretion of hormones released by the thyroid gland.
XI. Describe the function and secretion of hormones released by the parathyroid glands.
XII. Describe the function and secretion of hormones released by the adrenal gland.
XIII. Describe the function and secretion of hormones released by the pancreas.
XIV. Name the hormones produced by the following glands and describe their actions: ovaries, testes, stomach, duodenum, thymus and pineal gland.
XV. Describe prostaglandins, referring to their composition, where they are produced, where they generally have an effect, and four effects.
Learning Objectives and Guiding Questions
At the end of this unit, you should be able to complete all the following tasks, including answering the guiding questions associated with each task.
I. Define “gland”.
II. Distinguish between endocrine glands and exocrine glands.
- Describe the different means by which exocrine and endocrine glands release their secretions.
- Identify the general difference between the types of secretions that these two types of glands secrete and name two examples of secretions from each type of gland.
- Name two organs in the body that have both exocrine and endocrine functions. Identify the exocrine and endocrine secretions of one of these organs.
III. Describe the purpose and regulation of hormone secretion.
- Specify the fundamental function of the endocrine system (include the definition of homeostasis).
- Describe six overall functions of hormones.
- Describe an example of a hormonally based positive feedback mechanism, justifying why this example can be considered as one of positive feedback.
- Name and describe the more common method of hormone regulation. Identify the end result of this method.
IV. Describe stimuli for hormone secretion.
- Explain what is meant by humoral stimulation of hormonal release.
- Describe three examples of hormones that have humoral stimuli.
- Name the hormone, the organ that releases the hormone and the compound which is controlled by the hormone.
- Identify whether these are positive or negative feedback mechanisms.
- Describe one example of a hormone that is controlled by levels of other hormone(s).
- Name the hormone and the organ that releases it.
- Name the organs that release hormones that control the release of this hormone
- Describe how the levels of the first hormone and those of the controlling hormones are related to each other
- Identify whether this is a positive or negative feedback mechanism.
V. Describe the main categories of hormones, and how this relates to their receptors and signaling pathways.
- Explain the basis upon which hormones are divided into two major groups.
- Name and describe the three types of hormones.
- Identify from which compounds each type is derived.
- Name two examples of each type and actions of each example.
- Identify which type of hormone has the longest half-life and explain the reason for this difference.
- Explain why, although they circulate throughout the body, hormones are able to target specific cells.
- Name five responses that may occur when a hormone successfully interacts with a cell.
- Distinguish between intracellular and extracellular receptors
- For each type of receptor, identify the location (inside or on the cell membrane), and the type of hormone with which they interact (i.e., whether lipid or amino acid based).
- Explain why the type of receptor used by a particular hormone is related to the hydrophilic nature of the hormone.
- Give two examples of hormones that interact with each of the two types of receptor.
- Distinguish between the general mechanism that occurs after hormones interact with each of the two types of receptors.
VI. Compare and contrast the nervous and endocrine systems.
- Identify the type of intercellular communication each system uses.
- Describe the anatomical relationship between the sending and receiving cells in each system.
- Identify which system has the more rapid and specific method of message transmission and explain the reason for this.
- Differentiate between the general purposes of the two systems (i.e., which type of body function is mainly governed by each type).
VII. Identify on a diagram of the human body the locations of each of the following glands (or parts of glands):
- Pineal gland
- Thymus
- Hypothalamus
- Adrenal glands
- Adrenal cortex
- Adrenal medulla
- Anterior pituitary
- Posterior pituitary
- Pancreatic islets
- Thyroid
- Ovaries
- Testes
- Parathyroid glands
VIII. Describe the hypothalamus and pituitary glands and their interrelationship.
- Justify the basis for labelling the hypothalamus-pituitary complex as the “command center” of the endocrine system.
- Describe (or draw) the location of the hypothalamus and the anterior pituitary gland, and the anatomical connection between the two glands, including the nature of the vascular connection.
- Describe how a signal is sent from the hypothalamus to the anterior pituitary, to either inhibit or stimulate the release of an anterior pituitary hormone.
- Name and describe the functions of six hypothalamic hormones that control the secretions of the anterior pituitary.
- Describe (or draw) the location of the hypothalamus and the posterior pituitary gland, and the anatomical connection between the two glands, including the nature of the neural connection.
- Name the two hypothalamic hormones that are stored in and secreted from the posterior pituitary.
IX. Describe the function and secretion of hormones released by the pituitary gland.
- Explain what is meant by four of the anterior pituitary hormones being referred to as “tropic” hormones.
- Name and describe the functions of the two anterior pituitary hormones that do not control the secretion of other endocrine glands.
- Describe how the levels of these hormones are controlled.
- Name and describe the conditions caused by hypo- and hypersecretion of growth hormone in childhood and adulthood.
- Name and describe the actions of the four tropic hormones released by the anterior pituitary.
- Describe where the hormones that the posterior pituitary secretes are actually produced, and how they are transported to the posterior pituitary.
- Name and describe the actions of the two hormones released by the posterior pituitary.
- Describe how the levels of these hormones are controlled.
- Name and describe the condition caused by hyposecretion of antidiuretic hormone.
- Differentiate between the conditions of diabetes insipidus and diabetes mellitus.
X. Describe the function and secretion of hormones released by the thyroid gland.
- Describe the location of the thyroid gland.
- Name the two hormones released by the thyroid gland.
- Describe the stimulus for, and control of, the release of thyroid hormone.
- Name the two compounds that are grouped under the term “thyroid hormone”.
- Name and define the bodily process that is increased by the release of thyroid hormone.
- State four other processes for which thyroid hormone is required.
- Name and describe the conditions caused by over and undersecretion of thyroid hormone in childhood and adulthood.
- Describe a goiter and explain how this can develop from over- and understimulation of the thyroid gland.
- Identify the cause for “simple goiter”.
- Describe the stimulus for, control and action of, calcitonin. Identify the type of feedback mechanism involved.
XI. Describe the function and secretion of hormones released by the parathyroid glands.
- Describe the location of the parathyroid glands.
- Name and describe the actions of the hormone released by the parathyroid gland.
- Describe the stimulus for and control of the release of this hormone. Identify the type of feedback mechanism involved.
- Name and describe the conditions caused by over and undersecretion of parathyroid hormone.
XII. Describe the function and secretion of hormones released by the adrenal gland.
- Describe the location and the two general divisions of the adrenal gland.
- Name the three general classes of hormones produced by the adrenal cortex.
- Identify the major mineralocorticoid and describe the stimuli for its release, and the effects of its action.
- Identify the major glucocorticoid and describe the stimuli for its release, and the effects of its action.
- Name and describe the actions and stimulus of the third group of hormones released by the adrenal cortex.
- Name and describe the actions of the two hormones released by the adrenal medulla.
- Name two physical and two psychological stressors.
- Name and describe the three stages of the general adaptation syndrome. For each stage, identify the major hormone involved, its effects and either the purpose of the stage or its end result.
- Describe Addison’s disease: a cause, the effect on cortisol secretion and some effects.
- Describe Cushing’s syndrome: a cause, the effect on cortisol secretion and the resulting effect on fat distribution and blood glucose and sodium levels.
XIII. Describe the function and secretion of hormones released by the pancreas.
- Describe the location of the pancreas.
- Explain the exocrine and endocrine nature of the pancreas, including the name of the clusters of cells, and individual cell types that produce insulin and glucagon.
- Define gluconeogenesis, glycogenolysis and glycogenesis.
- Describe the stimulus for the release of, and three actions of, glucagon. Identify the type of feedback mechanism involved.
- Describe the stimulus for the release of, and five actions of, insulin. Identify the type of feedback mechanism involved.
- Name and describe the condition caused by undersecretion or ineffectiveness of insulin.
- Name another endocrine disorder with a very similar name that is caused by undersecretion of another hormone. Name that hormone.
- Name and describe the actions of the counterregulatory hormones (include glucagon).
XIV. Name the hormones produced by the following glands and describe their actions: ovaries, testes, stomach, duodenum, thymus and pineal gland.
XV. Describe prostaglandins, referring to their composition, where they are produced, where they generally have an effect, and four effects.
Part 1. General Properties of the Endocrine System
Introduction: The two general categories of glands in the body
The term ‘gland’ refers to any organ that produces a secretion. These secretions are produced by specialized cells in the glands from various components in the blood. There are two general categories of glands in the body: exocrine glands and endocrine glands.
Exocrine glands are very diverse and include the salivary glands, mammary glands, sweat glands, pancreas, stomach, prostate, and several others. Their secretions are also varied – saliva, milk, sweat, digestive enzymes and fluids to accompany gametes – just from the glands mentioned above. These glands are called exocrine glands because they have tubes or ducts to carry their secretions from the gland to another part of the body. These ducts may be simple tubes or complex, tree-like groups of ducts. Because of these tubes, the exocrine glands are also known as the ducted glands.
On the other hand, endocrine glands do not have ducts. Their secretions, called hormones, are carried to various body tissues by the blood and lymph, where they bind to receptors on target cells, inducing a characteristic response. Endocrine glands are sometimes called the ductless glands, and they all produce substances similar in nature, in that they are all hormones.
Some organs in the body contain both endocrine tissue and exocrine tissue. These organs include the pancreas, stomach and small intestine, all of which produce both hormones and digestive enzymes. The exocrine function of the pancreas (i.e., secretion of digestive enzymes into the duodenum) will be studied during the section on digestion. The endocrine function of the pancreas (release of the hormones insulin and glucagon, both of which are important in the control of blood sugar levels) will be studied later in this chapter.
General functions of endocrine hormones
You may never have thought of it this way, but when you send a text message to two friends to meet you at local cafe at six, you’re sending digital signals that (you hope) will affect their behavior—even though they are some distance away. Similarly, endocrine glands send chemical signals (hormones) to other cells in the body that influence their behavior. This long-distance intercellular communication, coordination, and control is critical for homeostasis, and it is the fundamental function of the endocrine system.
Although each has its own specific effects, hormones generally have the following functions:
- Some hormones stimulate exocrine glands to produce their secretions
- Some stimulate other endocrine glands to action
- Some affect the growth, development and personality of an individual
- Some regulate body chemistry such as the metabolism of cells
- Some regulate the contraction of muscle tissues and nervous stimulation
- Some control reproductive processes
Regulation of hormone secretion
Homeostasis is the condition in which the body’s internal environment remains relatively constant within limits. One of the main functions of the endocrine system is to aid in the maintenance of homeostasis. To prevent abnormal hormone levels and a potential disease state, hormone levels must be tightly controlled. The body maintains this control by balancing hormone production and degradation. Feedback mechanisms govern the initiation and maintenance of most hormone secretion in response to various stimuli.
The concept of homeostasis and the mechanisms of feedback mechanisms were presented in Unit 8 of the Biology 1103/1109 textbook (for review, refer to: https://pressbooks.bccampus.ca/dcbiol110311092nded/chapter/unit-8-homeostasis/). Recall that there are two types of feedback mechanisms: positive and negative. Positive feedback mechanisms intensify a change in the body’s physiological condition rather than reversing it and result in a definite end event. An example of a hormonally based positive feedback mechanism involves the release of oxytocin during childbirth. The initial release of oxytocin begins to signal the uterine muscles to contract, which pushes the fetus toward the cervix, causing it to stretch. This, in turn, signals the pituitary gland to release more oxytocin, causing labor contractions to intensify. This will bring about the final event of childbirth, after which the release of oxytocin decreases.
However, the more common method of hormone regulation is the negative feedback mechanism, which generally is involved in the continual maintenance of a characteristic within limits. Hormonally based negative feedback mechanisms are characterized by the inhibition of further secretion of a hormone in response to adequate levels of that hormone (as determined by the amount of the hormone in the blood, or by the extent of the effect that the hormone has had). This allows blood levels of the hormone to be regulated within a narrow range.
Stimuli for hormonal secretion
The stimulus for the levels of a particular hormone can be humoral, i.e., blood levels of non-hormone chemicals such as nutrients or ions. Changes in such levels can cause the release or inhibition of a hormone (under negative feedback control) to maintain homeostasis. For example, osmoreceptors in the hypothalamus detect changes in blood osmolarity (the concentration of solutes in the blood plasma) and will signal the hypothalamus to release greater or lesser amounts of antidiuretic hormone (ADH) to keep the levels of solutes in the blood within normal limits. The control of blood glucose levels by the pancreas is another example of such stimulation. Responding directly to the level of glucose in the blood, cells in the pancreas release appropriate amounts of the hormones insulin and glucagon to maintain normal blood glucose levels. A final example of response to the level of a nutrient or ion in the blood is the regulation of levels of calcium by the parathyroid gland, which responds to changes in calcium levels in the blood with the secretion of varying levels of parathyroid hormone. All these mechanisms will be covered in greater detail later in this chapter.
The stimulus for the secretion of a hormone may also be the presence of another hormone produced by a different endocrine gland. Such hormonal stimuli often involve the hypothalamus, which produces releasing and inhibiting hormones that control the secretion of a variety of pituitary hormones, that in turn, may affect other endocrine glands in the body. These secretions are also controlled through negative feedback mechanisms. An example of such a negative feedback mechanism is the release of glucocorticoid hormones from the adrenal glands, as directed by the hypothalamus and pituitary gland (this will also be covered in more detail later in this chapter). As the secretion of glucocorticoid from the adrenal glands cause concentrations of this hormone in the blood to rise, the hypothalamus and pituitary gland reduce their release of hormones that caused this secretion, thus signaling to the adrenal glands to decrease glucocorticoid secretion (Figure 1).
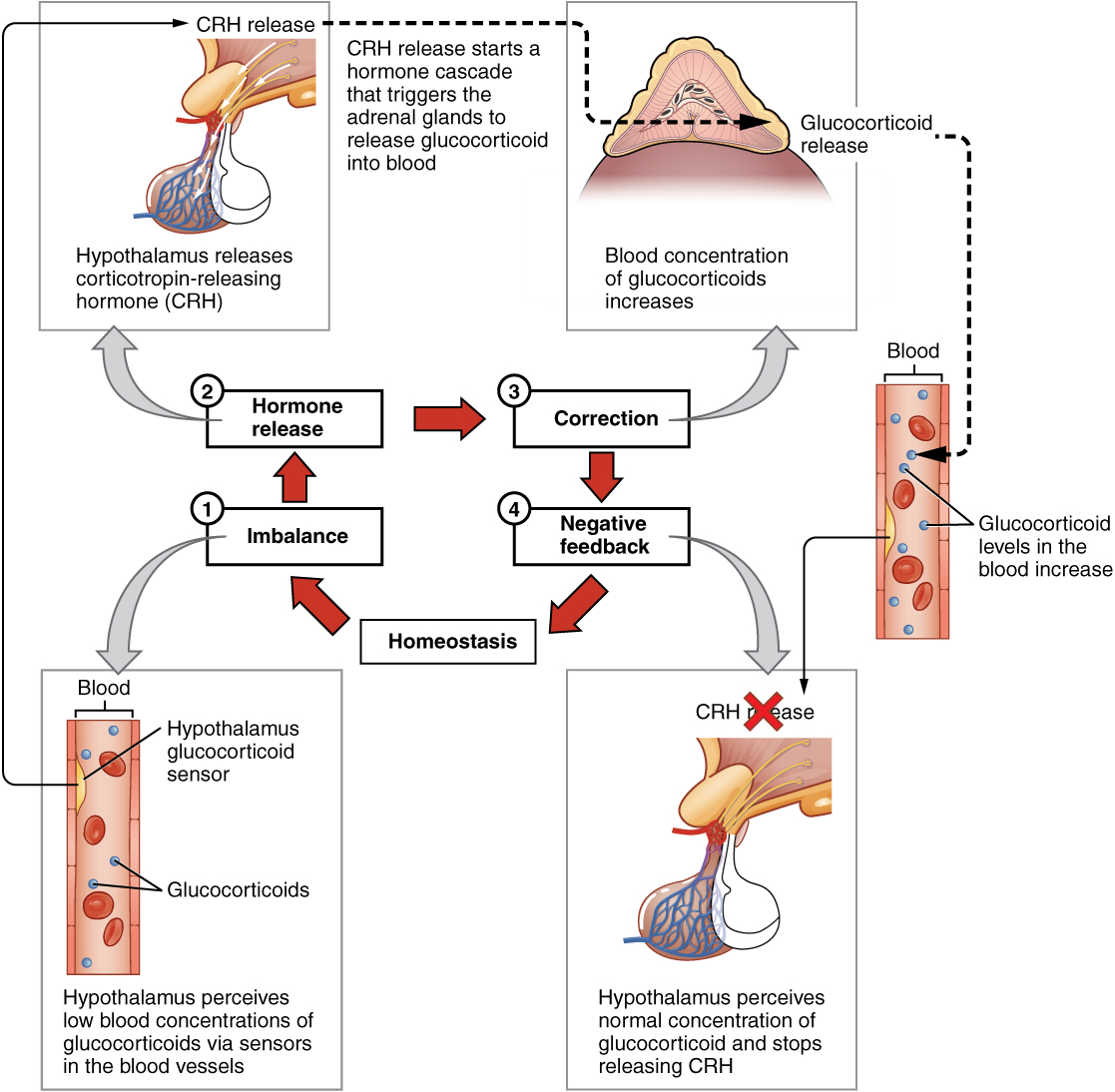
Types of Hormones
The hormones of the human body can be divided into two major groups on the basis of their chemical structure. Hormones derived from amino acids include amines, peptides, and proteins. Those derived from lipids include steroids (Table 1). These chemical groups affect a hormone’s distribution, the type of receptors it binds to, and other aspects of its function.
Amine Hormones
Hormones derived from the modification of amino acids are referred to as amine hormones. Examples these include the metabolism-regulating thyroid hormones, as well epinephrine and norepinephrine, which play a role in the fight-or-flight response.
Peptide and Protein Hormones
Whereas the amine hormones are derived from a single amino acid, peptide and protein hormones consist of multiple amino acids that link to form an amino acid chain. Peptide hormones consist of short chains of amino acids, whereas protein hormones are longer polypeptides.
An example of a peptide hormone is antidiuretic hormone (ADH), a pituitary hormone important in fluid balance. Examples of protein hormones include growth hormone, which is produced by the pituitary gland, and follicle-stimulating hormone (FSH), which helps stimulate the maturation of eggs in the ovaries and sperm in the testes.
Steroid Hormones
The primary hormones derived from lipids are steroids. Steroid hormones are derived from the lipid cholesterol. For example, the reproductive hormones testosterone and the estrogens—which are produced by the gonads (testes and ovaries)—are steroid hormones. The adrenal glands produce the steroid hormone aldosterone, which is involved in osmoregulation, and cortisol, which plays a role in metabolism.
Like cholesterol, steroid hormones are not soluble in water (they are hydrophobic). Because blood is water-based, lipid-derived hormones must travel to their target cell bound to a transport protein. This more complex structure extends the half-life of steroid hormones to much longer than that of hormones derived from amino acids. A hormone’s half-life is the time required for half the concentration of the hormone to be degraded. For example, the lipid-derived hormone cortisol has a half-life of approximately 60 to 90 minutes. In contrast, the amino acid–derived hormone epinephrine has a half-life of approximately one minute.
Pathways of Hormone Action
Although a given hormone may travel throughout the body in the bloodstream, it will affect the activity only of its target cells; that is, cells with receptors for that particular hormone. The message a hormone sends is received by a hormone receptor, a protein located either inside the cell or within the cell membrane. The receptor will process the message by initiating other signaling events or cellular mechanisms that result in the target cell’s response. Hormone receptors recognize molecules with specific shapes and side groups, and respond only to those hormones that are recognized. The same type of receptor may be located on cells in different body tissues, and trigger somewhat different responses. Thus, the response triggered by a hormone depends not only on the hormone, but also on the target cell.
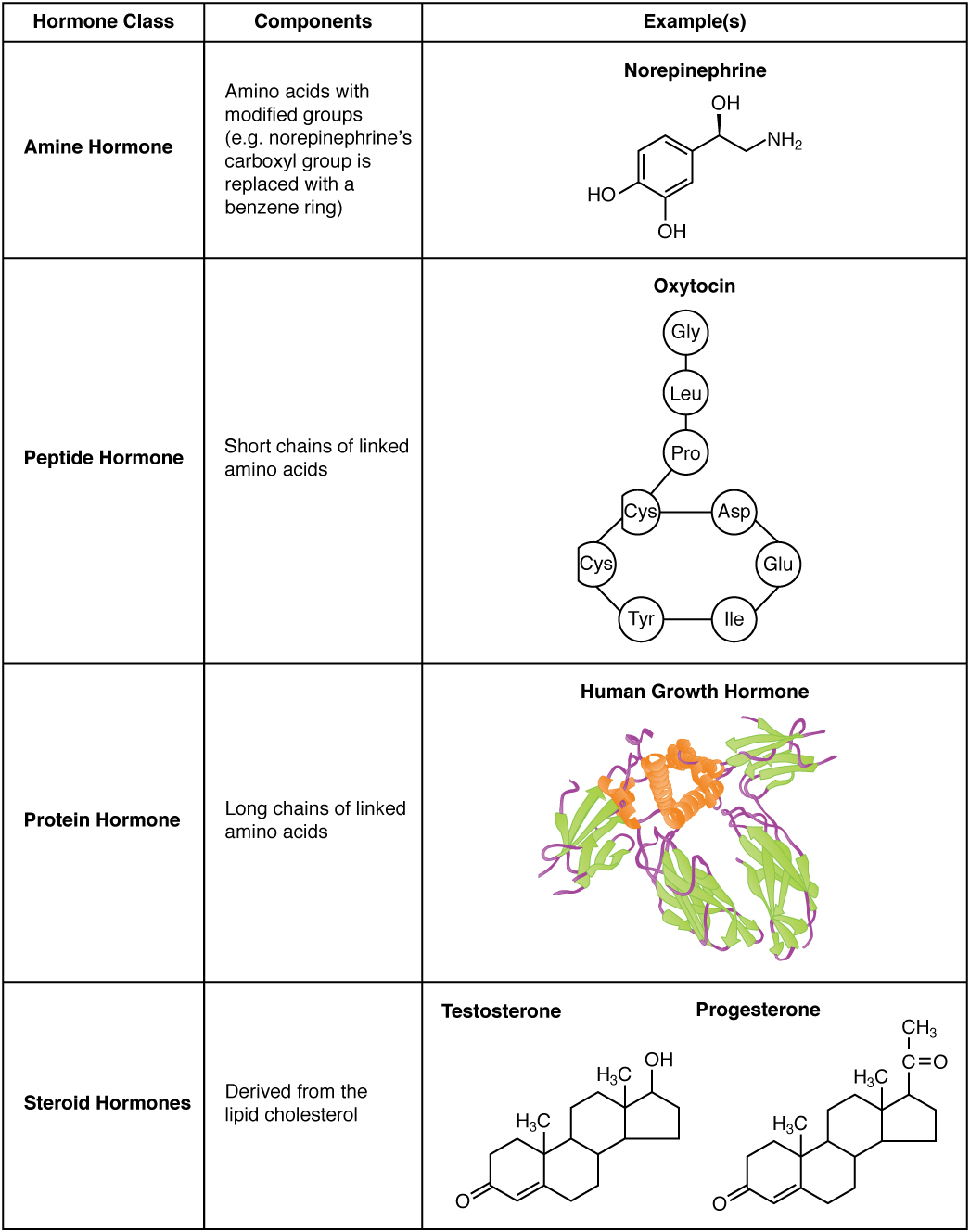
Once the target cell receives the hormone signal, it can respond in a variety of ways. The response may include the stimulation of protein synthesis, activation or deactivation of enzymes, alteration in the permeability of the cell membrane, altered rates of mitosis and cell growth, and stimulation of the secretion of products. Moreover, a single hormone may be capable of inducing different responses in a given cell.
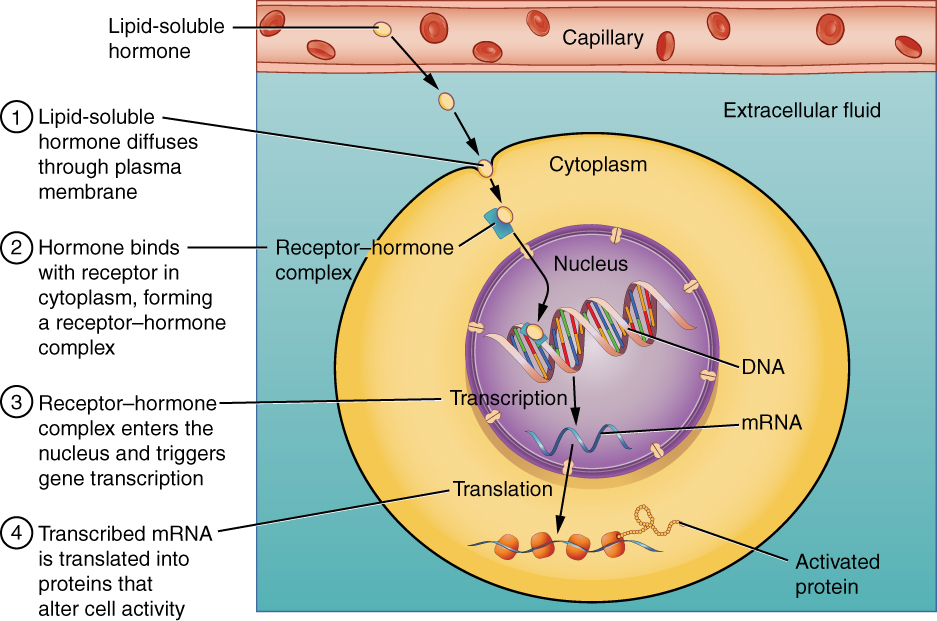
Pathways Involving Intracellular Hormone Receptors
Intracellular hormone receptors are located inside the cell. Hormones that bind to this type of receptor must be able to cross the cell membrane. Steroid hormones are derived from cholesterol and therefore can readily diffuse through the lipid bilayer of the cell membrane to reach the intracellular receptor (Figure 2). Thyroid hormones are also lipid-soluble and can enter the cell. Both hormones bind to DNA within the nucleus and trigger protein synthesis. The particular proteins synthesized will exert an effect.
Pathways Involving Cell Membrane Hormone Receptors
Hydrophilic, or water-soluble, hormones are unable to diffuse through the lipid bilayer of the cell membrane and must therefore pass on their message to a receptor located at the surface of the cell (Figure 3). Except for thyroid hormones, which are lipid-soluble, all amino acid–derived hormones bind to cell membrane receptors that are located, at least in part, on the extracellular surface of the cell membrane. Therefore, they do not directly affect the production of proteins, but instead initiate a signaling cascade (a series of sequential activation of enzymes within the cell) that can trigger a wide variety of effects, from nutrient metabolism to the synthesis of different hormones and other products. The effects vary according to the type of target cell and which signaling cascade is activated inside the cell. Examples of hormones that use this mechanism include calcitonin, which is important for bone construction and regulating blood calcium levels, and glucagon, which affects blood glucose levels.
Overall, such signaling cascades significantly increase the efficiency, speed, and specificity of the hormonal response, as thousands of signaling events can be initiated simultaneously in response to a very low concentration of hormone in the bloodstream, so the action of the hormone can be rapid and substantial. Additionally, the duration of this type of hormone signal is short.
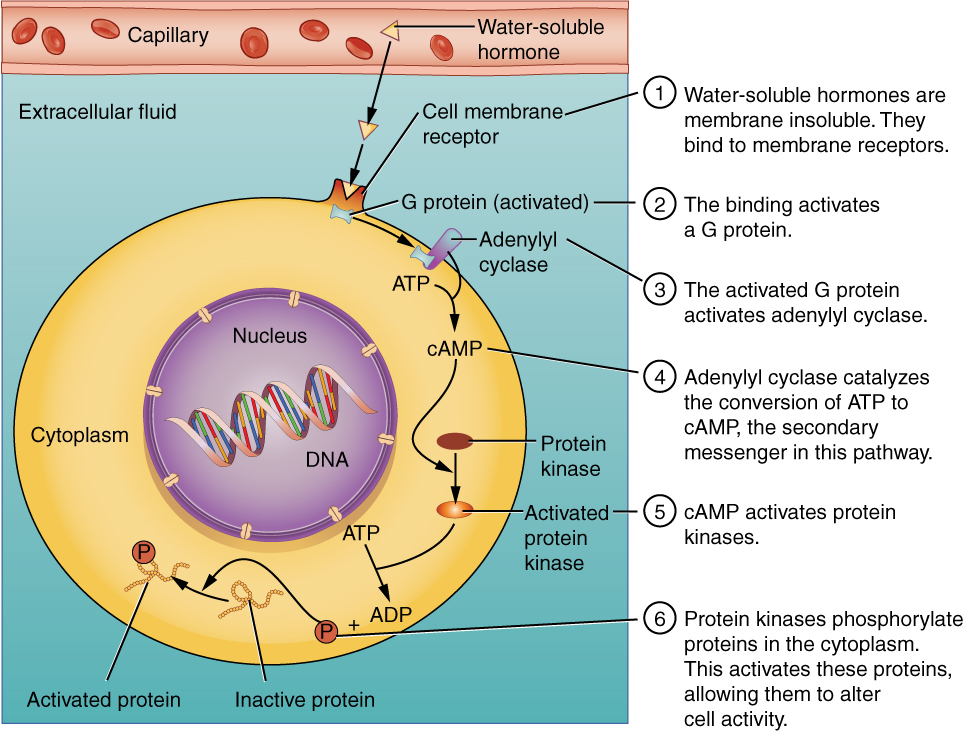
Comparison of the endocrine and nervous systems
Communication is a process in which a sender transmits signals to one or more receivers to control and coordinate actions. The part that the endocrine system plays in this has been stated, however in the human body, another major organ system participates in relatively “long distance” communication: the nervous system. Together, these two systems are primarily responsible for maintaining homeostasis in the body. Although both systems function to allow communication within the body, there are some significant differences in the anatomy and physiology and thus how the function is carried out between the endocrine and nervous systems.
The nervous system uses two types of intercellular communication—electrical and chemical signaling—either by the direct action of an electrical potential, or in the latter case, through the action of chemical neurotransmitters such as serotonin or norepinephrine. Neurotransmitters act locally and rapidly. When an electrical signal in the form of an action potential arrives at a synaptic terminal, it results in the release of neurotransmitters, which diffuse across the synaptic cleft (the gap between a sending neuron and a receiving neuron or muscle cell). Once the neurotransmitters interact (bind) with receptors on the receiving (post-synaptic) cell, the receptor stimulation is transduced into a response such as continued electrical signaling or modification of cellular response. The target cell responds within milliseconds of receiving the chemical “message”; this response then ceases very quickly once the neural signaling ends. In this way, neural communication enables body functions that involve quick, brief actions, such as movement, sensation, and cognition.
In contrast, the endocrine system uses just one method of communication: chemical signaling through hormones, which are secreted into the extracellular fluid. As previously stated, hormones are transported (primarily) via the bloodstream throughout the body, where they bind to receptors on target cells, inducing a characteristic response. As a result, endocrine signaling requires more time than neural signaling to prompt a response in target cells, though the precise amount of time varies with different hormones. For example, the hormones released when you are confronted with a dangerous or frightening situation, called the fight-or-flight response, occur by the release of adrenal hormones—epinephrine and norepinephrine—within seconds. In contrast, it may take up to 48 hours for target cells to respond to certain reproductive hormones. Similarly, due to the mechanism of transmission of hormonal signals, the effect tends to last longer than a nervous stimulation.
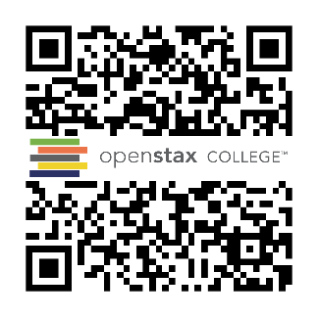
In addition, endocrine signaling is typically less specific than neural signaling. The same hormone may play a role in a variety of different physiological processes depending on the target cells involved. For example, the hormone oxytocin promotes uterine contractions in women in labor. It is also important in breastfeeding and may be involved in the sexual response and in feelings of emotional attachment in both males and females.
In general, the nervous system involves quick responses to rapid changes in the external environment, and the endocrine system is usually slower acting—taking care of the internal environment of the body, maintaining homeostasis, and controlling reproduction (Table 2).
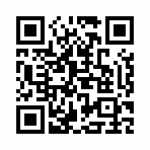
So how does the fight-or-flight response that was mentioned earlier happen so quickly if hormones are usually slower acting? It is because the two systems are connected. It is the fast action of the nervous system in response to the danger in the environment that stimulates the adrenal glands to secrete their hormones. As a result, the nervous system can cause rapid endocrine responses to keep up with sudden changes in both the external and internal environments when necessary.
Endocrine system | Nervous system | |
---|---|---|
Signaling mechanism(s) | Chemical | Chemical / electrical |
Primary chemical signal | Hormones | Neurotransmitters |
Distance travelled by chemical signal | Long or short | Always short |
Response time | Fast or slow | Always fast |
Duration of response | Longer than nervous | Very short |
Environment targeted | Internal | Internal and external |
Part 2. Major endocrine organs and their secretions
The major endocrine glands are shown in Figure 4, and are listed along with their associated hormones and their effects in Table 3. Note that hypothalamic hormones are not listed in Table 3; these consist of releasing/inhibiting hormones for the anterior pituitary hormones, and two hormones that are made by the hypothalamus, but actually stored and then secreted by the posterior pituitary. They are discussed in more detail in the next section of this chapter.
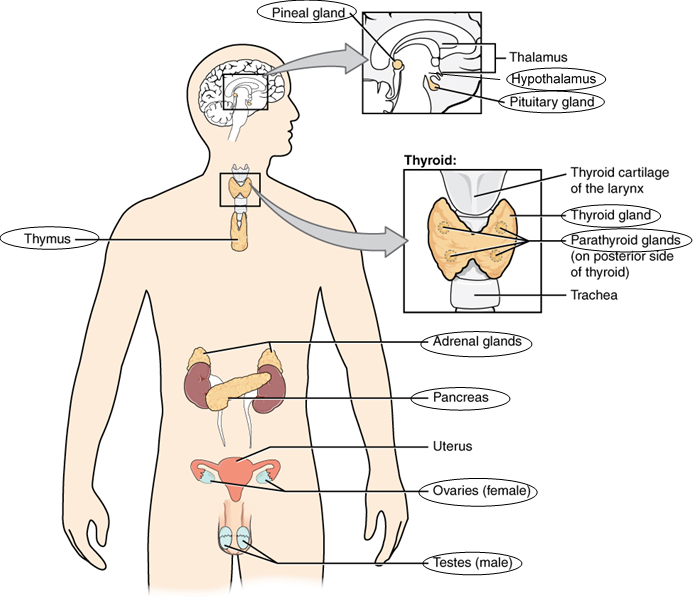
Endocrine gland | Associated hormones | Chemical class | Ultimate effect |
Anterior pituitary | Growth hormone (GH) | Protein | Promotes growth of body tissues |
Anterior pituitary | Prolactin (PRL) | Peptide | Promotes milk production |
Anterior pituitary | Thyroid-stimulating hormone (TSH) | Glycoprotein | Stimulates thyroid hormone release |
Anterior pituitary | Adrenocorticotropic hormone (ACTH) | Peptide | Stimulates hormone release by adrenal cortex |
Anterior pituitary | Follicle-stimulating hormone (FSH) | Glycoprotein | Stimulates gamete production |
Anterior pituitary | Luteinizing hormone (LH) | Glycoprotein | Stimulates androgen production by gonads |
Posterior pituitary | Antidiuretic hormone (ADH) | Peptide | Stimulates water reabsorption by kidneys |
Posterior pituitary | Oxytocin | Peptide | Stimulates uterine contractions during childbirth; may increase sperm motility and testosterone production; modulates social behaviour and social bonding |
Thyroid | Thyroxine (T4), triiodothyronine (T3) | Amine | Generally increase basal metabolic rate |
Thyroid | Calcitonin | Peptide | Reduces blood Ca2+ levels |
Parathyroid | Parathyroid hormone (PTH) | Peptide | Increased blood Ca2+ levels |
Adrenal cortex | Aldosterone | Steroid | Increases blood Na+ levels |
Adrenal cortex | Cortisol, corticosterone, cortisone (CORT) | Steroid | Increase blood glucose levels |
Adrenal medulla | Epinephrine (adrenaline), norepinephrine (noradrenaline) | Amine | Stimulate fight-or-flight response |
Pineal | Melatonin | Amine | regulates sleep cycles |
Pancreas | Insulin | Protein | Reduces blood glucose levels |
Pancreas | Glucagon | Protein | Increases blood glucose levels |
Testes | Testosterone (T) | Steroid | Stimulates development of male secondary sex characteristics and sperm production |
Ovaries | Estrogens, progesterone | Steroid | Stimulate development of female secondary sex characteristics and prepare the body for childbirth |
Hypothalamus
The Hypothalamus and Pituitary Gland
The hypothalamus–pituitary complex can be thought of as the “command center” of the endocrine system for basically two reasons. Besides secreting several hormones that directly produce responses in target tissues, it secretes hormones that regulate the synthesis and secretion of hormones of other endocrine glands. In addition, the hypothalamus–pituitary complex coordinates the messages of the endocrine and nervous systems. In many cases, a stimulus received by the nervous system must pass through the hypothalamus–pituitary complex to be translated into hormones that can initiate a response.
The hypothalamus is a structure of the diencephalon of the brain located anterior and inferior to the thalamus (Figure 5). The hypothalamus is anatomically and functionally related to the pituitary gland (or hypophysis), a bean-sized organ suspended from it by a stem called the infundibulum (or pituitary stalk).
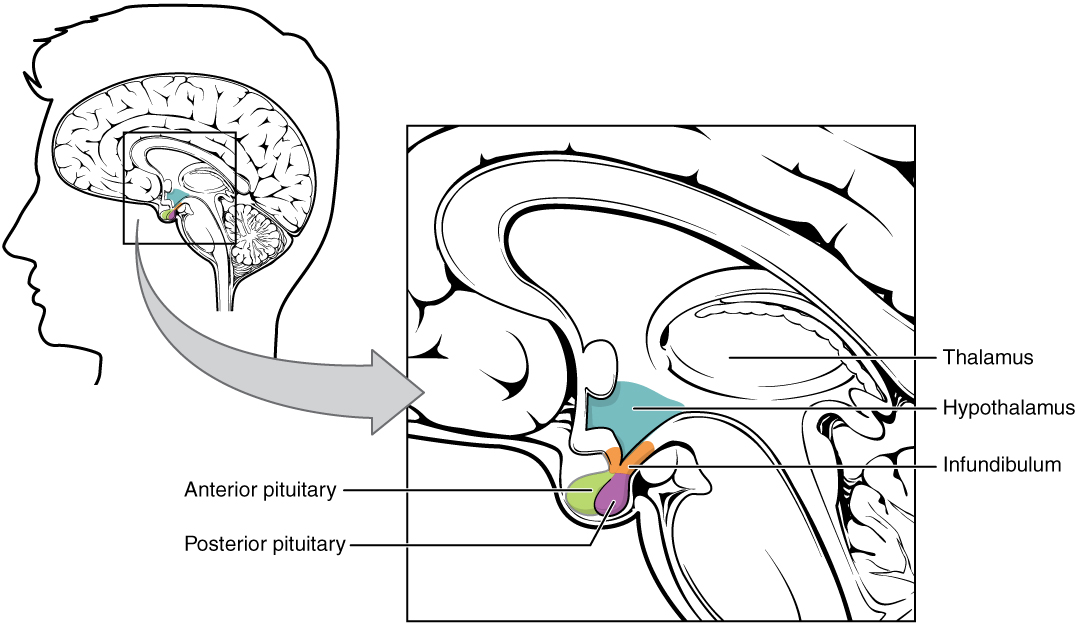
The pituitary gland is cradled within a cup-like hollow in the sphenoid bone of the skull. It consists of two lobes that arise from different parts of embryonic tissue: the anterior pituitary (also known as the adenohypophysis) is glandular tissue that develops from the primitive digestive tract, whereas the posterior pituitary (neurohypophysis) is neural tissue that is essentially an extension of the hypothalamus.
Hormonal secretion by the hypothalamus
All of the hormones that the hypothalamus produces either are directly secreted by the hypothalamus and control the release of hormones by the anterior pituitary (six of these will be discussed below) or are stored in and released by the posterior pituitary (there are two, as presently discussed).
Hypothalamic control of anterior pituitary gland secretion
The secretion of all hormones from the anterior pituitary is regulated by two classes of hormones secreted by the hypothalamus: releasing hormones that stimulate the secretion of hormones from the anterior pituitary, and inhibiting hormones that inhibit secretion (i.e., the anterior pituitary never increases or decreases the release of one of its hormones, without being signaled to do so by the hypothalamus).
Hypothalamic hormones that control the anterior pituitary are secreted by neurons in the hypothalamus and enter the anterior pituitary through blood vessels (Figure 6). Within the infundibulum (the connecting tissue between the hypothalamus and the pituitary) is a bridge of capillaries that connects the hypothalamus to the anterior pituitary. This network, called the hypophyseal portal system, allows hypothalamic hormones to be transported to the anterior pituitary without first entering the systemic circulation. Hormones produced by the anterior pituitary in response to these releasing or inhibiting hormones sent by the hypothalamus then enter a secondary capillary plexus, and from there drain into the general circulation.
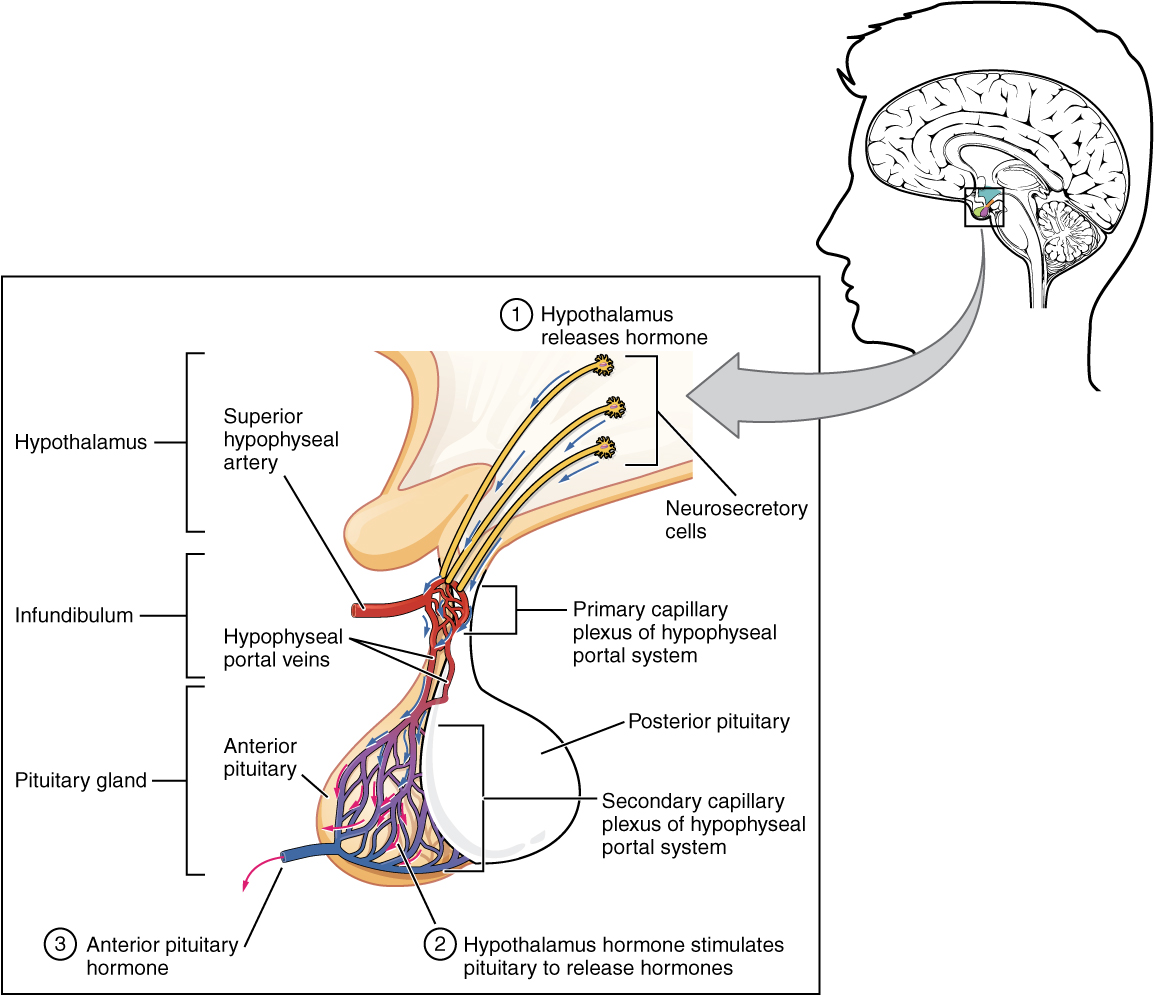
Four of the hormones the hypothalamus produces act as releasing factors which stimulate the secretion of five separate hormones from the anterior pituitary gland. These four releasing hormones are named after the pituitary hormones whose secretions they stimulate:
- Adrenocorticotropic hormone releasing hormone (ACTHRH, or CRH for corticotropin releasing hormone)
- Thyroid stimulating hormone releasing hormone (TSHRH, or TRH for thyrotropin releasing hormone)
- Growth hormone releasing hormone (GHRH)
- Gonadotropin releasing hormone (GnRH), which stimulates the release of hormones known as gonadotropins: follicle stimulating hormone (FSH) and luteinizing hormone (LH)
The hypothalamus also produces inhibiting factors, including growth hormone inhibiting hormone (GHIH) and prolactin inhibiting hormone (PIH).
Cells of the hypothalamus also produce hormones that are stored in and secreted by the posterior pituitary, rather than being secreted from the hypothalamus itself. The hypothalamus produces the hormones oxytocin and antidiuretic hormone, both of which are transported, stored in and then released from the posterior pituitary gland (discussed in the section describing secretions of the posterior pituitary) (Fig. 7).
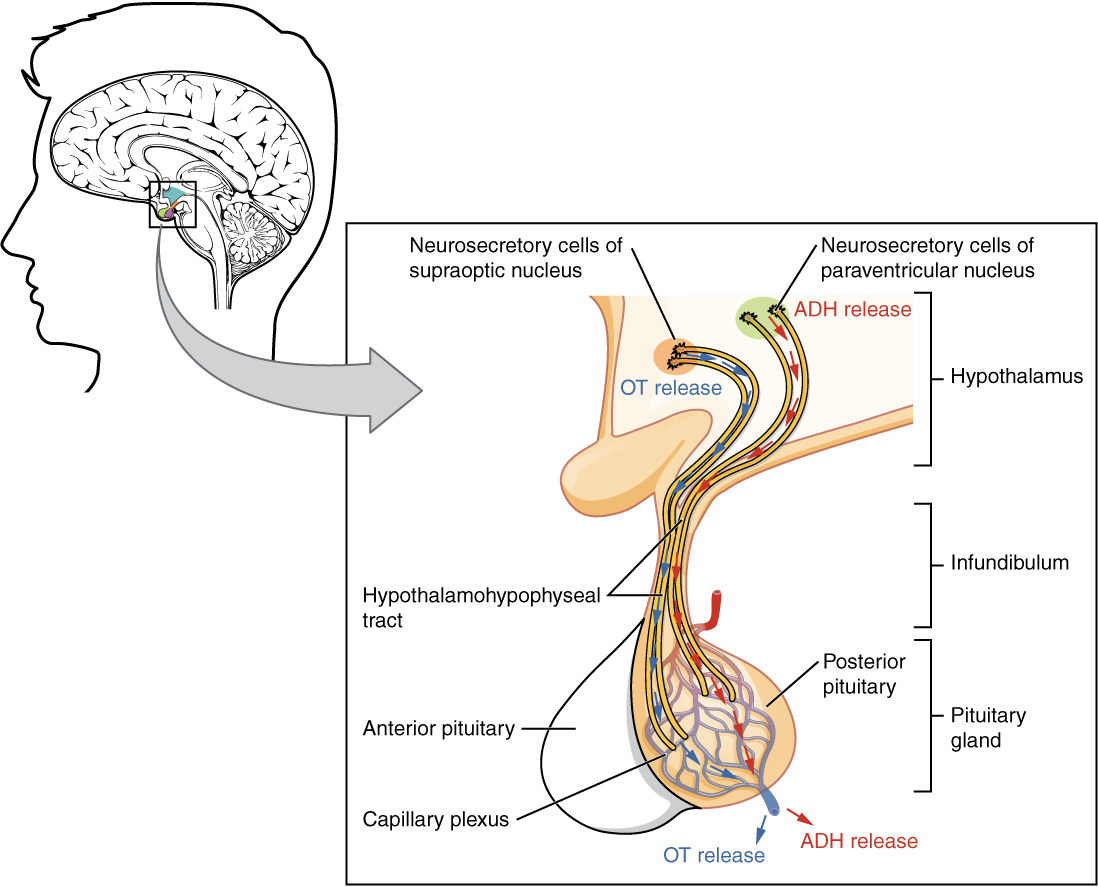
Hormonal secretion by the anterior pituitary (Table 4)
The anterior pituitary produces several hormones, including growth hormone (GH) and prolactin, neither of which affect other endocrine glands, and thyroid-stimulating hormone (TSH), adrenocorticotropic hormone (ACTH), follicle-stimulating hormone (FSH), and luteinizing hormone (LH). Of the hormones of the anterior pituitary, these last four (TSH, ACTH, FSH, and LH) are collectively referred to as tropic hormones (the suffix “tropic” = “turning towards / having an influence on”) because they travel to and affect the function of other endocrine glands.
Growth Hormone
The endocrine system regulates the growth of the human body, protein synthesis, and cellular replication. A major hormone involved in this process is growth hormone (GH), also called somatotropin (“soma” means body; “tropin” means going towards/having an effect on) —a protein hormone produced and secreted by the anterior pituitary gland. Its primary function is anabolic; it promotes protein synthesis and tissue building, increases catabolism of fats and generally slows down the catabolism of carbohydrates (thus helping to maintain blood glucose levels) (Figure 8). GH levels are controlled by the release of growth hormone-releasing hormone (GHRH) and growth hormone-inhibiting hormone (GHIH, also known as somatostatin) from the hypothalamus.
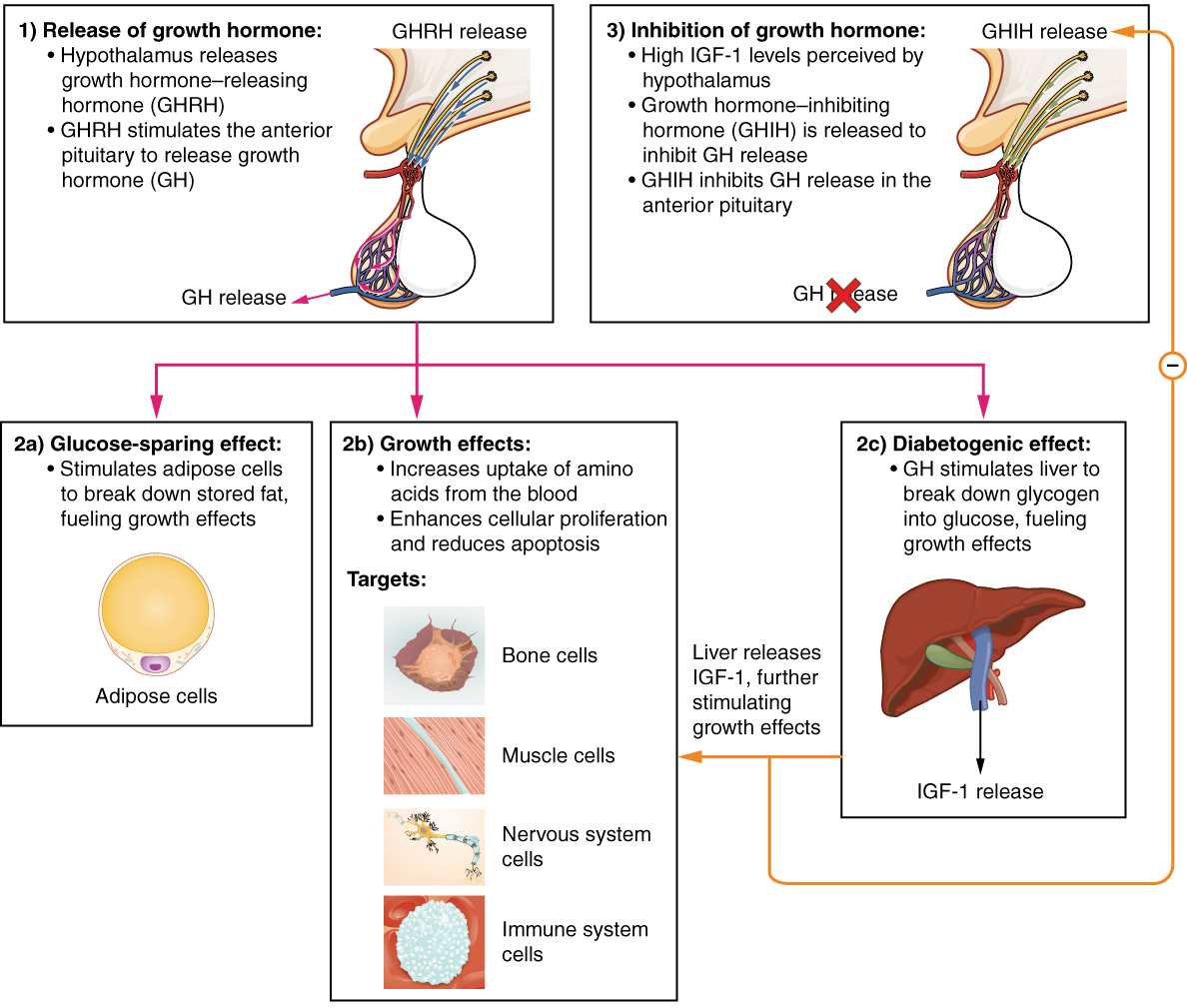
Disorders of Growth Hormone Secretion
Any abnormal levels of secretion of GH would affect all the above listed processes. In general, any underproduction of a hormone is called hyposecretion, and an overproduction is called hypersecretion.
Hyposecretion of GH during childhood (that is, during the growing years), results in a condition known as pituitary dwarfism. Under these circumstances, the epiphyseal plates in the long bones close before the person has grown to normal height. The other body organs also do not grow normally, so a person with pituitary dwarfism has many childlike characteristics. The situation is readily treated by administering growth hormone to the individual before the epiphyseal plates close.
Growth hormone is produced throughout life, and is necessary for normal metabolism and maintenance of body tissues in the adult. A hyposecretion of GH in the adult results in a condition known as Simmonds’ disease. This is characterized by extreme weakness, a wasting of body tissues and a loss of weight.
If there is a hypersecretion of GH during childhood, the individual will be abnormally large. This condition is known as gigantism.
In an adult, GH can no longer lengthen long bones, but excessive amounts can cause very slow growth of various parts of the body. Hypersecretion of GH results in a condition known as acromegaly, which is characterized by the very slow continual growth and thickening of bones (especially those of the hands, feet, cheeks and jaws) and also growth/thickening of organs such as the heart, liver and kidneys, as well as other tissues such as eyelids, forehead, lips, tongue and nose.
Prolactin
As its name implies, prolactin (PRL) promotes lactation (milk production) in women. During pregnancy, it contributes to the development of the mammary glands, and after birth, it stimulates the mammary glands to produce breast milk. (As will be noted later, the let-down (release) of milk from the breasts occurs in response to stimulation from oxytocin.)
In a non-pregnant woman, prolactin secretion is inhibited by prolactin-inhibiting hormone (PIH), which is actually the neurotransmitter dopamine, and is released from neurons in the hypothalamus. Only during pregnancy do prolactin levels rise in response to prolactin-releasing hormone (PRH) from the hypothalamus.
The Tropic Hormones
Thyroid-Stimulating Hormone
The activity of the thyroid gland is regulated by thyroid-stimulating hormone (TSH), also called thyrotropin, released from the anterior pituitary. In turn, TSH is released from the anterior pituitary in response to thyrotropin-releasing hormone (TRH or TSHRH) from the hypothalamus. As discussed shortly, TSH triggers the secretion of thyroid hormones by the thyroid gland. In a classic negative feedback mechanism, elevated levels of thyroid hormones in the bloodstream then trigger a decrease in production of TRH and subsequently TSH.
Adrenocorticotropic Hormone
The adrenocorticotropic hormone (ACTH), also called corticotropin, stimulates the adrenal cortex (the outer layer of the adrenal glands) to secrete corticosteroid hormones such as cortisol.
The release of ACTH is regulated by the corticotropin-releasing hormone (CRH) from the hypothalamus in response to normal physiologic rhythms (The release of ACTH typically peaks in the morning, and reaches its lowest levels in late evening). A variety of stressors can also influence its release, and the role of ACTH in the stress response is discussed later in this unit.
Follicle-Stimulating Hormone and Luteinizing Hormone
Several endocrine glands secrete a variety of hormones that control the development and regulation of the reproductive system (these glands include the anterior pituitary, the adrenal cortex, and the gonads – the testes in males and the ovaries in females). Much of the development of the reproductive system occurs during puberty and is marked by the development of sex-specific characteristics in both male and female adolescents. Puberty is initiated by gonadotropin-releasing hormone (GnRH), a hormone produced and secreted by the hypothalamus. GnRH stimulates the anterior pituitary to secrete gonadotropins—hormones that regulate the function of the gonads. The levels of GnRH are regulated through a negative feedback mechanism; high levels of reproductive hormones inhibit the release of GnRH. Throughout life, gonadotropins regulate reproductive function and, in the case of women, the onset and cessation of reproductive capacity.
The gonadotropins include two hormones: 1) Follicle-stimulating hormone (FSH) which stimulates the production and maturation of sex cells, or gametes (ova in females and sperm in males). FSH also promotes follicular growth; these follicles then release estrogens in the ovaries. 2) Luteinizing hormone (LH) triggers ovulation, as well as the production of estrogens and progesterone by the ovaries. LH stimulates production of testosterone by the testes.
Hormonal secretion by the posterior pituitary (Table 5)
The posterior pituitary is actually an extension of neurons that originate in two specific nuclei (clusters of neuronal cell bodies) in the hypothalamus. While the cell bodies of these neurons rest in the hypothalamus, their axons descend as the hypothalamic–hypophyseal tract within the infundibulum, and end in axon terminals that comprise the posterior pituitary (Figure 7).
The posterior pituitary gland does not produce hormones, but rather stores and secretes hormones produced by the hypothalamus. Neuronal cell bodies of one group of cells in the hypothalamus produces the hormone oxytocin, whereas neuronal cell bodies of another group of cells produces antidiuretic hormone (ADH). These hormones then travel along the axons belonging to the respective neurons into storage sites in the axon terminals of the posterior pituitary. In response to later signals from the hypothalamus, the hormones are then released from the axon terminals into the bloodstream.
Oxytocin
When fetal development is complete, the peptide-derived hormone oxytocin (tocia- = “childbirth”) stimulates uterine contractions and dilation of the cervix. Oxytocin is continually released throughout childbirth through a positive feedback mechanism that continues until birth.
Although the mother’s high blood levels of oxytocin begin to decrease immediately following birth, oxytocin continues to play a role in maternal and newborn health. First, oxytocin is necessary for the milk ejection reflex (commonly referred to as “let-down”) in breastfeeding women. Secondly, in both males and females, oxytocin is thought to contribute to parent–newborn bonding, known as attachment. In general, oxytocin is also thought to be involved in feelings of love and closeness, as well as in the sexual response.
Antidiuretic Hormone (ADH)
ADH is an important hormone of the urinary system. The solute concentration of the blood, or blood osmolarity, may change in response to the consumption of certain foods and fluids, as well as in response to disease, injury, medications, or other factors. Blood osmolarity is constantly monitored by osmoreceptors—specialized cells within the hypothalamus that are particularly sensitive to the concentration of sodium ions and other solutes.
In response to high blood osmolarity, which can occur during dehydration or following a very salty meal, the osmoreceptors in the hypothalamus signal the posterior pituitary to release antidiuretic hormone (ADH). The target cells of ADH are located in the tubular cells of the kidneys. Its effect is to increase epithelial permeability to water, allowing increased water reabsorption. A greater concentration of water in the blood results in a reduced concentration of solutes. ADH is also known as vasopressin because, in very high concentrations, it causes constriction of blood vessels, which increases blood pressure by increasing peripheral resistance. The release of ADH is controlled by a negative feedback mechanism. As blood osmolarity decreases, the hypothalamic osmoreceptors sense the change and prompt a corresponding decrease in the secretion of ADH. As a result, less water is reabsorbed from the urine filtrate.
Disorder of Antidiuretic Hormone Secretion
Interestingly, drugs can affect the secretion of ADH. For example, alcohol consumption inhibits the release of ADH, resulting in increased urine production that can eventually lead to dehydration and a hangover. A disease called diabetes insipidus is characterized by chronic underproduction of ADH that causes chronic dehydration. Because little ADH is produced and secreted, not enough water is reabsorbed by the kidneys. Although patients feel thirsty, and increase their fluid consumption, this doesn’t effectively decrease the solute concentration in their blood because ADH levels are not high enough to trigger water reabsorption in the kidneys. Electrolyte imbalances can occur in severe cases of diabetes insipidus.
Important note: The term “diabetes” simply means overflow, referring to the copious amounts of urine produced due to an excessive loss of water. In the case of diabetes insipidus, the excessive loss of water into the urine is caused by the underproduction of ADH, leading to pale (insipid) urine. In the case of diabetes mellitus, the overflow is due to the presence of glucose in the urine, caused by the lack of sufficient or effective insulin, which will be covered later in this chapter. These are two separate diseases caused by the insufficiency of two different hormones.
Pituitary hormone | Hypothalamic releasing (+) or inhibiting (-) hormone | Target | Effects Include |
---|---|---|---|
Tropic hormones: | |||
Lutenizing hormone (LH) | Gonadotropin-releasing hormone (GnRH) (+) | Reproductive system | Stimulates production of sex hormones by the gonads |
Follicle-stimulating hormone (FSH) | GnRH (+) | Reproductive system | Stimulates production of sperm and eggs |
Thyroid-stimulating hormone (TSH) | Thyroid releasing hormone (TRH) (+) | Thyroid gland | Stimulates the release of thyroid hormone (TH). TH regulates metabolism. |
Adrenocorticotropic hormone (ACTH) | Corticotropin-releasing hormone (CRH) (+) | Adrenal cortex | Induces targets to produce glucocorticoids, which regulate metabolism and then stress response |
Non-tropic hormones: | |||
Prolactin (PRL) | Prolactin-releasing hormone (PRH) (+) or prolactin-inhibiting hormone (PIH) (-) | Mammary glands | Promotes milk production |
Growth hormone (GH) | Growth hormone-releasing hormone (GHRH) (+) or growth hormone-inhibiting hormone (GHIH) (-) | Liver, bone, muscles | Induces targets to produce compounds that stimulate body growth and a higher metabolic rate. |
Released from posterior pituitary | Targets include | Effects include |
---|---|---|
Oxytocin | Female reproductive system | Triggers uterine contractions during childbirth |
Antidiuretic hormone (ADH) | Kidneys, sweat glands, circulatory system | Stimulates water reabsorption in the kidneys |
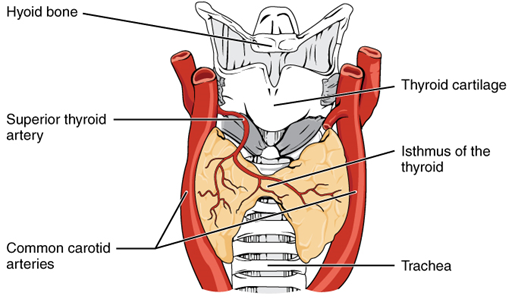
The Thyroid Gland
A butterfly-shaped organ, the thyroid gland is located anterior to the trachea, just inferior to the larynx (Figure 9). The medial region, called the isthmus, is flanked by wing-shaped left and right lobes. Each of the thyroid lobes are embedded with parathyroid glands, primarily on their posterior surfaces. The thyroid gland produces and secretes two hormones: thyroid hormone and calcitonin.
Thyroid Hormone
As with many other hormones, the release of thyroid hormone is under negative feedback control, as a result of stimulation by TSH (thyroid stimulating hormone) released by the anterior pituitary. Recall that TSH is stimulated in turn by the release of TSHRH (thyroid stimulating hormone releasing hormone) released by the hypothalamus. Thyroid hormone actually consists of two slightly different compounds: T3 (triiodothyronine) and T4 (thyroxine). These compounds are often referred to as metabolic hormones because their levels influence the body’s basal metabolic rate, which is the amount of energy used by the body to make ATP at rest. When T3 and T4 bind to intracellular receptors, they cause an increase in nutrient breakdown (thus causing a breakdown of fats and carbohydrates), and the increased use of oxygen to produce ATP. In addition, T3 and T4 initiate the transcription of genes involved in glucose oxidation. The process is inefficient, and an increased amount of heat is released as a byproduct of the increased rate of cellular respiration. This so-called calorigenic effect (calor- = “heat”) raises body temperature.
Adequate levels of thyroid hormones are also required for protein synthesis and for fetal and childhood tissue development and growth. They are especially critical for normal development of the nervous system both in utero and in early childhood, and they continue to support neurological function in adults.
These thyroid hormones also have a complex interrelationship with reproductive hormones, and deficiencies can influence libido, fertility, and other aspects of reproductive function. Finally, thyroid hormones increase the body’s sensitivity to catecholamines (epinephrine and norepinephrine) from the adrenal medulla by upregulation of receptors in the blood vessels and the heart. When levels of T3 and T4 hormones are excessive, this effect accelerates the heart rate, strengthens the heartbeat, and increases blood pressure. Because thyroid hormones regulate metabolism, heat production, protein synthesis, and many other body functions, thyroid disorders can have severe and widespread consequences.
Disorders of Thyroid Hormone Secretion
Hypersecretion of the thyroid hormones may have numerous causes, including an excess production of TSH (thyroid stimulating hormone) by the anterior pituitary gland, the presence of a tumor in the thyroid gland itself, or an immune disorder.
Hypersecretion of thyroid hormone caused by an immune disorder is known as Graves’ disease. Also known as exophthalmic goiter, the effects are similar in children and adults. One symptom is that the thyroid gland becomes enlarged – a condition called goiter (explained below). There is also a very high metabolic rate, which causes the person to have a high pulse rate, lose weight and become nervous and irritable. Inflammation and swelling occurs in the tissue behind the eyes, causing the eyes to protrude. Treatment involves either surgically removing part of the thyroid gland, or suppressing thyroid secretions with drugs.
Hyposecretion of thyroid hormones in childhood leads to cretinism. In this condition, the skeleton fails to grow and mature, and the brain does not develop fully. Other symptoms include general lethargy, a slow heart rate and a low body temperature. In addition, there is a tendency to easily gain weight.
Hyposecretion of thyroid hormone in adulthood is called myxedema. This is characterized by swelling and puffiness of the facial tissue (caused by an increased accumulation of hydrophilic sugar protein complexes in the skin) and lowered body metabolism. There is also a tendency to easily gain weight. Because the brain is already fully developed, there is no mental retardation.
A symptom of many thyroid disorders is a goiter, which is an increase in the overall size of the thyroid gland (Figure 10). Interestingly, a goiter can arise whether the thyroid gland is synthesizing too much or too little of thyroid hormone.
Consistent overstimulation of the thyroid gland that then produces more than normal amounts of thyroid hormone can occur in diseases such as Grave’s disease. Such stimulation may result in an increase in the size of the thyroid gland (= goiter).
On the other hand, underactivity of the thyroid gland would result in lower amounts of thyroid hormone in the blood. This would decrease the negative feedback effect that thyroid hormone has on the production of TRH from the hypothalamus and TSH from the anterior pituitary. The levels of these compounds in the blood will then rise and stay elevated as long as the level of thyroid hormone remains low. This will continuously stimulate the thyroid gland, causing it to increase in size (= goiter). One of the causes for the inability of the thyroid gland to produce thyroid hormone in the first place is a condition known as simple goiter, which occurs when the body’s intake of iodine (which is required for the production of thyroid hormone) is not sufficient for its needs.
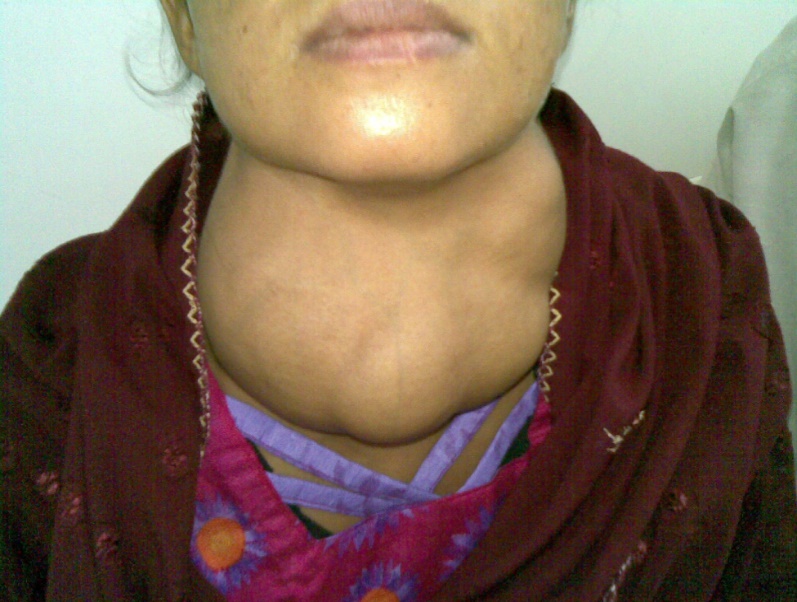
Calcitonin
The thyroid gland also secretes a hormone called calcitonin that is released in response to a rise in blood calcium levels. It appears to have a function in decreasing blood calcium concentrations by:
- Inhibiting the activity of osteoclasts, bone cells that release calcium into the circulation by degrading bone matrix
- Increasing osteoblastic activity (thereby increasing deposition of calcium into bones)
- Decreasing calcium absorption in the intestines
- Increasing calcium loss in the urine
However, these functions are usually not significant in maintaining calcium homeostasis (people with long term increased or decreased calcitonin secretion due to disease usually do not show abnormal blood calcium levels), so the importance of calcitonin is not entirely understood. The production of calcitonin does however respond to levels of calcium in the blood (Figure 12), and pharmaceutical preparations of calcitonin are sometimes prescribed to reduce osteoclast activity in people with osteoporosis and to reduce the degradation of cartilage in people with osteoarthritis.
Calcium is critical for many biological processes, acting as a second messenger in many signaling pathways, and essential for muscle contraction, nerve impulse transmission, and blood clotting. The necessary tight regulation of blood calcium levels is mainly carried out by the parathyroid glands.
The Parathyroid Glands
The parathyroid glands are tiny, round structures usually found embedded in the posterior surface of the thyroid gland (Figure 11). A thick connective tissue capsule separates the glands from the thyroid tissue. Most people have four parathyroid glands. The primary functional cells of the parathyroid glands cells produce and secrete the parathyroid hormone (PTH) (also known as parathormone), the major hormone involved in the regulation of blood calcium levels.
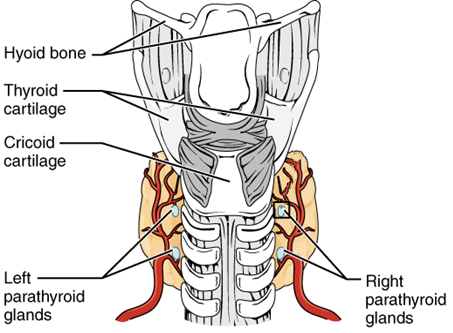
The parathyroid glands produce and secrete PTH, a peptide hormone, in response to low blood calcium levels (Figure 12). PTH secretion causes the increase in blood calcium via the following mechanisms:
- Stimulating the activity of osteoclasts, bone cells that release calcium into the circulation by degrading bone matrix
- Inhibiting osteoblastic activity (thereby decreasing deposition of calcium into bones)
- Increasing calcium absorption in the intestines by initiating the production of the steroid hormone calcitriol (also known as 1,25-dihydroxyvitamin D), which is the active form of vitamin D3, in the kidneys. Calcitriol then stimulates increased absorption of dietary calcium by the intestines.
- Decreasing calcium loss in the urine by causing increased reabsorption of calcium (and magnesium) in the kidney tubules from the urine filtrate
A negative feedback mechanism regulates the levels of PTH, with rising blood calcium levels inhibiting further release of PTH.
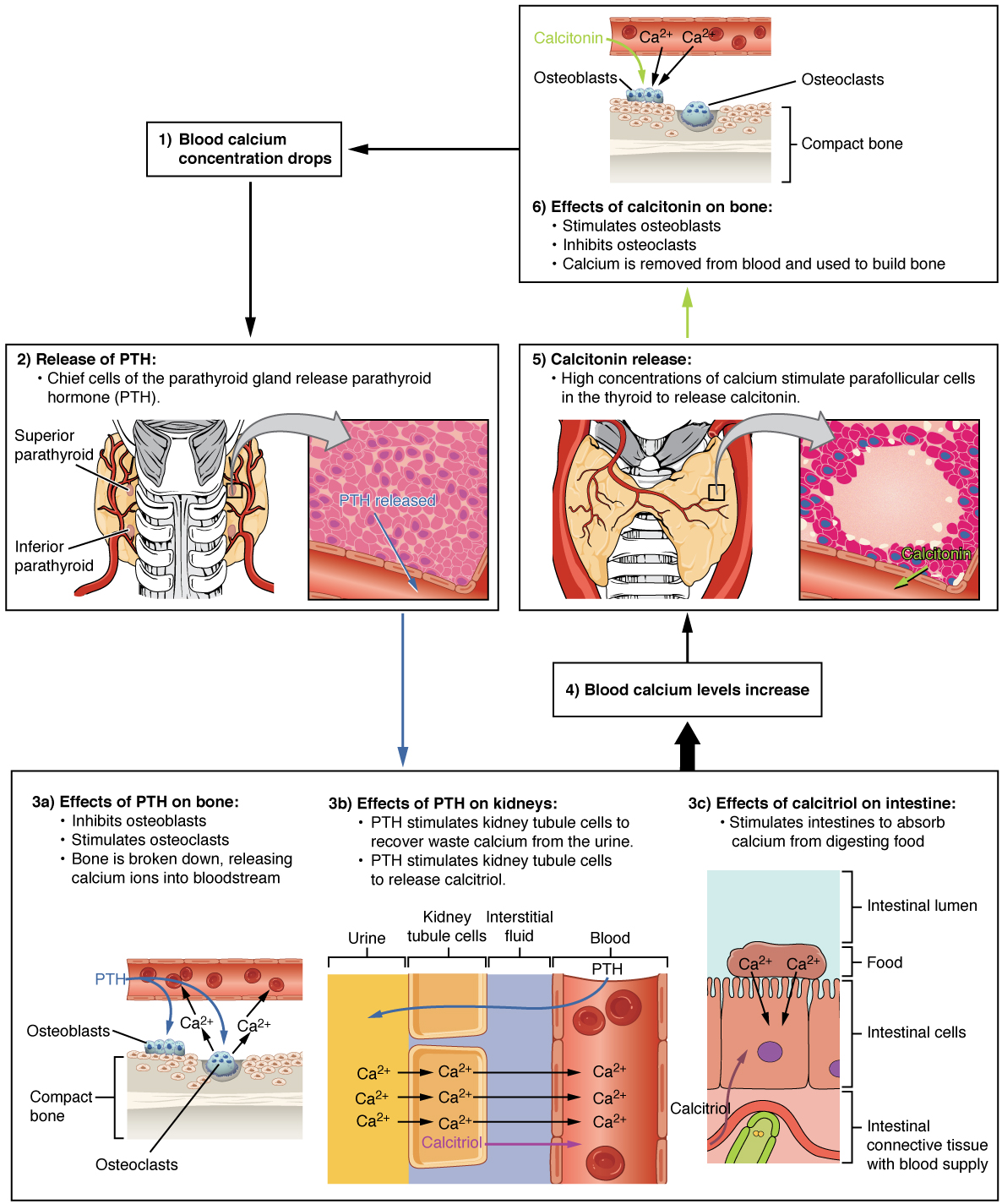
Disorders of parathyroid hormone secretion
Abnormally high activity of the parathyroid gland can cause hyperparathyroidism, a disorder caused by an overproduction of PTH that results in excessive calcium reabsorption from bone. Hyperparathyroidism can significantly decrease bone density, leading to spontaneous fractures or deformities. As blood calcium levels rise, cell membrane permeability to sodium is decreased (the exact mechanism has not yet been clarified), and the responsiveness of the nervous system is reduced. At the same time, calcium deposits may collect in the body’s tissues and organs, impairing their functioning.
In contrast, abnormally low blood calcium levels may be caused by parathyroid hormone deficiency, called hypoparathyroidism, which may develop following injury or surgery involving the thyroid gland. Low blood calcium increases membrane permeability to sodium, resulting in muscle twitching, cramping, spasms, or convulsions, a condition known as tetany. Severe deficits can paralyze muscles, including those involved in breathing, and can be fatal.
The Adrenal Glands
The adrenal glands are wedges of glandular and neuroendocrine tissue adhering to the top of the kidneys by a fibrous capsule (Figure 13). The adrenal glands have a rich blood supply and experience one of the highest rates of blood flow in the body. The adrenal gland consists of an outer cortex of glandular tissue and an inner medulla of nervous tissue.
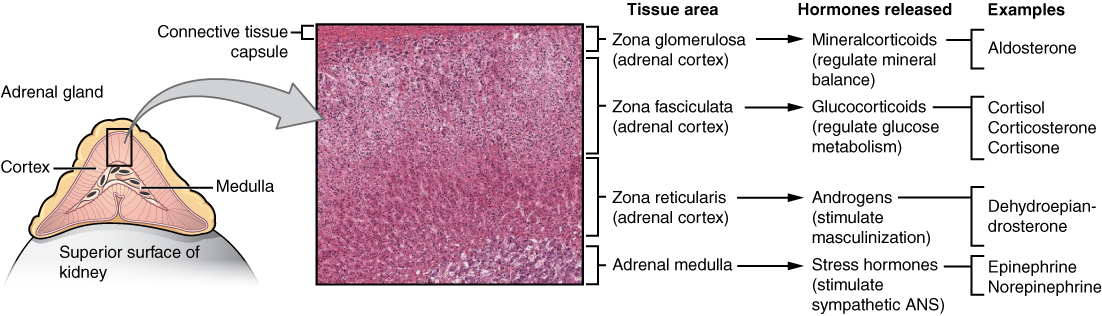
Hormones of the adrenal cortex
Mineralocorticoids
The most superficial region of the adrenal cortex (zona glomerulosa) produces a group of hormones collectively referred to as mineralocorticoids because of their effect on body minerals, especially sodium and potassium. These hormones are essential for fluid and electrolyte balance.
Aldosterone is the major mineralocorticoid. It is important in the regulation of the concentration of sodium and potassium ions in urine, sweat, and saliva. For example, it is released in response to elevated blood K+, low blood Na+, low blood pressure, low blood volume and activation of the renin-angiotensin-aldosterone system (RAAS) (this hormone will be discussed again during the unit dealing with the renal system). In response, aldosterone increases the excretion of K+ and the retention of Na+, which in turn increases blood volume and blood pressure.
Glucocorticoids
The cells of the middle layer (zona fasciculata) produce hormones called glucocorticoids because of their role in glucose metabolism. The most important of these is cortisol. In response to long-term stressors, the hypothalamus secretes CRH, which in turn triggers the release of ACTH by the anterior pituitary. ACTH triggers the release of cortisol. The overall effect is to inhibit tissue building while stimulating the breakdown of stored nutrients to maintain adequate fuel supplies. In conditions of long-term stress, for example, cortisol promotes the catabolism of glycogen to glucose, the catabolism of stored triglycerides into fatty acids and glycerol, and the catabolism of muscle proteins into amino acids. Cortisol increases the body’s resistance to stress by increasing muscle metabolism, maintaining the excitability of nerves and increasing the amount of sugars in the body by promoting gluconeogenesis, the conversion of fats and proteins into sugars.
Androgens
The innermost layer of the cortex (zona reticularis) produces small amounts of sex hormones called androgens, which are converted into testosterone and estrogen in the tissues. The adrenal cortex serves as the source of sex hormones in an individual until the gonads mature at puberty. The sex hormones of the adrenal cortex play a role in the development of secondary sex characteristics in both males and females. In males, they further increase muscle development. It is interesting to note that the production of the cortical sex hormones is under the influence of adrenocorticotropic hormone from the anterior pituitary gland, and not follicle stimulating hormone or luteinizing hormone.
Hormones of the Adrenal Medulla
Epinephrine and norepinephrine (also sometimes called adrenalin and noradrenalin): These two hormones are both involved in the response to fear, excitement and danger. They both increase blood pressure, and the rate and depth of breathing. Epinephrine, however, increases heart rate and blood sugar levels, whereas norepinephrine reduces the blood flow to the gut and skin.
The Stress Response
One of the major functions of the adrenal gland is to respond to stress. Stress can be defined as some occurrence that disrupts homeostasis, and can be either physical or psychological or both. Physical stresses include exposing the body to injury, walking outside in cold and wet conditions without a coat on, or malnutrition. Psychological stresses include the perception of a physical threat, a fight with a loved one, or just a bad day at school.
The body responds in different ways to short-term stress and long-term stress following a pattern known as the general adaptation syndrome. The first stage of the general adaptation syndrome is called the alarm reaction. This “fight-or-flight” response, the result of a short-term stressor, is mediated by the hormones epinephrine and norepinephrine from the adrenal medulla, as stimulated by the sympathetic nervous system. Their function is to prepare the body for extreme physical exertion by increasing the heart rate, dilating the airways, and other related responses. Once this stressor is relieved, the body quickly returns to normal.
If the stressor is not soon relieved, the body attempts to adapt to the stressor in the second stage called the stage of resistance. The primary stress hormone at this stage is cortisol, secreted by the adrenal cortex as a result of signals sent by the hypothalamus and pituitary gland. Cortisol’s effects are widespread. They include the maintenance of blood glucose through synthesizing glucose from compounds such as proteins (gluconeogenesis), and lipolysis so fatty acids can be used for energy by the body, thus preserving glucose. Additionally, the activity of the immune system and inflammation are reduced, as the resources of the body are directed towards dealing with the stress. The physiological adaptations during this stage allow the body to resist the most immediate negative effects of a longer-term stressor.
However, if the stressor continues for a longer term, the final stage of the stress response may occur. This is known as the stage of exhaustion. At this point, the resources of the body have become depleted and individuals may begin to suffer depression, severe fatigue, or even a fatal heart attack. The continued release of cortisol and other hormones associated with long term stress can cause damage to a variety of organ systems, and this condition has been linked to many diseases such as rheumatoid arthritis, hypertension and gastrointestinal diseases.
Disorders of Glucocorticoid (Cortisol) Secretion
Addison’s disease can be caused by an autoimmune attack on the adrenal cortex, resulting in a hyposecretion of adrenal cortex hormones, including glucocorticoids. Since one of the actions of glucocorticoids is maintenance of blood glucose levels, hyposecretion of this hormone is characterized by hypoglycemia. This leads to muscular weakness, mental lethargy and weight loss.
Abnormal hypersecretion of glucocorticoids from the adrenal cortex can be directly caused by a tumor of the adrenal cortex, (or indirectly caused through an overstimulation from other areas of the body; for example, an increased secretion of ACTH from the anterior pituitary gland). A hypersecretion of glucocorticoids due to an adrenal cortex tumour leads to Cushing’s syndrome. Associated with this syndrome is a redistribution of body fats, so the legs become spindly, the face becomes moon-shaped, a buffalo hump appears on the back and the abdomen becomes pendulous. The cause of this redistribution of body fat is poorly understood but is thought to be at least partially due to a difference in the distribution of glucocorticoid receptors between the various body tissues.
Another sign of Cushing’s syndrome is excess blood glucose, to be expected considering cortisol’s effect of increasing blood glucose. Not so expected is the increase in blood sodium that can be observed with this disease (which does not cause an increase in the levels of aldosterone). Cortisol does in fact have a very minor mineralocorticoid effect, but this is negligible at normal levels of cortisol. However, the very large values for blood cortisol that result in Cushing’s syndrome can exert a very noticeable effect, resulting in elevated levels of blood sodium.
The Endocrine Pancreas
The pancreas is a long, slender organ, most of which is located posterior to the bottom half of the stomach (Figure 14). Although it is primarily an exocrine gland, secreting a variety of digestive enzymes, the pancreas has an endocrine function. Its pancreatic islets – clusters of cells formerly known as the islets of Langerhans – secrete the hormones glucagon and insulin.
Cells and Secretions of the Pancreatic Islets
Cells residing in the pancreatic islets include the following two types of cells. The alpha cell produces the hormone glucagon and makes up approximately 20 percent of each islet. Glucagon plays an important role in blood glucose regulation; low blood glucose levels stimulate its release. The beta cell produces the hormone insulin and makes up approximately 75 percent of each islet. Elevated blood glucose levels stimulate the release of insulin.
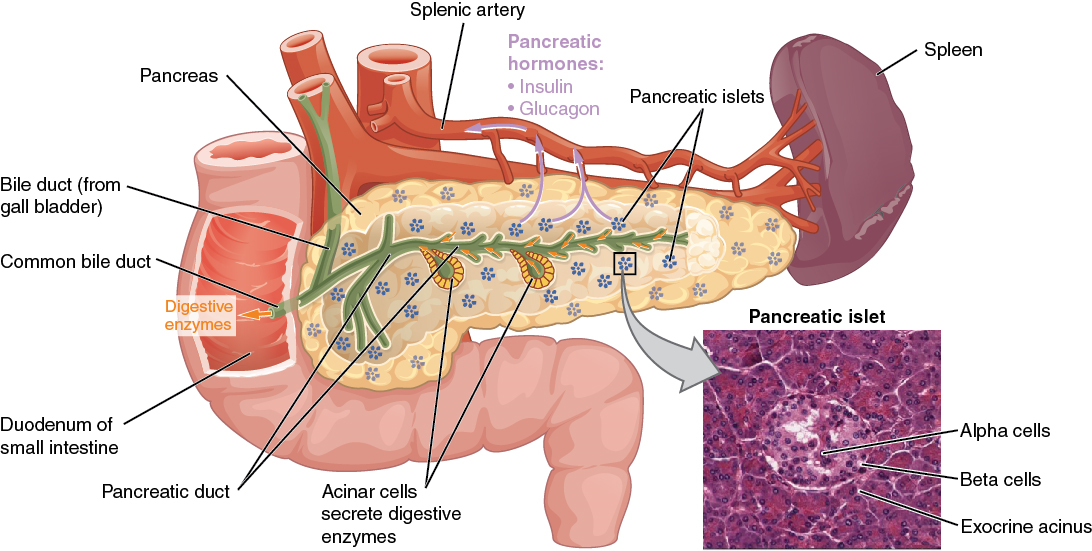
Regulation of Blood Glucose Levels by Insulin and Glucagon
Glucose is required for cellular respiration and is the preferred fuel for all body cells. The body derives glucose from the breakdown of the carbohydrate-containing foods and drinks we consume. Glucose not immediately taken up by cells for fuel can be stored by the liver and muscles as glycogen or converted to triglycerides and stored in the adipose tissue. Hormones regulate both the storage and the utilization of glucose as required. Receptors located in the pancreas sense blood glucose levels, and subsequently the pancreatic cells secrete glucagon or insulin to maintain normal levels.
Glucagon
Receptors in the pancreas can sense the decline in blood glucose levels, such as during periods of fasting or during prolonged labor or exercise (Figure 15). In response, the alpha cells of the pancreas secrete the hormone glucagon, which has several effects:
- It stimulates the liver to perform glycogenolysis, the breaking down of glycogen into its component glucose building blocks. The resulting glucose is then released into the circulation for use by body cells.
- It stimulates gluconeogenesis in the liver, converting amino acids from body proteins into glucose.
- It stimulates lipolysis, the breakdown of stored triglycerides into free fatty acids and glycerol. Some of the free glycerol released into the bloodstream travels to the liver, which converts it into glucose. This is also a form of gluconeogenesis.
Taken together, these actions increase blood glucose levels. The activity of glucagon is regulated through a negative feedback mechanism; rising blood glucose levels inhibit further glucagon production and secretion.
Insulin
The primary function of insulin is to facilitate the uptake of glucose into body cells. Red blood cells, as well as cells of the brain, kidneys, and the lining of the small intestine, do not have insulin receptors on their cell membranes and do not require insulin for glucose uptake. Although all other body cells do require insulin if they are to take glucose from the bloodstream, skeletal muscle cells and adipose cells are the primary targets of insulin.
Insulin also reduces blood glucose levels by stimulating glycolysis, the metabolism of glucose for generation of ATP. Moreover, it stimulates the liver to perform glycogenesis, converting excess glucose into glycogen for storage, and it inhibits enzymes involved in glycogenolysis and gluconeogenesis. Finally, insulin promotes triglyceride and protein synthesis. The secretion of insulin is regulated through a negative feedback mechanism. As blood glucose levels decrease, further insulin release is inhibited.
Disorders of Insulin Secretion / Sensitivity
Dysfunction of insulin production and secretion, as well as the target cells’ responsiveness to insulin, can lead to a condition called diabetes mellitus, traditionally characterized by the “three P’s”: polyphagia (excessive eating), polydipsia (excessive thirst) and polyuria (excessive urine production). Note that this is a different disease than diabetes insipidus, which is a decrease in the secretion of ADH, also causing increased urine production, but no glucose in the urine.
The hormonal control of blood glucose is more complex than just the interaction between insulin and glucagon. Along with glucagon, the following hormones are called “counterregulatory” hormones, because their effects on glucose levels are opposite to that of insulin, i.e., they all act to increase the level of glucose in the blood:
- Epinephrine stimulates the breakdown of glycogen in the liver and muscle (glycogenolysis)
- Growth hormone stimulates the mobilization and breakdown of fats, and decreases the uptake of glucose by fat cells
- Cortisol stimulates the breakdown of proteins and their use in the production of glucose (gluconeogenesis)
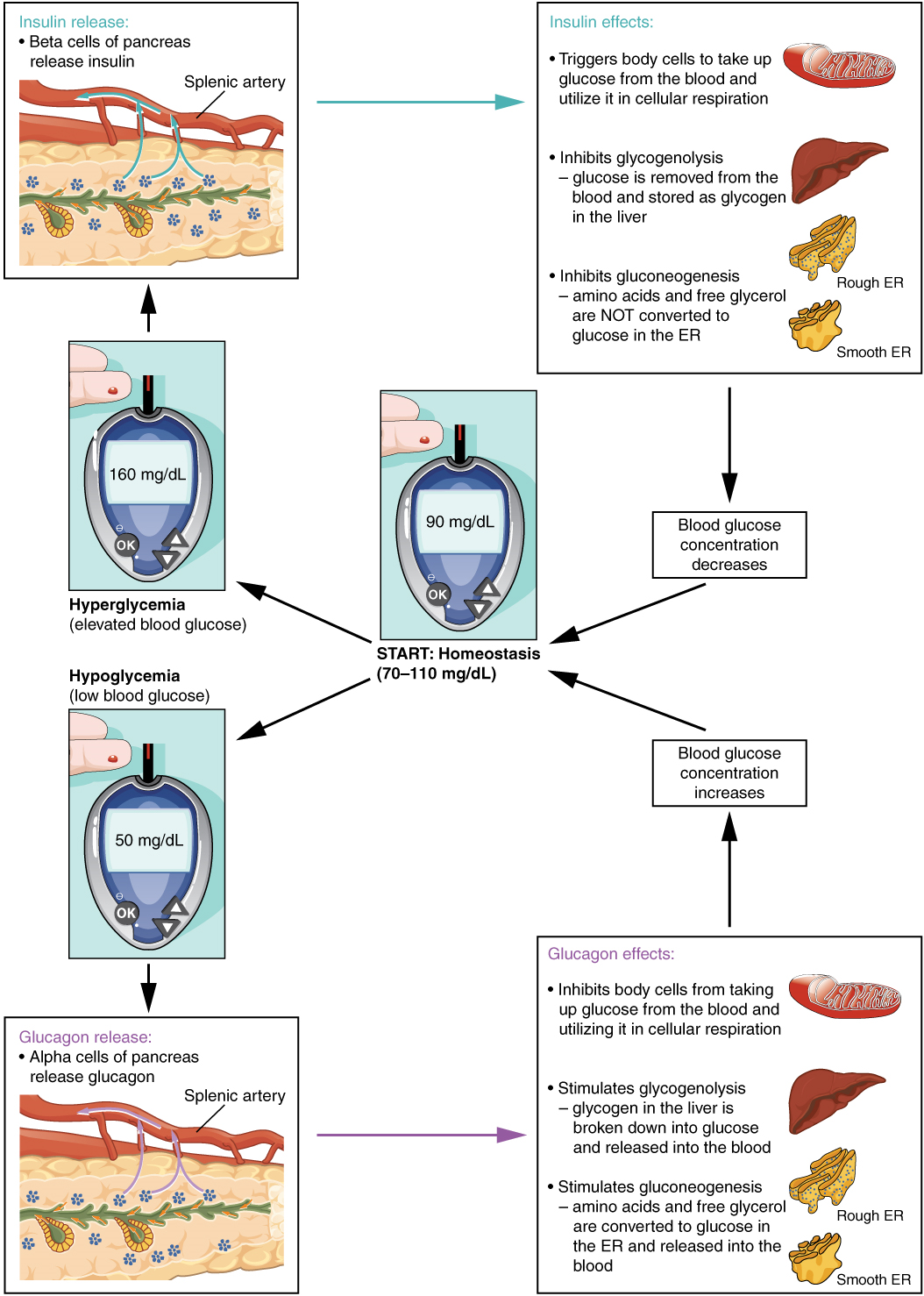
The endocrine functions of the ovaries and testes
The ovaries produce two hormones: estrogen and progesterone. Estrogen is produced by ovarian follicles. Estrogen stimulates the growth of both primary and secondary sex characteristics. Primary sex characteristics in the female include the growth of the uterus and vagina, and the secondary characteristics include the development of body hair, enlarged breasts and a wider pelvis.
Progesterone is produced by the corpus luteum. It stimulates the development of milk-secretory tissue in the breasts, prepares the uterine lining for the implantation of a fertilized oocyte, and helps to maintain pregnancy.
The testes in the male produce the hormone testosterone. This hormone stimulates the development of primary sex characteristics, such as the accessory glands and the penis, and secondary sex characteristics such as body hair and a deepening of the voice.
The endocrine functions of the stomach and the duodenum
The principal function of the stomach is, of course, digestion. We are most familiar with the capacity of the stomach to store food, and its role in the mechanical and chemical digestion of food. However, there is also some endocrine tissue in the stomach. The secretion of hydrochloric acid in the stomach, and some enzymes, is under the control of a hormone called gastrin, which is produced by glandular tissue in the wall of the stomach. This hormone is produced by the stomach, travels through the bloodstream, and stimulates the exocrine tissue of the stomach.
The duodenum also has glandular tissue in its walls. One of the hormones it produces is called secretin. Secretin travels through the blood and stimulates the pancreas to produce pancreatic juice, which then enters the duodenum and aids in digestion.
The thymus and the pineal gland
The thymus gland was once thought to be a vestigial organ. However, it has been determined that it is a central gland of the lymphatic system, which is involved in the body’s immune system. As part of this activity, the gland produces a hormone called thymosin, which is involved in the maturation and development of the immune system. This gland is larger in infants and decreases in size through adulthood. As a result of the change in size of the gland, the amounts of thymosin produced similarly decrease throughout adulthood.
The pineal gland, situated in the brain, produces the hormone melatonin. This hormone acts on the hypothalamus inhibiting the release of luteinizing hormone, thus affecting the activity of the gonads. The pineal gland is an interesting structure, because it is responsive to light and may be involved in the seasonal behaviour changes by some animals in response to changes in day length.
The special nature of the prostaglandins
Prostaglandins are lipids, much like some hormones. However, they are not produced in special organs or glands, but rather by many cell types from lipids in their own plasma membranes. They usually do not travel long distances within the body, but typically have effects on the tissue where they are produced.
The effects of prostaglandins are numerous, including the regulation of blood pressure, regulation of stomach secretions, stimulation and inhibition of uterine contractions, and the transmission of nerve impulses. At least 15 different prostaglandins have been discovered so far. They are vital for the normal functioning of the body. Prostaglandins were originally discovered in the secretions of the prostate gland, from which they got their name.