Chapter 10. Organic Chemistry
10.2 Functional Groups
Learning Objectives
By the end of this section, you will be able to:
- Define functional group.
- Identify and name the functional groups mentioned here.
- Draw the functional groups mentioned here.
- Know the difference between saturated and unsaturated hydrocarbons.
Functional groups are structural units within organic compounds that are defined by specific bonding arrangements between specific atoms. The structure of capsaicin, the compound which is the source of the heat in hot chili peppers, incorporates several functional groups, labeled in the figure below and explained throughout this section.
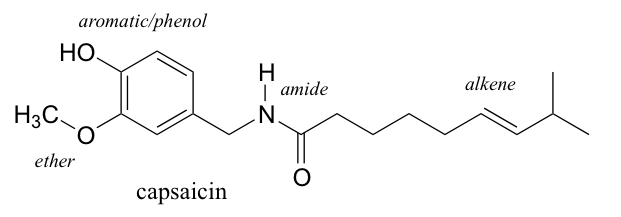
As one progresses their study of organic chemistry, it becomes extremely important to be able to quickly recognize the most common functional groups, because they are the key structural elements that define how organic molecules react. For now, we will only worry about drawing and recognizing each functional group, as depicted by Lewis and line structures, and the nomenclature of simple organic compounds. Much of the remainder of your study of organic chemistry will be taken up with learning about how the different functional groups behave in organic reactions.
The ‘default’ in organic chemistry, essentially, the lack of any functional groups, is given the term alkane, characterized by single bonds between carbon and carbon, or between carbon and hydrogen. Methane, CH4, is the natural gas you may burn in your furnace. Octane, C8H18, is a component of gasoline.
Alkanes are relatively stable molecules, but heat or light will activate reactions that involve the breaking of C–H or C–C single bonds. Combustion is one such reaction:
Alkanes burn in the presence of oxygen, a highly exothermic oxidation-reduction reaction that produces carbon dioxide and water. As a consequence, alkanes are excellent fuels. For example, methane, CH4, is the principal component of natural gas. Butane, C4H10, used in camping stoves and lighters is an alkane. Gasoline is a liquid mixture of continuous- and branched-chain alkanes, each containing from five to nine carbon atoms, plus various additives to improve its performance as a fuel. Kerosene, diesel oil, and fuel oil are primarily mixtures of alkanes with higher molecular masses. The main source of these liquid alkane fuels is crude oil, a complex mixture that is separated by fractional distillation. Fractional distillation takes advantage of differences in the boiling points of the components of the mixture (see Figure 2).
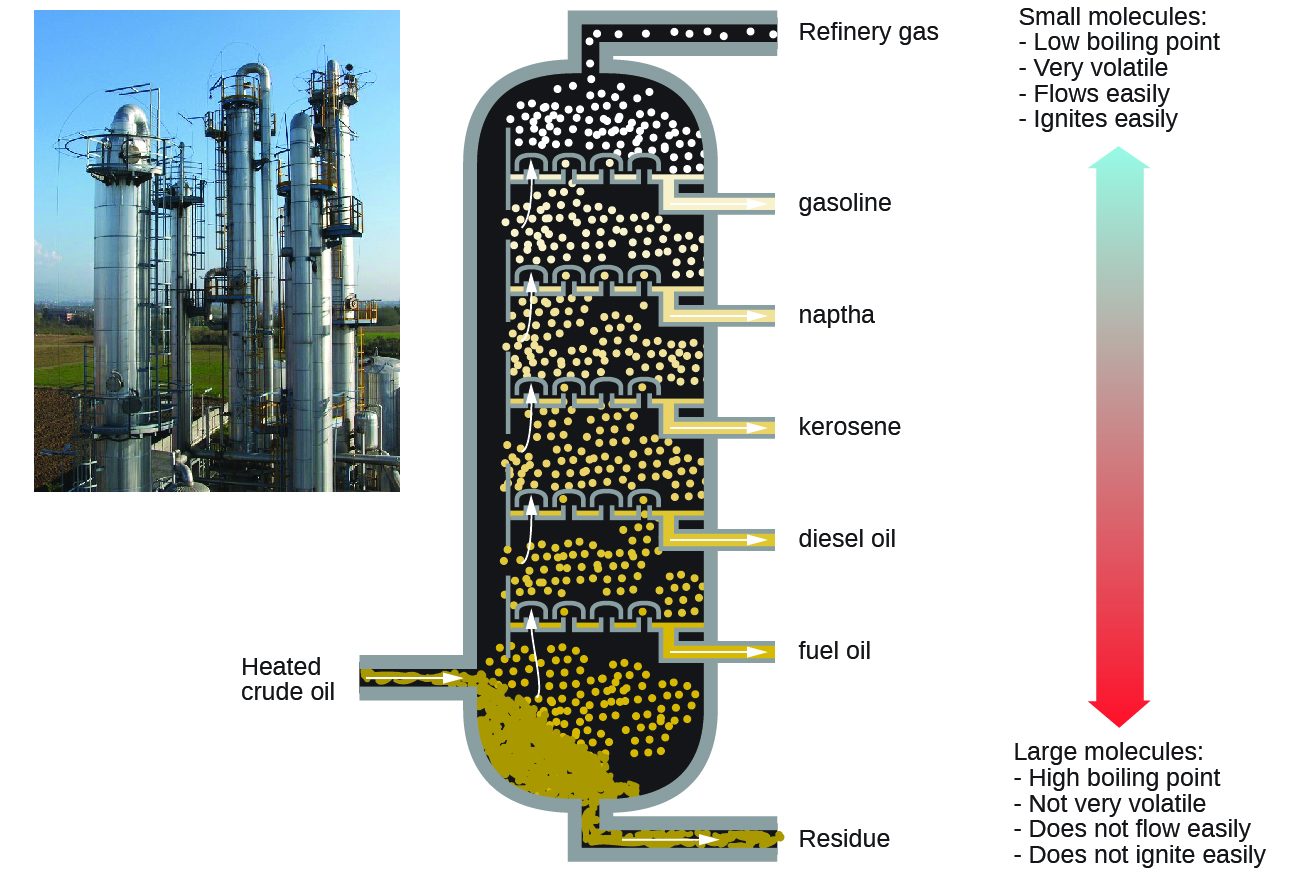
Alkenes, sometimes called olefins, have carbon-carbon double bonds, and alkynes have carbon-carbon triple bonds. Ethene, the simplest alkene example, is a gas that serves as a cellular signal in fruits to stimulate ripening. If you want bananas to ripen quickly, put them in a paper bag along with an apple – the apple emits ethene gas, setting off the ripening process in the bananas. Alkynes burn readily. Ethyne, commonly called acetylene, is used as a fuel in welding blow torches.
Alkanes, alkenes, and alkynes are all classified as hydrocarbons, because they are composed solely of carbon and hydrogen atoms. Alkanes are said to be saturated hydrocarbons, because the carbons are bonded to the maximum possible number of hydrogens – in other words, they are saturated with hydrogen atoms. The double and triple-bonded carbons in alkenes and alkynes have fewer hydrogen atoms bonded to them – they are thus referred to as unsaturated hydrocarbons.
An arene, or an aromatic hydrocarbon is a hydrocarbon with alternative double and single bonds between carbon atoms forming ring. This functional group is exemplified by benzene, which used to be a commonly used solvent on the organic lab, but which was shown to be carcinogenic, and naphthalene, a compound with a distinctive ‘mothball’ smell. Arenes are planar ring structures, and are widespread in nature.
When the carbon of an alkane is bonded to one or more halogens, the group is referred to as a alkyl halide or a haloalkane. Chloroform is a useful solvent in the laboratory, and was one of the earlier anesthetic drugs used in surgery. Chlorodifluoromethane was used as a refrigerant and in aerosol sprays until the late twentieth century, but its use was discontinued after it was found to have harmful effects on the ozone layer. Bromoethane is a simple alkyl halide often used in organic synthesis. Alkyl halides groups are quite rare in biomolecules.
In the alcohol functional group, a carbon is single-bonded to an OH group. The OH group, by itself, is referred to as a hydroxyl. Ethanol, CH3CH2OH, also called ethyl alcohol, is a particularly important alcohol for human use. Ethanol is the alcohol produced by some species of yeast that is found in wine, beer, and distilled drinks. It has long been prepared by humans harnessing the metabolic efforts of yeasts in fermenting various sugars:
Except for methanol, all alcohols can be classified as primary, secondary, or tertiary. In a primary alcohol, the carbon bonded to the OH group is also bonded to only one other carbon. In a secondary alcohol and tertiary alcohol, the carbon is bonded to two or three other carbons, respectively. When the hydroxyl group is directly attached to an aromatic ring, the resulting group is called a phenol. The sulfur analog of an alcohol is called a thiol, from the Greek thio, for sulfur.
Note that the definition of a phenol states that the hydroxyl oxygen must be directly attached to one of the carbons of the aromatic ring. The compound below, therefore, is not a phenol – it is a primary alcohol. The distinction is important, because there is a significant difference in the reactivity of alcohols and phenols.
In an ether functional group, a central oxygen is bonded to two carbons. Below is the structure of diethyl ether, a common laboratory solvent and also one of the first compounds to be used as an anesthetic during operations. Diethyl ether, the most widely used compound of this class, is a colorless, volatile liquid that is highly flammable. It was first used in 1846 as an anesthetic, but better anesthetics have now largely taken its place. Diethyl ether and other ethers are presently used primarily as solvents for gums, fats, waxes, and resins. The sulfur analog of an ether is called a thioether or sulfide.
Amines are characterized by nitrogen atoms with single bonds to hydrogen and carbon. Just as there are primary, secondary, and tertiary alcohols, there are primary, secondary, and tertiary amines. Ammonia is a special case with no carbon atoms. One of the most important properties of amines is that they are basic, and are readily protonated to form ammonium cations when reacted with an acid. In the case where a nitrogen has four bonds to carbon, which is somewhat unusual in biomolecules, it is called a quaternary ammonium ion.
Note: Do not be confused by how the terms ‘primary’, ‘secondary’, and ‘tertiary’ are applied to alcohols and amines – the definitions are different. In alcohols, what matters is how many other carbons the alcohol carbon is bonded to, while in amines, what matters is how many carbons the nitrogen is bonded to.
In some amines, the nitrogen atom replaces a carbon atom in an aromatic hydrocarbon. Pyridine is one such heterocyclic amine. A heterocyclic compound contains atoms of two or more different elements in its ring structure.
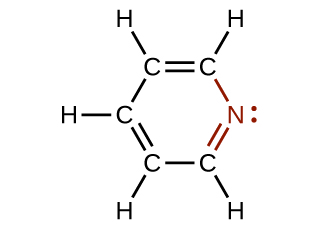
DNA in Forensics and Paternity
The genetic material for all living things is a polymer of four different molecules, which are themselves a combination of three subunits. The genetic information, the code for developing an organism, is contained in the specific sequence of the four molecules, similar to the way the letters of the alphabet can be sequenced to form words that convey information. The information in a DNA sequence is used to form two other types of polymers, one of which are proteins. The proteins interact to form a specific type of organism with individual characteristics.
A genetic molecule is called DNA, which stands for deoxyribonucleic acid. The four molecules that make up DNA are called nucleotides. Each nucleotide consists of a single- or double-ringed molecule containing nitrogen, carbon, oxygen, and hydrogen called a nitrogenous base. Each base is bonded to a five-carbon sugar called deoxyribose. The sugar is in turn bonded to a phosphate group [latex](-\text{PO}_4^{\;\;3-})[/latex] When new DNA is made, a polymerization reaction occurs that binds the phosphate group of one nucleotide to the sugar group of a second nucleotide. The nitrogenous bases of each nucleotide stick out from this sugar-phosphate backbone. DNA is actually formed from two such polymers coiled around each other and held together by hydrogen bonds between the nitrogenous bases. Thus, the two backbones are on the outside of the coiled pair of strands, and the bases are on the inside. The shape of the two strands wound around each other is called a double helix (see Figure 3).
It probably makes sense that the sequence of nucleotides in the DNA of a cat differs from those of a dog. But it is also true that the sequences of the DNA in the cells of two individual pugs differ. Likewise, the sequences of DNA in you and a sibling differ (unless your sibling is an identical twin), as do those between you and an unrelated individual. However, the DNA sequences of two related individuals are more similar than the sequences of two unrelated individuals, and these similarities in sequence can be observed in various ways. This is the principle behind DNA fingerprinting, which is a method used to determine whether two DNA samples came from related (or the same) individuals or unrelated individuals.

Using similarities in sequences, technicians can determine whether a man is the father of a child (the identity of the mother is rarely in doubt, except in the case of an adopted child and a potential birth mother). Likewise, forensic geneticists can determine whether a crime scene sample of human tissue, such as blood or skin cells, contains DNA that matches exactly the DNA of a suspect.
The basicity of an amine’s nitrogen atom plays an important role in much of the compound’s chemistry. Amine functional groups are found in a wide variety of compounds, including natural and synthetic dyes, polymers, vitamins, and medications such as penicillin and codeine. They are also found in many molecules essential to life, such as amino acids, hormones, neurotransmitters, and DNA.
Addictive Alkaloids
Since ancient times, plants have been used for medicinal purposes. One class of substances, called alkaloids, found in many of these plants has been isolated and found to contain cyclic molecules with an amine functional group. These amines are bases. They can react with H3O+ in a dilute acid to form an ammonium salt, and this property is used to extract them from the plant:
The name alkaloid means “like an alkali.” Thus, an alkaloid reacts with acid. The free compound can be recovered after extraction by reaction with a base:
The structures of many naturally occurring alkaloids have profound physiological and psychotropic effects in humans. Examples of these drugs include nicotine, morphine, codeine, and heroin. The plant produces these substances, collectively called secondary plant compounds, as chemical defenses against the numerous pests that attempt to feed on the plant:
In these diagrams, as is common in representing structures of large organic compounds, carbon atoms in the rings and the hydrogen atoms bonded to them have been omitted for clarity. The solid wedges indicate bonds that extend out of the page. The dashed wedges indicate bonds that extend into the page. Notice that small changes to a part of the molecule change the properties of morphine, codeine, and heroin. Morphine, a strong narcotic used to relieve pain, contains two hydroxyl functional groups, located at the bottom of the molecule in this structural formula. Changing one of these hydroxyl groups to a methyl ether group forms codeine, a less potent drug used as a local anesthetic. If both hydroxyl groups are converted to esters of acetic acid, the powerfully addictive drug heroin results (Figure 4).
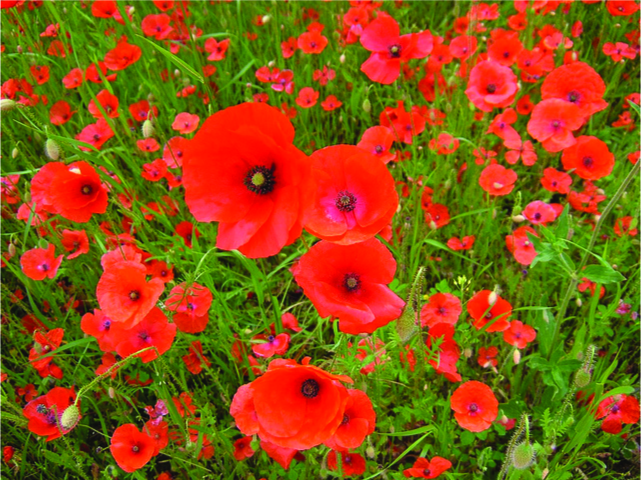
There are a number of functional groups that contain a carbon-oxygen double bond, which is commonly referred to as a carbonyl. Ketones and aldehydes are two closely related carbonyl-based functional groups that react in very similar ways. In a ketone, the carbon atom of a carbonyl is bonded to two other carbons. In an aldehyde, the carbonyl carbon is bonded on one side to a hydrogen, and on the other side to a carbon. The exception to this definition is formaldehyde, in which the carbonyl carbon has bonds to two hydrogens.
Formaldehyde, an aldehyde with the formula HCHO, is a colorless gas with a pungent and irritating odor. It is sold in an aqueous solution called formalin, which contains about 37% formaldehyde by weight. Formaldehyde causes coagulation of proteins, so it kills bacteria (and any other living organism) and stops many of the biological processes that cause tissue to decay. Thus, formaldehyde is used for preserving tissue specimens and embalming bodies. It is also used to sterilize soil or other materials. Formaldehyde is used in the manufacture of Bakelite, a hard plastic having high chemical and electrical resistance.
Dimethyl ketone, CH3COCH3, commonly called acetone, is the simplest ketone. It is made commercially by fermenting corn or molasses, or by oxidation of 2-propanol. Acetone is a colorless liquid. Among its many uses are as a solvent for lacquer (including fingernail polish), cellulose acetate, cellulose nitrate, acetylene, plastics, and varnishes; as a paint and varnish remover; and as a solvent in the manufacture of pharmaceuticals and chemicals.
When a carbonyl carbon is bonded on one side to a carbon (or hydrogen) and on the other side to an oxygen, nitrogen, or sulfur, the functional group is considered to be one of the ‘carboxylic acid derivatives’, a designation that describes a set of related functional groups. The eponymous member of this family is the carboxylic acid functional group, in which the carbonyl is bonded to a hydroxyl group. The conjugate base of a carboxylic acid is a carboxylate. Carboxylic esters, usually just called ‘esters’ and amides are some of the other carboxylic acid derivatives.
The simplest carboxylic acid is formic acid, HCO2H, known since 1670. Its name comes from the Latin word formicus, which means “ant”; it was first isolated by the distillation of red ants. It is partially responsible for the pain and irritation of ant and wasp stings, and is responsible for a characteristic odor of ants that can be sometimes detected in their nests.
Acetic acid, CH3CO2H, constitutes 3–6% vinegar. Cider vinegar is produced by allowing apple juice to ferment without oxygen present. Yeast cells present in the juice carry out the fermentation reactions. The fermentation reactions change the sugar present in the juice to ethanol, then to acetic acid. Pure acetic acid has a penetrating odor and produces painful burns. It is an excellent solvent for many organic and some inorganic compounds, and it is essential in the production of cellulose acetate, a component of many synthetic fibers such as rayon.
The distinctive and attractive odors and flavors of many flowers, perfumes, and ripe fruits are due to the presence of one or more esters (Figure 5). Among the most important of the natural esters are fats (such as lard, tallow, and butter) and oils (such as linseed, cottonseed, and olive oils), which are esters of the trihydroxyl alcohol glycerine, [latex]\text{C}_3\text{H}_5\text{(OH)}_3[/latex], with large carboxylic acids, such as palmitic acid, [latex]\text{CH}_3\text{(CH}_2)_{14}\text{CO}_2\text{H}[/latex], stearic acid, [latex]\text{CH}_3\text{(CH}_2)_{16}\text{CO}_2\text{H}[/latex], and oleic acid, [latex]\text{CH}_3\text{(CH}_2)_7\text{CH} = \text{CH(CH}_2)_7\text{CO}_2\text{H}[/latex]. Oleic acid is an unsaturated acid; it contains a [latex]\text{C} = \text{C}[/latex] double bond. Palmitic and stearic acids are saturated acids that contain no double or triple bonds.
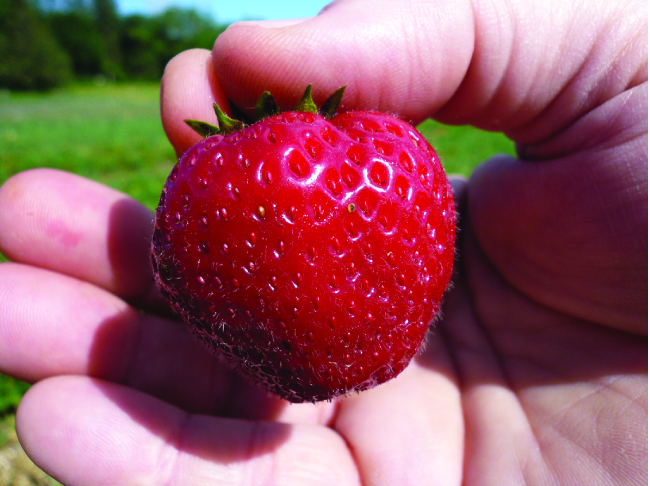
Esters and amides are very common in biological molecules and/or metabolic pathways. Amides can be produced when carboxylic acids react with amines or ammonia in a process called amidation. A water molecule is eliminated from the reaction, and the amide is formed from the remaining pieces of the carboxylic acid and the amine:
The reaction between amines and carboxylic acids to form amides is biologically important. It is through this reaction that amino acids (molecules containing both amine and carboxylic acid substituents) link together in a polymer to form proteins.
Proteins and Enzymes
Proteins are large biological molecules made up of long chains of smaller molecules called amino acids. Organisms rely on proteins for a variety of functions—proteins transport molecules across cell membranes, replicate DNA, and catalyze metabolic reactions, to name only a few of their functions. The properties of proteins are functions of the combination of amino acids that compose them and can vary greatly. Interactions between amino acid sequences in the chains of proteins result in the folding of the chain into specific, three-dimensional structures that determine the protein’s activity.
Amino acids are organic molecules that contain an amine functional group (–NH2), a carboxylic acid functional group (–COOH), and a side chain (that is specific to each individual amino acid). Most living things build proteins from the same 20 different amino acids. Amino acids connect by the formation of a peptide bond, which is a covalent bond formed between two amino acids when the carboxylic acid group of one amino acid reacts with the amine group of the other amino acid. The formation of the bond results in the production of a molecule of water (in general, reactions that result in the production of water when two other molecules combine are referred to as condensation reactions). The resulting bond—between the carbonyl group carbon atom and the amine nitrogen atom is called a peptide link or peptide bond. Since each of the original amino acids has an unreacted group (one has an unreacted amine and the other an unreacted carboxylic acid), more peptide bonds can form to other amino acids, extending the structure. (Figure 6) A chain of connected amino acids is called a polypeptide. Proteins contain at least one long polypeptide chain.
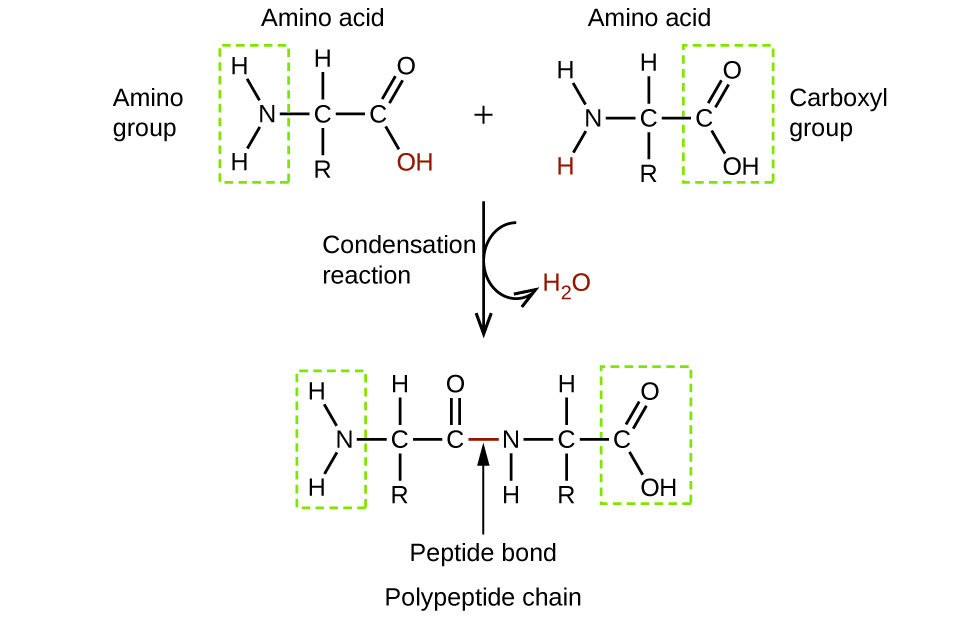
Enzymes are large biological molecules, mostly composed of proteins, which are responsible for the thousands of metabolic processes that occur in living organisms. Enzymes are highly specific catalysts; they speed up the rates of certain reactions. Enzymes function by lowering the activation energy of the reaction they are catalyzing, which can dramatically increase the rate of the reaction. Most reactions catalyzed by enzymes have rates that are millions of times faster than the noncatalyzed version. Like all catalysts, enzymes are not consumed during the reactions that they catalyze. Enzymes do differ from other catalysts in how specific they are for their substrates (the molecules that an enzyme will convert into a different product). Each enzyme is only capable of speeding up one or a few very specific reactions or types of reactions. Since the function of enzymes is so specific, the lack or malfunctioning of an enzyme can lead to serious health consequences. One disease that is the result of an enzyme malfunction is phenylketonuria. In this disease, the enzyme that catalyzes the first step in the degradation of the amino acid phenylalanine is not functional (Figure 7). Untreated, this can lead to an accumulation of phenylalanine, which can lead to intellectual disabilities.
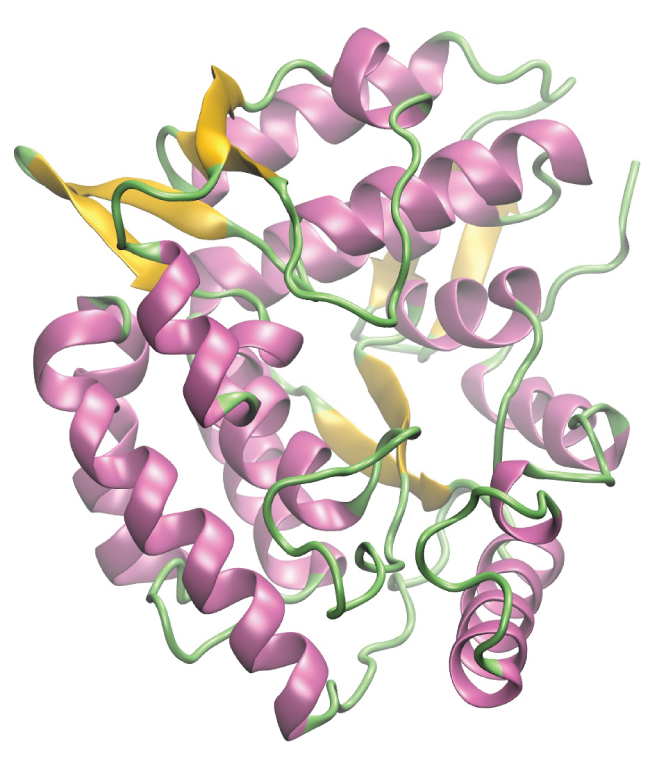
Finally, a nitrile group is characterized by a carbon triple-bonded to a nitrogen.
A single compound often contains several functional groups, particularly in biological organic chemistry. The six-carbon sugar molecules glucose and fructose, for example, contain aldehyde and ketone groups, respectively, and both contain five alcohol groups, a compound with several alcohol groups is often referred to as a ‘polyol’.
The hormone testosterone, the amino acid phenylalanine, and the glycolysis metabolite dihydroxyacetone phosphate all contain multiple functional groups, as labeled below.
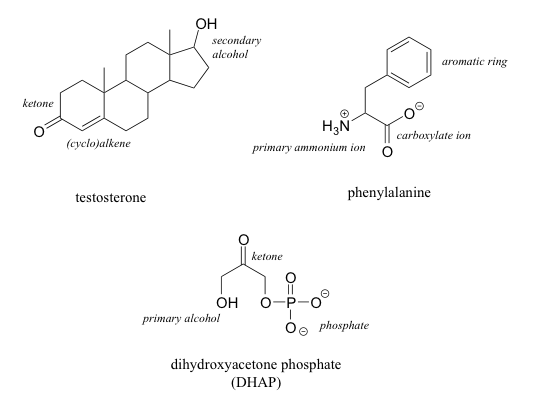
While not in any way a complete list, this section has covered many of the important functional groups that are encountered in organic chemistry. Table 1 provides a summary of all of the functional groups listed in this section, plus a few more that may be encountered in a second year organic chemistry course.
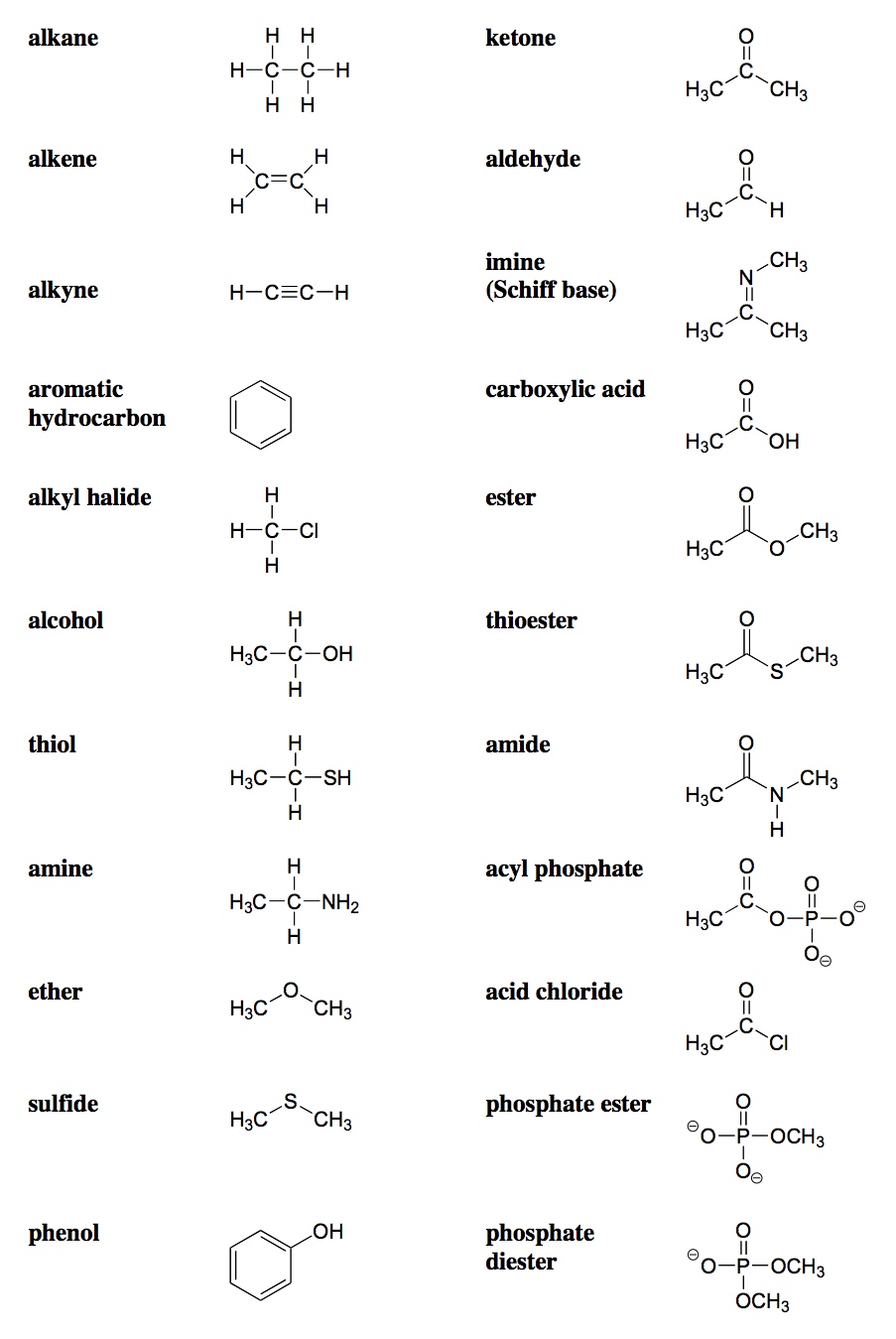
Key Concepts and Summary
Functional groups are structural units within organic compounds that are defined by specific bonding arrangements between specific atoms. Organic chemist learn to correlate functional groups to the chemistry that they do. For example, any molecule that contains a carboxylic acid, will be able to do an acid-base reaction when mixed with a base. This allows the organic chemist to have a sense of the various chemistry a compound can or cannot do based on the functional groups that are present in the structure.
Activity
Make yourself a stack of small sized Qcards. On one side have the name of the functional group (e.g. alcohol) and on the other side have its structure (see Table 1). Make a complete set of all the functional groups you should know (see Table 1). You can even include some compounds like those below in exercise 1 – on one side of the card have the compound, on the other the names of the functional groups. Then use these Qcards to quiz yourself. This will help you recognize the functional groups in larger compounds.
Exercises
1. Answer the following questions for each of these compounds.
a) Name the circled functional groups: A = ?, B =?, C = ?
b) What is the chemical formula of the compound?
c) How many lone pairs are there in the compound?
Phenylpropanolamine is a psychoactive drug which is used as a stimulant and decongestant in prescription and over-the-counter cough and cold medicines.
Triiodothyronine is a thyroid hormone which affects many physiological processes in the body, such as growth, metabolism and heart rate.
Aldosterone is a hormone which is involved in the function of the kidneys.
Ephedrine is a drug commonly used as a stimulant, decongestant and also as a concentration aid.
Clomifene is mainly used for ovarian stimulation in female infertility which is due to anomulation.
2. Among the five compounds listed in question 1, which would do an acid-base reaction when mixed with sodium hydroxide (which is a base).
Answers
Phenylpropanolamine:
a) A = Primary Amine, B = Secondary Alcohol, C = Arene
b) C9H13NO
c) 3 lone pairs – one on the nitrogen and two on the oxygen
Triiodothyronine:
a) A = Carboxylic Acid, B = Ether, C = Primary Amine
b) C15H12I3NO4
c) 18 lone pairs – one on each nitrogen, two on each oxygen, and three on each iodine
Aldosterone:
a) A = Secondary Alcohol, B = Aldehyde, C = Ketone
b) C21H28O5
c) 10 lone pairs – two on each oxygen
Ephedrine:
a) A = Secondary Amine, B = Secondary Alcohol, C = Arene
b) C10H15NO
c) 3 lone pairs – one on the nitrogen and two on the oxygen
Clomifene:
a) A = Arene, B = Ether, C = Tertiary Amine
b) C26H28ClNO
c) 6 lone pairs – one on the nitrogen, two on the oxygen, and three on the chlorine
2. Any molecule that contains a carboxylic acid, will be able to do an acid-base reaction when mixed with a base. Therefore among the five compounds in question 1, the only one that has a carboxylic acid is Triiodothyronine.