Neuromuscular control of motion
J. Gordon Betts; James A. Wise; Kelly A. Young; Eddie Johnson; Brandon Poe; Dean H. Kruse; Oksana Korol; Jody E. Johnson; Mark Womble; and Peter DeSaix
Learning Objectives
By the end of this section, you will be able to:
- Describe the basic structure of a neuron
- Describe the basic components and functions of the action potential
- Identify the different types of neurons on the basis of polarity
- List the glial cells of the CNS and describe their function
- List the glial cells of the PNS and describe their function
Nervous tissue is composed of two types of cells, neurons and glial cells. Neurons are responsible for the computation and communication that the nervous system provides. Changes in the electrical activity of neurons can create an action potential, which is the main form of cell-cell communication in the nervous system. The Glial cells, or glia, are known to play a supporting role for nervous tissue. Ongoing research pursues an expanded role that glial cells might play in signaling, but neurons are still considered the basis of this function. Neurons are important, but without glial support they would not be able to perform their function.
Neurons
Neurons are the cells considered to be the basis of nervous tissue. They are responsible for the electrical signals that communicate information about sensations, and that produce movements in response to those stimuli, along with inducing thought processes within the brain. An important part of the function of neurons is in their structure, or shape. The three-dimensional shape of these cells makes the immense numbers of connections within the nervous system possible.
Parts of a Neuron
The main part of a neuron is the cell body, which is also known as the soma (soma = “body”). The cell body contains the nucleus and most of the major organelles. But what makes neurons special is that they have many extensions of their cell membranes, which are referred to as processes. Neurons are usually described as having one, and only one, axon—a type of process that emerges from the cell body and projects to other neurons, known as target cells. That single axon can branch repeatedly to communicate with many target cells. It is the axon that propagates the action potential which is communicated to one or more cells. The other processes of the neuron are dendrites, which receive information from other neurons at specialized areas of contact between two neurons called synapses. The dendrites are usually highly branched processes, providing locations for many other neurons to communicate with the cell body. Information flows through a neuron from the dendrites, across the cell body, and down the axon. This gives the neuron a polarity—meaning that information flows in this one direction. Figure 12.7 shows the relationship of these parts to one another.
Where the axon first emerges from the cell body, there is a special region referred to as the axon hillock. This is a tapering of the cell body toward the axon fibre. Many axons are wrapped by an insulating substance called myelin, which is actually made from glial cells. Myelin acts as insulation much like the plastic or rubber that is used to insulate electrical wires. A key difference between myelin and the insulation on a wire is that there are gaps in the myelin covering of an axon. Each gap is called a node of Ranvier and is important to the way that electrical signals travel down the axon. The length of the axon between each gap, which is wrapped in myelin, is referred to as an axon segment. At the end of the axon is the axon terminal, where there are usually several branches extending toward the target cell, each of which ends in an enlargement called a synaptic end bulb. These bulbs are what make the connection with the target cell at the synapse.
Action Potentials: The Basics
Neurons communicate with one another by propagating electrical impulses from the axon hillock down their axon segments and towards the axon terminal and synaptic end bulbs. These electrical impulses happen because positively charged ions such as sodium (Na+) and potassium (K+) flow into or out of the cell very rapidly through small channels in the neuron cell membrane. Once the electrical impulse reaches the synaptic end bulbs, this serves as a trigger for the neuron to release small chemical messengers called neurotransmitters into the synapse (the space between two adjacent neurons). You may have heard of neurotransmitters like serotonin or dopamine before— these are the signals that neurons use to communicate with each other. Neurotransmitters will move through the synapse and bind to the dendrites of the next neuron and trigger a response. The type of response in the next neuron can vary, it may include promoting or preventing the firing of another action potential down it’s own axon. The two neurons that are communicating with each other are sometimes called the “post-synaptic” and “pre-synaptic” cell to describe their positions relative to the synapse.
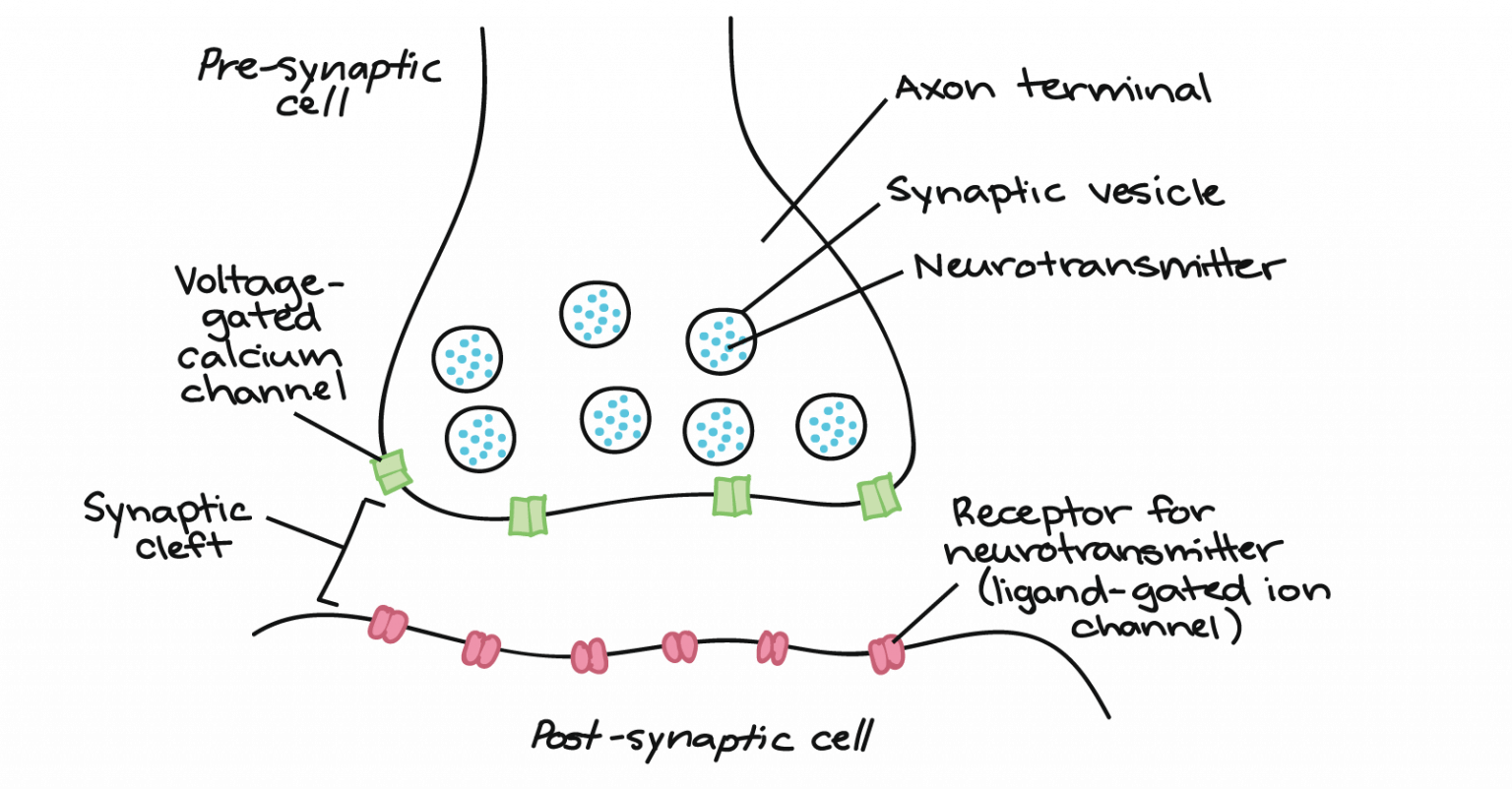
Glial cells, or neuroglia or simply glia, are the other type of cell found in nervous tissue. They function largely in supporting neurons to carry out their functions, although new research suggests they may have more complex roles. They are considered to be supporting cells, and many functions are directed at helping neurons complete their function for communication.
There are six types of glial cells. Four of them are found in the CNS and two are found in the PNS. Table 12.2 outlines some common characteristics and functions.
CNS glia | PNS glia | Basic function |
---|---|---|
Astrocyte | Satellite cell | Support |
Oligodendrocyte | Schwann cell | Insulation, myelination |
Microglia | – | Immune surveillance and phagocytosis |
Ependymal cell | – | Creating CSF |
Glial Cells of the CNS
One cell providing support to neurons of the CNS is the astrocyte, so named because it appears to be star-shaped under the microscope (astro- = “star”). Astrocytes have many processes extending from their main cell body (not axons or dendrites like neurons, just cell extensions). Those processes extend to interact with neurons, blood vessels, or the connective tissue covering the CNS that is called the pia mater (Figure 12.11). Generally, they are supporting cells for the neurons in the central nervous system. Some ways in which they support neurons are by maintaining the concentration of chemicals in the extracellular space, removing excess signaling molecules, reacting to tissue damage, and contributing to the blood-brain barrier (BBB). The BBB is a physiological barrier that keeps many substances that circulate in the rest of the body from getting into the central nervous system, restricting what can cross from circulating blood into the CNS. Nutrient molecules, such as glucose or amino acids, can pass through the BBB, but other molecules cannot. This actually causes problems with drug delivery to the CNS. Pharmaceutical companies are challenged to design drugs that can cross the BBB and have an effect on the nervous system.
Another cell type found in CNS tissue is the oligodendrocyte, sometimes called just “oligo,” which is the glial cell type that insulates axons in the CNS. The name means “cell of a few branches” (oligo- = “few”; dendro- = “branches”; -cyte = “cell”). There are a few processes that extend from the cell body. Each one reaches out and surrounds an axon to insulate it in myelin. The function of myelin will be discussed below.
Microglia are, as the name implies, smaller than most of the other glial cells. Ongoing research into these cells, although not entirely conclusive, suggests that they may originate as white blood cells, called macrophages, that become part of the CNS during early development. While their origin is not conclusively determined, their function is related to what macrophages do in the rest of the body. When macrophages encounter diseased or damaged cells in the rest of the body, they ingest and digest those cells or the pathogens that cause disease. Microglia are the cells in the CNS that can do this in normal, healthy tissue, and they are therefore also referred to as CNS-resident macrophages.
The ependymal cell is a glial cell that filters blood to make cerebrospinal fluid (CSF), the fluid that circulates through the CNS. Because of the privileged blood supply inherent in the BBB, the extracellular space in nervous tissue does not easily exchange components with the blood. Ependymal cells line each ventricle, one of four central cavities that are remnants of the hollow center of the neural tube formed during the embryonic development of the brain. The choroid plexus is a specialized structure in the ventricles where ependymal cells come in contact with blood vessels and filter and absorb components of the blood to produce cerebrospinal fluid. Because of this, ependymal cells can be considered a component of the BBB, or a place where the BBB breaks down. These glial cells appear similar to epithelial cells, making a single layer of cells with little intracellular space and tight connections between adjacent cells. They also have cilia on their apical surface to help move the CSF through the ventricular space. The relationship of these glial cells to the structure of the CNS is seen in Figure 12.11.
Glial Cells of the PNS
One of the two types of glial cells found in the PNS is the satellite cell. Satellite cells are found in sensory and autonomic ganglia, where they surround the cell bodies of neurons. They provide support, performing similar functions in the periphery as astrocytes do in the CNS—except, of course, for establishing the BBB.
The second type of glial cell is the Schwann cell, which insulate axons with myelin in the periphery. Schwann cells are different than oligodendrocytes, in that a Schwann cell wraps around a portion of only one axon segment and no others. Oligodendrocytes have processes that reach out to multiple axon segments, whereas the entire Schwann cell surrounds just one axon segment. The nucleus and cytoplasm of the Schwann cell are on the edge of the myelin sheath. The relationship of these two types of glial cells to ganglia and nerves in the PNS is seen in Figure 12.10.
Myelin
The insulation for axons in the nervous system is provided by oligodendrocytes in the CNS, and Schwann cells in the PNS. Myelin is a lipid-rich material that surrounds the axon and by doing so creates a sheath that facilitates the transmission of electrical signals along the axon.
The glial cell is wrapped around the axon several times with little to no cytoplasm between the glial cell layers. During development, the glial cell is loosely or incompletely wrapped around the axon (Figure 12.11a). The edges of this loose enclosure extend toward each other, and one end tucks under the other. The inner edge wraps around the axon, creating several layers, and the other edge closes around the outside so that the axon is completely enclosed.
Myelin sheaths can extend for one or two millimeters, depending on the diameter of the axon. Axon diameters can be as small as 1 to 20 micrometers. Because a micrometer is 1/1000 of a millimeter, this means that the length of a myelin sheath can be 100–1000 times the diameter of the axon. Figure 12.11 show the myelin sheath surrounding an axon segment, but are not to scale. If the myelin sheath were drawn to scale, the neuron would have to be immense—possibly covering an entire wall of the room in which you are sitting.
Disorders of the Myelin Sheath
Several diseases can result from the demyelination of axons. The causes of these diseases are not the same; some have genetic causes, some are caused by pathogens, and others are the result of autoimmune disorders. Though the causes are varied, the results are largely similar. The myelin insulation of axons is compromised, making electrical signaling slower.
Multiple sclerosis (MS) is a demyelinating disease caused by our own immune systems, making it an autoimmune disorder. The antibodies produced by b-lymphocytes (a type of white blood cell) mark myelin as a substance that is foreign to the body. This causes inflammation and the destruction of the myelin in the central nervous system. As the insulation around the axons is destroyed by the disease, scarring becomes obvious. This is where the name of the disease comes from; sclerosis means hardening of tissue, which is what a scar is. Multiple scars are found in the white matter of the brain and spinal cord. The symptoms of MS include both somatic and autonomic deficits. Control of the musculature is compromised, as is control of organs such as the bladder. We will explore MS in greater detail in a later chapter.
Guillain-Barré (pronounced gee-YAN bah-RAY) syndrome is an example of a demyelinating disease of the peripheral nervous system. It is also the result of an autoimmune reaction, but the inflammation is in peripheral nerves. Sensory symptoms or motor deficits are common, and autonomic failures can lead to changes in the heart rhythm or a drop in blood pressure, especially when standing, which causes dizziness.
Adaption
This chapter was adapted by Valerie Swanston and Carter Allen from the following text:
Nervous Tissue – Anatomy in Anatomy and Physiology by OSCRiceUniversity is licensed under a Creative Commons Attribution 4.0 International License