Acute Kidney Injury
Physiology of Urine Formation
J. Gordon Betts; Kelly A. Young; James A. Wise; Eddie Johnson; Brandon Poe; Dean H. Kruse; Oksana Korol; Jody E. Johnson; Mark Womble; and Peter DeSaix
Learning Objectives
By the end of this section, you will be able to:
- Describe the hydrostatic and osmotic forces that influence renal filtration
- Define glomerular filtration rate (GFR)
- List specific transport mechanisms occurring in different parts of the nephron, including active transport, osmosis, facilitated diffusion, and passive electrochemical gradients
- Explain why the differential permeability or impermeability of specific sections of the nephron tubules is necessary for urine formation
- Describe how and where water, organic compounds, and ions are reabsorbed in the nephron
- List the locations in the nephron where tubular secretion occurs
Having reviewed the anatomy and microanatomy of the urinary system, now is the time to focus on the physiology. You will discover that different parts of the nephron use filtration, reabsorption, and secretion to produce urine. You will learn how each of these processes works and where they occur along the nephron and collecting ducts. The physiologic goal is to modify the composition of the glomerular filtrate and produce the waste product urine.
Glomerular Filtration Rate (GFR)
The volume of filtrate formed by both kidneys per minute is termed the glomerular filtration rate (GFR). The volume of filtrate produced by the kidneys per day is about 180 L in males and 150 L in females. Ninety-nine percent of this filtrate is returned to the circulation by reabsorption so that only about 1–2 liters of urine are produced per day.
GFR is influenced by the hydrostatic pressure and osmotic pressure on either side of the capillary membrane of the glomerulus. Filtration occurs as pressure forces fluid and solutes through a semipermeable barrier with the solute movement constrained by particle size. Hydrostatic pressure is the pressure produced by a fluid against a surface. If you have a fluid on both sides of a barrier, both fluids exert a pressure in opposing directions. Net fluid movement will be in the direction of the lower pressure.
Osmosis is the movement of solvent (water) across a semipermeable membrane from an area of low solute concentration to an area of high solute concentration in an attempt to balance the concentration on both sides. This creates a pressure, osmotic pressure, which will exist until the solute concentration is the same on both sides of a semipermeable membrane. As long as the concentration differs, water will move.
Glomerular filtration occurs when glomerular hydrostatic pressure exceeds the hydrostatic pressure of Bowman’s space. Osmotic pressure tends to oppose filtration because solute concentration is typically higher in the glomerular capillary. This is because there is a size limitation on the substances that can pass into the filtrate, leading to higher solute concentrations in the blood compared to the filtrate. The sum of all of the influences, both osmotic and hydrostatic, results in a net filtration pressure (NFP) of about 10 mm Hg (Figure 9.9).
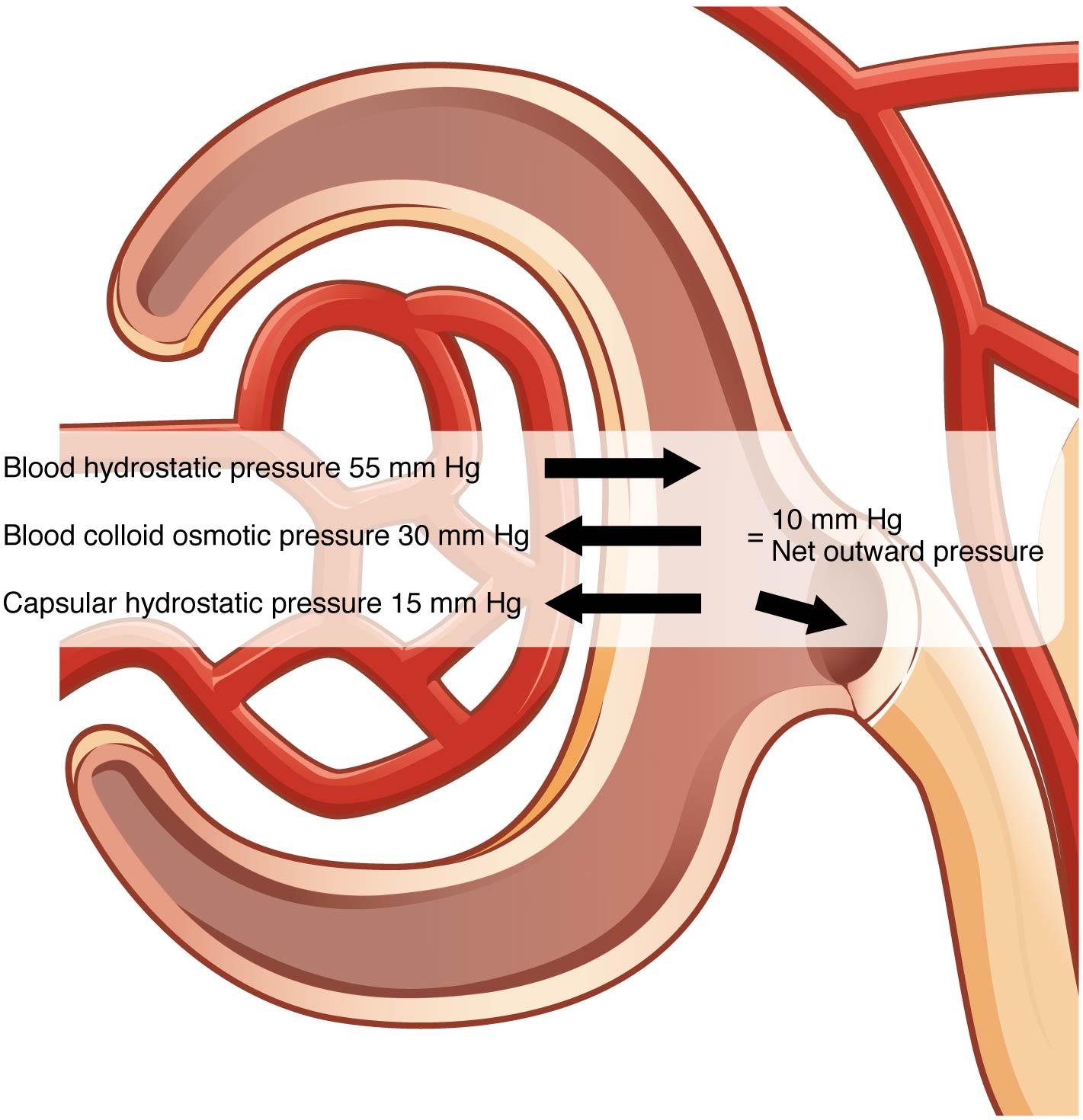
A proper concentration of solutes in the blood is important in maintaining osmotic pressure both in the glomerulus and systemically. There are disorders in which too much protein passes through the filtration slits into the kidney filtrate. This excess protein in the filtrate leads to a deficiency of proteins in the blood. Because there is less circulating protein, the osmotic pressure of the blood falls. Less osmotic pressure pulling water into the capillaries tips the balance towards hydrostatic pressure, which tends to push it out of the capillaries. The overall effect is that water is lost from the circulation to interstitial tissues and cells. This “plumps up” the tissues and cells, causing a condition termed systemic edema.
Net Filtration Pressure (NFP)
When combining hydrostatic and osmotic pressures (Figure 9.9), there is a low net pressure across the filtration membrane. Therefore, minor changes in osmolarity of the blood or capillary blood pressure result in major changes in the amount of filtrate formed. The kidney is able to cope with a wide range of blood pressures because the smooth muscle in the afferent arterioles that feed the glomerular capillaries is autoregulatory. When you stretch it, it contracts. Thus, when blood pressure goes up, smooth muscle in the afferent arterioles contracts to limit any increase in blood flow and filtration rate. When blood pressure drops, the same arterioles relax to let more blood through and maintain filtration rate. This autoregulation can compensate for a wide range of blood pressures, but will start being unable to compensate below a mean arterial pressure of around 60 mmHg. Blood pressures below this level will impair renal function and cause systemic disorders that are severe enough to threaten survival. This condition is called shock.
Clinical use of GFR
Determination of GFR is important clinically. For example, many dyes and drugs are excreted by the kidneys. In patients with impaired renal function, drug dose adjustments may be required to avoid accumulation of the drug to a toxic level. The GFR is used in this calculation.
But how do we determine a patient’s GFR? It is calculated by measuring creatinine present in the blood. Creatinine is a protein-derived molecule produced by muscle tissue that is freely filtered at the glomerulus and is not significantly secreted or reabsorbed by the renal tubules, making it a good candidate to assess GFR. If kidney function is impaired, creatinine will rise because it is not being adequately filtered out by the kidneys.
- Medical Lab technologists receive blood samples to measure the amount of creatinine and nitrogenous waste products (e.g. urea). These wastes should be in low amounts in the blood when the kidney is functioning properly to eliminate these wastes every minute of the day.
- Medical imaging and nuclear medicine tests sometimes use dyes or radioisotopes to help visualize their images (e.g. MRI, CT) or function tests (e.g. myocardial perfusion test). However, these dyes and radioisotopes must be eliminated from the body once the imaging/tests have been completed. Thus, GFR must be within normal range to allow for such dyes in these procedures, knowing that they can be safely eliminated
Tubular reabsorption
Seeing as up to 180 liters of fluid pass through the nephrons of the kidney per day, it is important that most of that water be reabsorbed, along with the electrolytes and nutrients that also pass into the filtrate. The term “reabsorption” is used because these substances originated in the blood and are returning to the blood, in contrast to the digestive system in which nutrients are absorbed from food. The main functions of the kidney tubules are reabsorption of nutrients, maintenance of homeostasis, and elimination of waste, all of which they accomplish by selective reabsorption of nutrients, electrolytes, and water. This is a brief overview of the mechanisms of reabsorption and the functions of the different parts of the nephron, but if you are interested in more detail please refer to Tubular Reabsorption.
The kidney has some additional functions that won’t be explored in this chapter. These include regulation of electrolytes and hormone activation and/or release (e.g. vitamin D activation, release of the hormone erythropoietin for RBC synthesis). For more detail on these topics, refer to Endocrine regulation of kidneys and Regulation of fluid volume and electrolytes.
Mechanisms of Reabsorption
You may be wondering how this process of reabsorption occurs. The tubules are lined by a single layer of cells and the luminal side (the side on the inside of tubule, where the filtrate is) contains transport proteins that help to move nutrients via the mechanisms outlined below.
- Active transport: Substances travel against their concentration gradient via a protein “pump” that consumes ATP
- Example: In the proximal convoluted tubule (PCT), a protein actively transports sodium.
- Facilitated diffusion: Substances follow their concentration gradient using a transport protein to cross the cell membrane. This process does not require energy.
- Example: Glucose transport in the PCT.
- Simple diffusion: Substances follow their concentration gradient and diffuse across a membrane. In order to do so, their chemical properties need to allow them to cross the cell membrane, therefore they cannot be charged or too large.
- Example: In parts of the Loop of Henle, urea diffuses across the cell membrane.
- Osmosis: movement of water across the membrane to equalize a concentration gradient of a substance that cannot cross the membrane.
- Example: Water follows actively pumped ions, like Na+.
The Role of Secretion
In addition to reabsorbing important substances, the nephron also secretes certain substances. These are often waste compounds that the body is removing via excretion in the urine. Examples of such waste components are urea and certain drugs. The nephron also uses secretion to balance the body’s pH, usually by secreting H+ and NH4+ to make the urine more acidic and the body more alkaline.
Functions of Renal Tubules
The following section will outline the function of each part of the nephron. Refer to the figure below for an overview.
Proximal Convoluted Tubule (PCT)
Once the filtrate is filtered into Bowman’s space, the first structure it enters is the PCT. Hence, this is the part of the nephron where the filtrate contains the highest amount of substances that need to be reabsorbed, like water, sodium, glucose, amino acids, small proteins, calcium, potassium, magnesium, and chloride. Hence, there are transport proteins located in the PCT to enable reabsorption of all these substances by the mechanisms outlined previously. This is also where the majority of water reabsorption occurs.
Many parts of the nephron have a role in maintaining pH balance and the PCT is one of them. It does this by reabsorbing bicarbonate (HCO3-), which acts as a buffer to balance blood pH. pH balance in the body is vital, because outside of the narrow normal pH range proteins begin to take on or lose hydrogen ions and lose their shape, and therefore their function. In cases of kidney pathology, one of the signs can be acidemia (blood that is too acidic) because the kidneys are not able to adequately balance the pH.
Loop of Henle (LoH)
The main function of the LoH is to reabsorb water and electrolytes. The loop descends towards or into the medulla, where there is a higher concentration of salt and urea. In the descending limb, water responds to the hyperosmotic environment by leaving the tubule by osmosis. The ascending portion is impermeable to water, so the previously reabsorbed water doesn’t just return to the tubule. Here, electrolytes are actively pumped out to maintain the hyperosmotic environment around the LoH.
Distal Convoluted Tubule (DCT)
After passing through the LoH, the filtrate returns to the cortex and enters the DCT. At this point, 80% of the water in the filtrate has been reabsorbed and the DCT recovers another 10-15%. It also has a role in the reabsorption of sodium, chloride, and calcium.
Collecting Ducts
The collecting ducts are not technically part of the nephron and several nephrons will drain into a single collecting duct. However, they still play an important role in controlling the recovery of water. If the body detects a need to retain water, a hormone called antidiuretic hormone (ADH) will be released, which causes aquaporins (water channels) to be inserted into the membranes of the otherwise water-impermeable collecting duct cells. This allows water to be reabsorbed by facilitated diffusion as the filtrate passes through the hyperosmotic medulla on its way to the renal collecting system.
The collecting ducts also have a role in increasing blood pressure when under the influence of the hormone aldosterone. Aldosterone is formed by a chain of events that begin elsewhere in the kidney. The macula densa is a structure that is located where the LoH returns to the cortex and becomes the DCT. It detects the amount of sodium in the filtrate and if it is low, it begins a cascade of events to increase blood pressure and GFR. This is because in a healthy individual, low sodium in this portion of the nephron is usually due to low renal perfusion. The hormone renin is released, which converts angiotensinogen to angiotensin I, which is then converted to angiotensin II. Angiotensin II acts on the vasculature to cause vasoconstriction and also causes release of aldosterone from the adrenal glands, which sit above the kidneys. Aldosterone increases retention of sodium in the collecting duct. When water passively follows, the result is to increase blood volume and assist in maintaining the blood pressure and perfusion of the kidneys. This complex system is often referred to as the RAAS, short for the renin-angiotensin-aldosterone system.
Critical thinking Exercise
Thinking back on the normal physiology of urine formation (one of the main functions of the kidney), that things are possible to appear in the urine? And what is supposed to be there?
- Now speculate what could have happened for you to see RBCs in the urine? What about very large proteins?
Chapter Review
The entire volume of the blood is filtered through the kidneys about 300 times per day, and 99 percent of the water filtered is recovered. The GFR is influenced by hydrostatic pressure and osmotic pressure. Under normal circumstances, hydrostatic pressure is greater and filtration occurs. The hydrostatic pressure of the glomerulus depends on systemic blood pressure and autoregulatory mechanisms, among other mechanisms. The kidney can function normally under a wide range of blood pressures due to the autoregulatory nature of smooth muscle.
The kidney regulates water recovery and blood pressure by producing the enzyme renin, thus starting the Renin-Angiotensin-Aldosterone (RAAS) system. The result of RAAS activation is vasoconstriction and retention of salt and water. Water recovery is also powerfully and directly influenced by the hormone ADH. Even so, it only influences the last 10 percent of water available for recovery after filtration at the glomerulus, because 90 percent of water is recovered before reaching the collecting ducts.
Mechanisms of solute recovery include active transport, simple diffusion, and facilitated diffusion. Most filtered substances are reabsorbed. Urea, NH3, creatinine, and some drugs are filtered or secreted as wastes. H+ and HCO3– are secreted or reabsorbed as needed to maintain acid–base balance. Movement of water from the glomerulus is primarily due to pressure, whereas that of peritubular capillaries and vasa recta is due to osmolarity and concentration gradients. Almost 100 percent of glucose, amino acids, and vitamins are recovered in the PCT. The recovery of solutes creates an osmotic gradient to promote the recovery of water. The descending loop promotes recovery of water. The ascending loop is impervious to water but actively recovers Na+, reducing filtrate osmolarity. The vasa recta recover the solute and water in the medulla, returning them to the circulation. Nearly 90 percent of water is recovered before the forming urine reaches the DCT, which will recover another 10 percent.
Review Questions
Adaption
This chapter is adapted from the following text:
Tubular reabsorption in Anatomy and Physiology by OSCRiceUniversity is licensed under a Creative Commons Attribution 4.0 International License
rate of renal filtration
pressure of fluid across the glomerulus; calculated by taking the hydrostatic pressure of the capillary and subtracting the colloid osmotic pressure of the blood and the hydrostatic pressure of Bowman’s capsule
increased fluid retention in the interstitial spaces and cells of the body; can be seen as swelling over large areas of the body, particularly the lower extremities